
- •Analysis and Application of Analog Electronic Circuits to Biomedical Instrumentation
- •Dedication
- •Preface
- •Reader Background
- •Rationale
- •Description of the Chapters
- •Features
- •The Author
- •Table of Contents
- •1.1 Introduction
- •1.2 Sources of Endogenous Bioelectric Signals
- •1.3 Nerve Action Potentials
- •1.4 Muscle Action Potentials
- •1.4.1 Introduction
- •1.4.2 The Origin of EMGs
- •1.5 The Electrocardiogram
- •1.5.1 Introduction
- •1.6 Other Biopotentials
- •1.6.1 Introduction
- •1.6.2 EEGs
- •1.6.3 Other Body Surface Potentials
- •1.7 Discussion
- •1.8 Electrical Properties of Bioelectrodes
- •1.9 Exogenous Bioelectric Signals
- •1.10 Chapter Summary
- •2.1 Introduction
- •2.2.1 Introduction
- •2.2.4 Schottky Diodes
- •2.3.1 Introduction
- •2.4.1 Introduction
- •2.5.1 Introduction
- •2.5.5 Broadbanding Strategies
- •2.6 Photons, Photodiodes, Photoconductors, LEDs, and Laser Diodes
- •2.6.1 Introduction
- •2.6.2 PIN Photodiodes
- •2.6.3 Avalanche Photodiodes
- •2.6.4 Signal Conditioning Circuits for Photodiodes
- •2.6.5 Photoconductors
- •2.6.6 LEDs
- •2.6.7 Laser Diodes
- •2.7 Chapter Summary
- •Home Problems
- •3.1 Introduction
- •3.2 DA Circuit Architecture
- •3.4 CM and DM Gain of Simple DA Stages at High Frequencies
- •3.4.1 Introduction
- •3.5 Input Resistance of Simple Transistor DAs
- •3.7 How Op Amps Can Be Used To Make DAs for Medical Applications
- •3.7.1 Introduction
- •3.8 Chapter Summary
- •Home Problems
- •4.1 Introduction
- •4.3 Some Effects of Negative Voltage Feedback
- •4.3.1 Reduction of Output Resistance
- •4.3.2 Reduction of Total Harmonic Distortion
- •4.3.4 Decrease in Gain Sensitivity
- •4.4 Effects of Negative Current Feedback
- •4.5 Positive Voltage Feedback
- •4.5.1 Introduction
- •4.6 Chapter Summary
- •Home Problems
- •5.1 Introduction
- •5.2.1 Introduction
- •5.2.2 Bode Plots
- •5.5.1 Introduction
- •5.5.3 The Wien Bridge Oscillator
- •5.6 Chapter Summary
- •Home Problems
- •6.1 Ideal Op Amps
- •6.1.1 Introduction
- •6.1.2 Properties of Ideal OP Amps
- •6.1.3 Some Examples of OP Amp Circuits Analyzed Using IOAs
- •6.2 Practical Op Amps
- •6.2.1 Introduction
- •6.2.2 Functional Categories of Real Op Amps
- •6.3.1 The GBWP of an Inverting Summer
- •6.4.3 Limitations of CFOAs
- •6.5 Voltage Comparators
- •6.5.1 Introduction
- •6.5.2. Applications of Voltage Comparators
- •6.5.3 Discussion
- •6.6 Some Applications of Op Amps in Biomedicine
- •6.6.1 Introduction
- •6.6.2 Analog Integrators and Differentiators
- •6.7 Chapter Summary
- •Home Problems
- •7.1 Introduction
- •7.2 Types of Analog Active Filters
- •7.2.1 Introduction
- •7.2.3 Biquad Active Filters
- •7.2.4 Generalized Impedance Converter AFs
- •7.3 Electronically Tunable AFs
- •7.3.1 Introduction
- •7.3.3 Use of Digitally Controlled Potentiometers To Tune a Sallen and Key LPF
- •7.5 Chapter Summary
- •7.5.1 Active Filters
- •7.5.2 Choice of AF Components
- •Home Problems
- •8.1 Introduction
- •8.2 Instrumentation Amps
- •8.3 Medical Isolation Amps
- •8.3.1 Introduction
- •8.3.3 A Prototype Magnetic IsoA
- •8.4.1 Introduction
- •8.6 Chapter Summary
- •9.1 Introduction
- •9.2 Descriptors of Random Noise in Biomedical Measurement Systems
- •9.2.1 Introduction
- •9.2.2 The Probability Density Function
- •9.2.3 The Power Density Spectrum
- •9.2.4 Sources of Random Noise in Signal Conditioning Systems
- •9.2.4.1 Noise from Resistors
- •9.2.4.3 Noise in JFETs
- •9.2.4.4 Noise in BJTs
- •9.3 Propagation of Noise through LTI Filters
- •9.4.2 Spot Noise Factor and Figure
- •9.5.1 Introduction
- •9.6.1 Introduction
- •9.7 Effect of Feedback on Noise
- •9.7.1 Introduction
- •9.8.1 Introduction
- •9.8.2 Calculation of the Minimum Resolvable AC Input Voltage to a Noisy Op Amp
- •9.8.5.1 Introduction
- •9.8.5.2 Bridge Sensitivity Calculations
- •9.8.7.1 Introduction
- •9.8.7.2 Analysis of SNR Improvement by Averaging
- •9.8.7.3 Discussion
- •9.10.1 Introduction
- •9.11 Chapter Summary
- •Home Problems
- •10.1 Introduction
- •10.2 Aliasing and the Sampling Theorem
- •10.2.1 Introduction
- •10.2.2 The Sampling Theorem
- •10.3 Digital-to-Analog Converters (DACs)
- •10.3.1 Introduction
- •10.3.2 DAC Designs
- •10.3.3 Static and Dynamic Characteristics of DACs
- •10.4 Hold Circuits
- •10.5 Analog-to-Digital Converters (ADCs)
- •10.5.1 Introduction
- •10.5.2 The Tracking (Servo) ADC
- •10.5.3 The Successive Approximation ADC
- •10.5.4 Integrating Converters
- •10.5.5 Flash Converters
- •10.6 Quantization Noise
- •10.7 Chapter Summary
- •Home Problems
- •11.1 Introduction
- •11.2 Modulation of a Sinusoidal Carrier Viewed in the Frequency Domain
- •11.3 Implementation of AM
- •11.3.1 Introduction
- •11.3.2 Some Amplitude Modulation Circuits
- •11.4 Generation of Phase and Frequency Modulation
- •11.4.1 Introduction
- •11.4.3 Integral Pulse Frequency Modulation as a Means of Frequency Modulation
- •11.5 Demodulation of Modulated Sinusoidal Carriers
- •11.5.1 Introduction
- •11.5.2 Detection of AM
- •11.5.3 Detection of FM Signals
- •11.5.4 Demodulation of DSBSCM Signals
- •11.6 Modulation and Demodulation of Digital Carriers
- •11.6.1 Introduction
- •11.6.2 Delta Modulation
- •11.7 Chapter Summary
- •Home Problems
- •12.1 Introduction
- •12.2.1 Introduction
- •12.2.2 The Analog Multiplier/LPF PSR
- •12.2.3 The Switched Op Amp PSR
- •12.2.4 The Chopper PSR
- •12.2.5 The Balanced Diode Bridge PSR
- •12.3 Phase Detectors
- •12.3.1 Introduction
- •12.3.2 The Analog Multiplier Phase Detector
- •12.3.3 Digital Phase Detectors
- •12.4 Voltage and Current-Controlled Oscillators
- •12.4.1 Introduction
- •12.4.2 An Analog VCO
- •12.4.3 Switched Integrating Capacitor VCOs
- •12.4.6 Summary
- •12.5 Phase-Locked Loops
- •12.5.1 Introduction
- •12.5.2 PLL Components
- •12.5.3 PLL Applications in Biomedicine
- •12.5.4 Discussion
- •12.6 True RMS Converters
- •12.6.1 Introduction
- •12.6.2 True RMS Circuits
- •12.7 IC Thermometers
- •12.7.1 Introduction
- •12.7.2 IC Temperature Transducers
- •12.8 Instrumentation Systems
- •12.8.1 Introduction
- •12.8.5 Respiratory Acoustic Impedance Measurement System
- •12.9 Chapter Summary
- •References
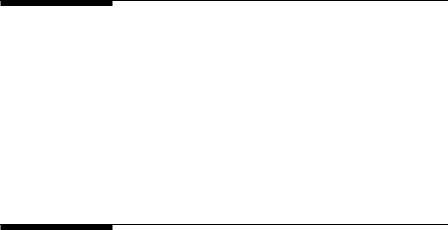
Sources and Properties of Biomedical Signals |
13 |
noise (Northrop, 2002, 2003). Evoked transient electrical response can be recovered by averaging even when the input SNR to the averager is as low as −60 dB.
1.6.3Other Body Surface Potentials
The electrooculogram (EOG), electroretinogram (ERG), and electrocochleogram (ECoG) are transient, low-amplitude, low-bandwidth potentials recorded for diagnostic and research purposes (Northrop, 2002). Each transient waveform is generally accompanied by unwanted, uncorrelated noise from EMGs and from the electrodes. The EOG is the largest of these three potentials, with a peak on the order of single millivolt. Thus, an ECG amplifier can be used with a 0.05 to 100 Hz −3-dB bandwidth and gain of 103. Averaging is generally not required.
The ERG, on the other hand, has a peak amplitude on the order of hundreds of microvolts and accompanying noise makes signal averaging expeditious. The ERG preamplifier generally has a band pass of 0.3 to 300 Hz, a gain of between 103 and 104, and an input impedance of at least 10 MΩ.
The electrocochleogram is the lowest amplitude transient, with a peak of only approximately 6 μV and waveform features of <1 μV. Signal averaging must be used to resolve the ECoG evoked transient. The signal conditioning amplifier has a midband gain of 104 and −3-dB frequencies of 5 and 3 kHz. The ECoG amplifier band pass is defined by 12 dB/octave (two-pole) filters.
1.7Discussion
The preceding descriptions indicate that the frequency content of endogenous signals from the body ranges from near dc to about 3 kHz. These signals are accompanied by noise, which means that linear filtering to improve the SNRin can often help. Some signals, such as ECoG and evoked brain cortical transients, require signal averaging for meaningful resolution. Endogenous signal peak amplitudes range from over 100 mV for nerve and muscle transmembrane potentials recorded with glass micropipette electrodes to less than a microvolt for evoked cortical transients recorded on the scalp.
1.8Electrical Properties of Bioelectrodes
To record biopotentials, an interface is needed between the electron-conduct- ing copper wires connected to signal conditioning amplifiers and the ion-conducting, “wet” environment of living animals. Electrodes form this
© 2004 by CRC Press LLC

14 |
Analysis and Application of Analog Electronic Circuits |
interface. Some of the many kinds of electrodes are better than others in terms of low noise and ease of use. Early ECG electrodes were nondisposable, nickel–silver-plated copper, or stainless steel disks hard-wired to the amplifier input leads. Although conductive gel was used, this type of electrode had inherently high low-frequency noise due to the complex redox reactions taking place at the metal electrode surfaces. Still, acceptable ECG and EMG signals could be recorded.
Present practice in measuring ECG and EMG signals from the skin surface is to use disposable sticky patch electrodes. Patch electrodes use a silverΩsilver chloride interface to a conductive gel containing Na+, K+, and Cl– ions. The gel makes direct, wet contact with the skin. Adhesive for the skin is found in a ring surrounding the electrolyte and AgCl. The copper wire makes contact with the Ag metal backing of the electrode, generally with a snap connector. More recently, skin electrodes have used AgΩAgCl deposited in a thin layer on an approximately 2.5-cm square of thin plastic film. The conductive gel and adhesive are combined in a layer all over the AgCl film. Contact with this inexpensive electrode is made with a miniature metal alligator clip on one raised corner.
Silver chloride is used with skin patch electrodes and many other types because the dc half-cell potential of the AgΩAgCl electrode depends on the logarithm of the concentration of chloride ions (Webster, 1992). Because the AgCl is in direct contact with the coupling gel, which has a high concentration of Cl− ions, the dc half-cell potential of the electrode remains fairly stable and has a low impedance, thus low thermal noise.
Figure 1.5 illustrates the electrical characteristics of a pair of AgΩAgCl electrodes facing each other. The impedance measured as a function of frequency, Ω2 Zel(f) Ω suggests that each electrode can be modeled by a parallel R–C circuit in series with a resistance. Analysis of the impedance magnitude in this figure reveals that RG = 65 Ω, Ri = 1935 Ω, and Ci = 0.274 μF for one electrode. The skin also adds a parallel R–C circuit to the electrode’s equivalent circuit.
In general, it is desirable for the electrode Z to be as small as possible. Both resistors in the electrode model make thermal (white) noise. At low frequencies
where the capacitive reactance is 1935 Ω, one electrode’s root noise spectrum is 4kT 2000 = 5.76 nV RMS/ Hz — the same order of magnitude as an
amplifier’s equivalent short-circuit input noise. If the gel dries out during prolonged use, the Zel will rise, as will the white noise from its real part.
Another type of electrode used in neurophysiological research is the salinefilled, glass micropipette electrode used for recording transmembrane potentials in neurons and muscle fibers. Because the tips of these electrodes are drawn down to diameters of a fraction of a micron before filling, their resistances when filled can range from approximately 20 to 103 MΩ, depending on the tip geometry, the filling medium, and the surround medium in which the tip is placed. Because of their high series resistances, glass micropipette electrodes create three major problems not seen with other types of bioelectrodes:
© 2004 by CRC Press LLC
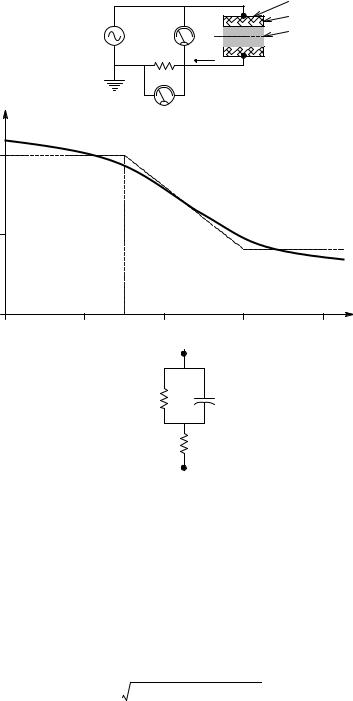
Sources and Properties of Biomedical Signals |
|
15 |
||
|
Measurement circuit |
|
||
|
|
|
|
Ag |
|
|
|
|
AgCl |
Oscillator |
Voltmeter |
+ |
|
Gel |
|
Ve |
|||
|
|
|
|
1Ω Ie
(2 electrodes)
|
|
|
A |
|
|
Ze(f) |
V Ie |
|
|
|
(ohms) |
|
|
|
103 |
|
|
|
|
102 |
|
|
|
|
|
|
B |
|
|
|
|
|
|
f (Hz) |
10 |
102 |
103 |
104 |
105 |
10 |
||||
|
|
|
Ze |
|
AC equivalent circuit for one electrode
Ri |
Ci |
C
RG
FIGURE 1.5
(A) Impedance magnitude measurement circuit for a pair of face-to-face, silver–silver chloride skin surface electrodes. (B) Typical impedance magnitude for the pair of electrodes in series. (C) Linear equivalent circuit for one electrode.
1.Because of their high series resistances, they must be used with special signal conditioning amplifiers called electrometer amplifiers,
which have ultra-low, input dc bias currents. Electrometer input bias currents are on the order of 10 fA (10−14 A) and their input resistances are approximately 1015 Ω.
2.Glass micropipette electrodes make an awesome amount of Johnson noise. For example, a 200 MΩ electrode at 300 K, with a noise band-
width of 3 kHz, makes 4kT ∞ 2 ∞ 108 ∞ 3 ∞ 103 = 99.68 μV RMS of noise.
© 2004 by CRC Press LLC
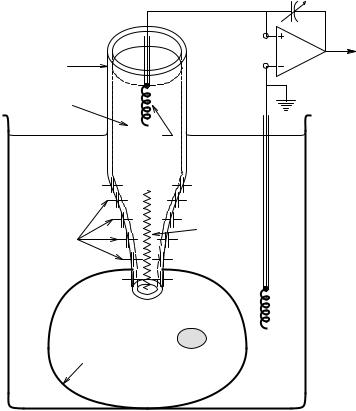
16 |
Analysis and Application of Analog Electronic Circuits |
CN
E
Vo
EA
Glass tube |
R |
Filling electrolyte
AgCl electrode
Extracellular electrolyte
Shunting |
|
|
|
|
|
Tip resistance |
|
|||||
capacitance |
|
|
|
|
|
|
|
|
|
|||
|
|
|
|
+ |
+ |
+ |
+ + |
+ |
|
|
|
|
|
|
|
+ |
|
− |
− − |
+ + |
|
|
|
||
|
|
+ |
− |
− |
− − |
|
|
|
||||
|
+ |
|
− |
|
|
|
|
|
− + |
|
|
|
|
− |
|
|
|
|
|
|
|
||||
+ |
|
|
|
|
|
− |
+ |
|
|
|||
− |
|
|
|
|
|
|
|
|
||||
+ |
|
|
|
|
|
|
|
− |
|
|
||
+ |
− |
Cell cytoplasm |
|
|
− |
+ |
|
|||||
− |
|
|
|
|
+ |
|
||||||
|
|
|
|
|
Nucleus |
− |
External |
|||||
+ |
− |
|
|
|
|
|
||||||
|
|
|
|
|
|
|
|
|||||
|
|
|
|
|
|
|
− |
+ AgCl |
||||
+ |
− |
|
|
|
|
|
|
|
||||
|
|
Cell membrane |
|
|
− |
|
+ |
electrode |
||||
+ |
− |
|
|
|
|
|
||||||
|
|
|
|
|
|
−− |
+ |
|
|
|||
|
+ |
− |
|
|
|
|
− |
+ |
|
|
|
|
|
|
− |
|
|
|
|
|
|
||||
|
|
+ |
− |
|
−+ |
+ |
|
|
|
|||
|
|
+ |
+ |
|
|
|
|
FIGURE 1.6
Schematic cross section (not to scale) of an electrolyte-filled, glass micropipette electrode inserted into the cytoplasm of a cell. AgΩAgCl electrodes are used to interface recording wires (generally Cu) with the electrolytes.
3.The tips of glass micropipette electrodes have significant distributed capacitance between the electrolyte inside and the electrolyte outside the tip (see Figure 1.6), which makes them behave like distributedparameter low-pass filters, as shown in Figure 1.7(A).
This figure also illustrates the equivalent circuits of the AgΩAgCl coupling electrodes, the cell membrane, and the microelectrode tip spreading resistance and tip EMF. The distributed resistance of the internal electrolyte in the tip, Rtip, plus the tip spreading resistance, Rtc, plus the cell membrane’s resistance, 1/Gc, are orders of magnitude larger than the impedances associated with the AgCl coupling electrodes. Thus, they can be lumped together as a single Rμ and the distributed tip capacitance can be represented by a
single, lumped Cμ in the simplified R–C LPF of Figure 1.7(B). Vbio is the bioelectric EMF across the cell membrane in the vicinity of the microelectrode tip. The break frequency of the B circuit is simply fb = 1/(2πRμCμ) Hz.
© 2004 by CRC Press LLC