
- •COMPUTATIONAL CHEMISTRY
- •CONTENTS
- •PREFACE
- •1.1 WHAT YOU CAN DO WITH COMPUTATIONAL CHEMISTRY
- •1.2 THE TOOLS OF COMPUTATIONAL CHEMISTRY
- •1.3 PUTTING IT ALL TOGETHER
- •1.4 THE PHILOSOPHY OF COMPUTATIONAL CHEMISTRY
- •1.5 SUMMARY OF CHAPTER 1
- •REFERENCES
- •EASIER QUESTIONS
- •HARDER QUESTIONS
- •2.1 PERSPECTIVE
- •2.2 STATIONARY POINTS
- •2.3 THE BORN–OPPENHEIMER APPROXIMATION
- •2.4 GEOMETRY OPTIMIZATION
- •2.6 SYMMETRY
- •2.7 SUMMARY OF CHAPTER 2
- •REFERENCES
- •EASIER QUESTIONS
- •HARDER QUESTIONS
- •3.1 PERSPECTIVE
- •3.2 THE BASIC PRINCIPLES OF MM
- •3.2.1 Developing a forcefield
- •3.2.2 Parameterizing a forcefield
- •3.2.3 A calculation using our forcefield
- •3.3 EXAMPLES OF THE USE OF MM
- •3.3.2 Geometries and energies of polymers
- •3.3.3 Geometries and energies of transition states
- •3.3.4 MM in organic synthesis
- •3.3.5 Molecular dynamics and Monte Carlo simulations
- •3.4 GEOMETRIES CALCULATED BY MM
- •3.5 FREQUENCIES CALCULATED BY MM
- •3.6 STRENGTHS AND WEAKNESSES OF MM
- •3.6.1 Strengths
- •3.6.2 Weaknesses
- •3.7 SUMMARY OF CHAPTER 3
- •REFERENCES
- •EASIER QUESTIONS
- •HARDER QUESTIONS
- •4.1 PERSPECTIVE
- •4.2.1 The origins of quantum theory: blackbody radiation and the photoelectric effect
- •4.2.2 Radioactivity
- •4.2.3 Relativity
- •4.2.4 The nuclear atom
- •4.2.5 The Bohr atom
- •4.2.6 The wave mechanical atom and the Schrödinger equation
- •4.3.1 Introduction
- •4.3.2 Hybridization
- •4.3.3 Matrices and determinants
- •4.3.4 The simple Hückel method – theory
- •4.3.5 The simple Hückel method – applications
- •4.3.6 Strengths and weaknesses of the SHM
- •4.4.1 Theory
- •4.4.2 An illustration of the EHM: the protonated helium molecule
- •4.4.3 The extended Hückel method – applications
- •4.4.4 Strengths and weaknesses of the EHM
- •4.5 SUMMARY OF CHAPTER 4
- •REFERENCES
- •EASIER QUESTIONS
- •5.1 PERSPECTIVE
- •5.2.1 Preliminaries
- •5.2.2 The Hartree SCF method
- •5.2.3 The HF equations
- •5.2.3.1 Slater determinants
- •5.2.3.2 Calculating the atomic or molecular energy
- •5.2.3.3 The variation theorem (variation principle)
- •5.2.3.4 Minimizing the energy; the HF equations
- •5.2.3.5 The meaning of the HF equations
- •5.2.3.6a Deriving the Roothaan–Hall equations
- •5.3 BASIS SETS
- •5.3.1 Introduction
- •5.3.2 Gaussian functions; basis set preliminaries; direct SCF
- •5.3.3 Types of basis sets and their uses
- •5.4 POST-HF CALCULATIONS: ELECTRON CORRELATION
- •5.4.1 Electron correlation
- •5.4.3 The configuration interaction approach to electron correlation
- •5.5.1 Geometries
- •5.5.2 Energies
- •5.5.2.1 Energies: Preliminaries
- •5.5.2.2 Energies: calculating quantities relevant to thermodynamics and to kinetics
- •5.5.2.2a Thermodynamics; “direct” methods, isodesmic reactions
- •5.5.2.2b Thermodynamics; high-accuracy calculations
- •5.5.2.3 Thermodynamics; calculating heats of formation
- •5.5.2.3a Kinetics; calculating reaction rates
- •5.5.2.3b Energies: concluding remarks
- •5.5.3 Frequencies
- •Dipole moments
- •Charges and bond orders
- •Electrostatic potential
- •Atoms-in-molecules
- •5.5.5 Miscellaneous properties – UV and NMR spectra, ionization energies, and electron affinities
- •5.5.6 Visualization
- •5.6 STRENGTHS AND WEAKNESSES OF AB INITIO CALCULATIONS
- •5.7 SUMMARY OF CHAPTER 5
- •REFERENCES
- •EASIER QUESTIONS
- •HARDER QUESTIONS
- •6.1 PERSPECTIVE
- •6.2 THE BASIC PRINCIPLES OF SCF SE METHODS
- •6.2.1 Preliminaries
- •6.2.2 The Pariser-Parr-Pople (PPP) method
- •6.2.3 The complete neglect of differential overlap (CNDO) method
- •6.2.4 The intermediate neglect of differential overlap (INDO) method
- •6.2.5 The neglect of diatomic differential overlap (NDDO) method
- •6.2.5.2 Heats of formation from SE electronic energies
- •6.2.5.3 MNDO
- •6.2.5.7 Inclusion of d orbitals: MNDO/d and PM3t; explicit electron correlation: MNDOC
- •6.3 APPLICATIONS OF SE METHODS
- •6.3.1 Geometries
- •6.3.2 Energies
- •6.3.2.1 Energies: preliminaries
- •6.3.2.2 Energies: calculating quantities relevant to thermodynamics and kinetics
- •6.3.3 Frequencies
- •6.3.4 Properties arising from electron distribution: dipole moments, charges, bond orders
- •6.3.5 Miscellaneous properties – UV spectra, ionization energies, and electron affinities
- •6.3.6 Visualization
- •6.3.7 Some general remarks
- •6.4 STRENGTHS AND WEAKNESSES OF SE METHODS
- •6.5 SUMMARY OF CHAPTER 6
- •REFERENCES
- •EASIER QUESTIONS
- •HARDER QUESTIONS
- •7.1 PERSPECTIVE
- •7.2 THE BASIC PRINCIPLES OF DENSITY FUNCTIONAL THEORY
- •7.2.1 Preliminaries
- •7.2.2 Forerunners to current DFT methods
- •7.2.3.1 Functionals: The Hohenberg–Kohn theorems
- •7.2.3.2 The Kohn–Sham energy and the KS equations
- •7.2.3.3 Solving the KS equations
- •7.2.3.4a The local density approximation (LDA)
- •7.2.3.4b The local spin density approximation (LSDA)
- •7.2.3.4c Gradient-corrected functionals and hybrid functionals
- •7.3 APPLICATIONS OF DENSITY FUNCTIONAL THEORY
- •7.3.1 Geometries
- •7.3.2 Energies
- •7.3.2.1 Energies: preliminaries
- •7.3.2.2 Energies: calculating quantities relevant to thermodynamics and kinetics
- •7.3.2.2a Thermodynamics
- •7.3.2.2b Kinetics
- •7.3.3 Frequencies
- •7.3.6 Visualization
- •7.4 STRENGTHS AND WEAKNESSES OF DFT
- •7.5 SUMMARY OF CHAPTER 7
- •REFERENCES
- •EASIER QUESTIONS
- •HARDER QUESTIONS
- •8.1 FROM THE LITERATURE
- •8.1.1.1 Oxirene
- •8.1.1.2 Nitrogen pentafluoride
- •8.1.1.3 Pyramidane
- •8.1.1.4 Beyond dinitrogen
- •8.1.2 Mechanisms
- •8.1.2.1 The Diels–Alder reaction
- •8.1.2.2 Abstraction of H from amino acids by the OH radical
- •8.1.3 Concepts
- •8.1.3.1 Resonance vs. inductive effects
- •8.1.3.2 Homoaromaticity
- •8.2 TO THE LITERATURE
- •8.2.1 Books
- •8.2.2 The Worldwide Web
- •8.3 SOFTWARE AND HARDWARE
- •8.3.1 Software
- •8.3.2 Hardware
- •8.3.3 Postscript
- •REFERENCES
- •INDEX

268 Computational Chemistry
5.5.2.2Energies: calculating quantities relevant to thermodynamics and to kinetics
5.5.2.2a Thermodynamics; “direct” methods, isodesmic reactions
Here we are concerned with the relative energies of species other than transition states. Such molecules are sometimes called “stable species,” even if they are not at all stable in the usual sense, to distinguish them from transition states, which exist only for an instant on the way from reactants to products, A “stable species,” in contrast, sits in a potential energy well and survives at least a few molecular vibrations The very useful book by Hehre [32] contains a wealth of information on computational and experimental results concerning thermodynamic quantities.
The ab initio reaction energy that is most commonly calculated is simply the difference in ZPE-corrected energies, which is the reaction enthalpy change at 0 K (Eq. (5.176)). This provides an easily-obtained indication of whether a reaction is likely to be exothermic or endothermic, or of the relative stabilities of isomers. Table 5.9 illustrates this procedure. The results are only semiquantitatively correct, and the HF/6-31G* method is not necessarily better here than the HF/3-21G. In fact, it has been documented by extensive calculations that such HF/3-21G and HF/6-31G* energy differences generally give only a rough indication of energy changes. Much better results are obtained from MP2/6-31G* calculations on MP2/6-31G*, HF/3-21G* or even semiempirical AM1 geometries, and it is well worth consulting the book by Hehre for details [108].
To get the best results from relatively low-level calculations, one can utilize isodesmic reactions (Greek: “same bond,” i.e. similar bonding on both sides of the equation). These are reactions in which the number of each kind of bond and each kind of lone pair is conserved. For example,
and
are isodesmic reactions; the first one has on each side 6 N–H bonds, one C–N bond and one nitrogen lone pair, and the second has on each side 6 C–H and 2 C–F bonds. The reaction
is, strictly speaking, not isodesmic, since although it has the same number ofbonds, even the same number of single bonds, on both sides, there are 6 C–H, one C–C, and 2 H–H bonds on one side and 8 C–H bonds on the other. Note that an isodesmic reaction does not have to be experimentally realizable: it is an artifice to obtain a reasonably accurate energy difference by ensuring that as far as possible errors cancel. This will happen to the extent that particular errors are associated with particular structural features; electron correlation effects are thought to be especially important in calculating energy differences, and such effects tend to cancel when the number of electron pairs of each kind is conserved.
Reactions like (5.182) give fairly good quantitative results for the proton affinities (essentially, the basicity) of nitrogen bases [109], byusing to deprotonate a series
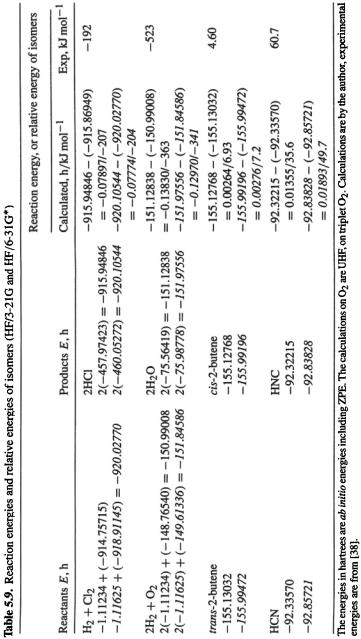
Ab initio calculations 269

270 Computational Chemistry
oftheconjugate acids. Isodesmic processes like (5.183) have been used to reveal the nature and amount of interaction between groups X and Y on an
carbon [110]. IfX and Y interact in a stabilizing way, then separating them should be reflected in an endothermic reaction, and conversely mutually destabilizing groups should give an exothermic reaction. For reaction (5.183) at the 3-21G level the product energies minus the reactant energies are
i.e. the reaction is endothermic by
while for the corresponding
reaction the reaction energy is
which indicates a qualitatively different mutual interaction between two fluorines, as compared to two chlorines, on the same carbon (actually, a bigger basis set indicates that the Cl/Cl interaction in
is about zero). Another application of isodesmic reactions has been to estimating aromatic character [111].
There is no unique isodesmic reaction for a particular problem. For example, to compare the ring strain in oxacyclopropane (oxirane, ethylene oxide) with that in cyclopropane, one might calculate the reaction energy of the oxygen exchange reaction (remember that isodesmic reactions do not have to be experimentally achievable):
Here the equilibrium should favor the less-strained ring. Alternatively, one might compare the reaction energies of the two cleavage reactions:
Here, the reaction that forms the less-strained ring should be the less endothermic (or the more exothermic, if the reactions turn out to be exothermic).
Let us calculate the reaction energies for the three reactions. For (5.185), the HF/6-31G* (including ZPE) energies are
E(pdts) – E(reactants)
The reaction is calculated to be exothermic by and the simple interpretation is that cyclopropane is more strained than oxacyclopropane by
To compare oxacyclopropane and cyclopropane using (5.186) and (5.187), we do need not calculate the energies of
and
since these will cancel out:

Ab initio calculations 271
and for (187):
Reaction (5.187) is more endothermic than reaction (5.186)by 1.18152 – 1.17357 h = 0.00795 h = indicating that cyclopropane is more strained than oxacyclopropane by this amount [112]. The agreement between the two approaches is not coincidental, since the cancellation of the methane and the ethane energies makes these two conceptually different approaches mathematically identical. Note, however, that for any isodesmic reaction we can always write some non-equivalent (unlike the case of (5.185) vs. (5.186)/(5.187)) isodesmic process to get the desired quantity. Also, different isodesmic reactions will give somewhat different results; this is essentially because the “reagents” of one reaction will not be calculated to exactly the same degree of accuracy as those of another reaction.
As we saw in section 5.4.1, calculation of homolytic cleavage energies by simply subtracting HF energies gives very poor results. Let us calculate a homolytic bond dissociation energy by an isodesmic-type approach. The idea is to combine the desired homolytic reaction with one of known (either from experiment or from as high-level calculation) energy in a reaction which, although not strictly isodesmic, conserves the number ofsingle, double, etc. bonds and the numberofunpaired electrons. Forexample,
suppose we want E(C--O), the C–O bond dissociation energy in |
We might |
utilize the scheme |
|
Here E(C–C) and E(C–O) are homolytic dissociation energies(bond energies) and E(iso) is the energy of the isodesmic-type reaction shown. This reaction is not strictly isodesmic, since a C–C bond is replaced by a C–O bond, but it does have the same number of unpaired electrons(one) on each side, so net correlation effects should be much less than for a reaction in which two unpaired electrons are created from a bond. A reaction in which the number of unpaired electrons is conserved is called an isogyric(Greek, “same spin”) reaction. Since the overall energy change in a process is independent of the path connecting the initial and final states [113] we write
i.e.
E(C–C) must be known, and E(iso) is to be obtained by calculation. Taking an experimental value of for the bond energy of the ethane C–C bond [61], and