
- •Preface
- •Acknowledgments
- •Contents
- •Contributors
- •1. Introduction
- •2. Evaluation of the Craniomaxillofacial Deformity Patient
- •3. Craniofacial Deformities: Review of Etiologies, Distribution, and Their Classification
- •4. Etiology of Skeletal Malocclusion
- •5. Etiology, Distribution, and Classification of Craniomaxillofacial Deformities: Traumatic Defects
- •6. Etiology, Distribution, and Classification of Craniomaxillofacial Deformities: Review of Nasal Deformities
- •7. Review of Benign Tumors of the Maxillofacial Region and Considerations for Bone Invasion
- •8. Oral Malignancies: Etiology, Distribution, and Basic Treatment Considerations
- •9. Craniomaxillofacial Bone Infections: Etiologies, Distributions, and Associated Defects
- •11. Craniomaxillofacial Bone Healing, Biomechanics, and Rigid Internal Fixation
- •12. Metal for Craniomaxillofacial Internal Fixation Implants and Its Physiological Implications
- •13. Bioresorbable Materials for Bone Fixation: Review of Biological Concepts and Mechanical Aspects
- •14. Advanced Bone Healing Concepts in Craniomaxillofacial Reconstructive and Corrective Bone Surgery
- •15. The ITI Dental Implant System
- •16. Localized Ridge Augmentation Using Guided Bone Regeneration in Deficient Implant Sites
- •17. The ITI Dental Implant System in Maxillofacial Applications
- •18. Maxillary Sinus Grafting and Osseointegration Surgery
- •19. Computerized Tomography and Its Use for Craniomaxillofacial Dental Implantology
- •20B. Atlas of Cases
- •21A. Prosthodontic Considerations in Dental Implant Restoration
- •21B. Overdenture Case Reports
- •22. AO/ASIF Mandibular Hardware
- •23. Aesthetic Considerations in Reconstructive and Corrective Craniomaxillofacial Bone Surgery
- •24. Considerations for Reconstruction of the Head and Neck Oncologic Patient
- •25. Autogenous Bone Grafts in Maxillofacial Reconstruction
- •26. Current Practice and Future Trends in Craniomaxillofacial Reconstructive and Corrective Microvascular Bone Surgery
- •27. Considerations in the Fixation of Bone Grafts for the Reconstruction of Mandibular Continuity Defects
- •28. Indications and Technical Considerations of Different Fibula Grafts
- •29. Soft Tissue Flaps for Coverage of Craniomaxillofacial Osseous Continuity Defects with or Without Bone Graft and Rigid Fixation
- •30. Mandibular Condyle Reconstruction with Free Costochondral Grafting
- •31. Microsurgical Reconstruction of Large Defects of the Maxilla, Midface, and Cranial Base
- •32. Condylar Prosthesis for the Replacement of the Mandibular Condyle
- •33. Problems Related to Mandibular Condylar Prosthesis
- •34. Reconstruction of Defects of the Mandibular Angle
- •35. Mandibular Body Reconstruction
- •36. Marginal Mandibulectomy
- •37. Reconstruction of Extensive Anterior Defects of the Mandible
- •38. Radiation Therapy and Considerations for Internal Fixation Devices
- •39. Management of Posttraumatic Osteomyelitis of the Mandible
- •40. Bilateral Maxillary Defects: THORP Plate Reconstruction with Removable Prosthesis
- •41. AO/ASIF Craniofacial Fixation System Hardware
- •43. Orbital Reconstruction
- •44. Nasal Reconstruction Using Bone Grafts and Rigid Internal Fixation
- •46. Orthognathic Examination
- •47. Considerations in Planning for Bimaxillary Surgery and the Implications of Rigid Internal Fixation
- •48. Reconstruction of Cleft Lip and Palate Osseous Defects and Deformities
- •49. Maxillary Osteotomies and Considerations for Rigid Internal Fixation
- •50. Mandibular Osteotomies and Considerations for Rigid Internal Fixation
- •51. Genioplasty Techniques and Considerations for Rigid Internal Fixation
- •52. Long-Term Stability of Maxillary and Mandibular Osteotomies with Rigid Internal Fixation
- •53. Le Fort II and Le Fort III Osteotomies for Midface Reconstruction and Considerations for Internal Fixation
- •54. Craniofacial Deformities: Introduction and Principles of Management
- •55. The Effects of Plate and Screw Fixation on the Growing Craniofacial Skeleton
- •56. Calvarial Bone Graft Harvesting Techniques: Considerations for Their Use with Rigid Fixation Techniques in the Craniomaxillofacial Region
- •57. Crouzon Syndrome: Basic Dysmorphology and Staging of Reconstruction
- •58. Hemifacial Microsomia
- •59. Orbital Hypertelorism: Surgical Management
- •60. Surgical Correction of the Apert Craniofacial Deformities
- •Index
13
Bioresorbable Materials for Bone Fixation: Review of Biological Concepts and Mechanical Aspects
Riitta Suuronen and Christian Lindqvist
The development of biodegradable devices for fracture fixation and guided bone regeneration has been intense for three decades. An optimal device should not cause any local or systemic disorders and should degrade slowly, transferring the stress to the healing bone. Hence, it need not be removed after the fracture or defect has healed.
The first material to be used as biodegradable suture was catgut (collagen), which degrades proteolytically and disappears from tissue via phagocytosis, thus causing a local inflammation in the tissue. Modern suture materials are made of polyhydroxy acids (polyesters). Most widely used are polylactide (PLA), polyglycolide (PGA), and polydioxanone (PDS). In fracture fixation, PGA was the first material to be used by Schmitt and Polistina,1 but their results were never reported in a scientific journal. The first reports on the use of PLA in the fixation of fractures or osteotomies were published by Cutright et al.2 and Kulkarni et al.3 Later, devices made of PGA and PDS were used successfully for fracture fixation in maxillofacial surgery.4,5
The development of PLA devices for maxillofacial surgery started in the 1970s, when Cutright et al.2 used PLA sutures and Kulkarni3 used PLA rods in the repair of experimental mandibular fractures. The following year, sheets of PLA were used in experimental blow-out fractures.6 Better knowledge in material management has led to a wide use of biodegradable materials, and today PLA screws are routinely used in our department in the fixation of sagittal split osteotomies.7
Materials
The synthetic biodegradable materials most widely used in fracturefixationarehighmolecularweightalphahydroxyacidpolymers:polydioxanone(Figure13.1),polyglycolide(Figure13.2), and polylactide (Figure 13.3). Of these, PGA and PLA have received the most interest, partly because they can be self-rein- forced to gain better strength properties.
Sterilization also contributes to the degradation rate. Ethylene oxide does not alter the polymer or cause degradation,8 but it can introduce residues to the polymer.9,10 By con-
trast, gamma radiation causes both chain-scissioring and cross-linking in the polymer11 and can change its mechanical properties.12,13 It also reduces the molecular weight of the polymer.14
Polydioxanone
Polydioxanone (Figure 13.1) is a colorless crystalline polymer. At room temperature it is rubberlike, its melting point being 110°C and glass transition temperature 16°C. It is degraded by hydrolysis, and the end products are excreted mainly in urine, some in feces, and some exhaled as CO2.15 It is completely resorbed in 6 months, and only a minimal foreign body tissue reaction in the vicinity of the implant can be seen.15–17 Implants made of PDS can be sterilized by ethylene oxide.
These properties make it well suited for sutures. Because of its flexibility, it has been used in soft tissue, tendon, and ligament surgery,18 and in orthopedic surgery (cords, pins, and screws).19–25 Only a few studies have been published of its use in maxillofacial surgery.5,26,27
Polyglycolic Acid (Polyglycolide, PGA)
PGA is a hard, brownish crystalline polymer, which is insoluble in most solvents (Figure 13.2). It has a melting point of 224–226°C and glass transition temperature of 36°C.28 It is degraded in hydrolysis, and it is also broken down by nonspecific esterases and carboxy peptidases. Monomeric units of glycolic acid can be excreted in urine or enzymatically converted to end products H2O and CO2 (Figure 13.4).29 Depending on its molecular weight, purity, and crystallinity as well as the size and shape of the implant, it loses its mechanical strength in 6 weeks and is totally resorbed in a few months.18,30 Degradation is faster in vivo than in vitro, supposedly because of cellular enzymes.31 PGA is usually sterilized by using ethylene oxide.
Because it loses its mechanical strength rather quickly, it has been used mainly in sutures (e.g., Dexon, Davis and Geck, UK) and in rods and screws in fracture fixation of cancellous bone.32–35
113
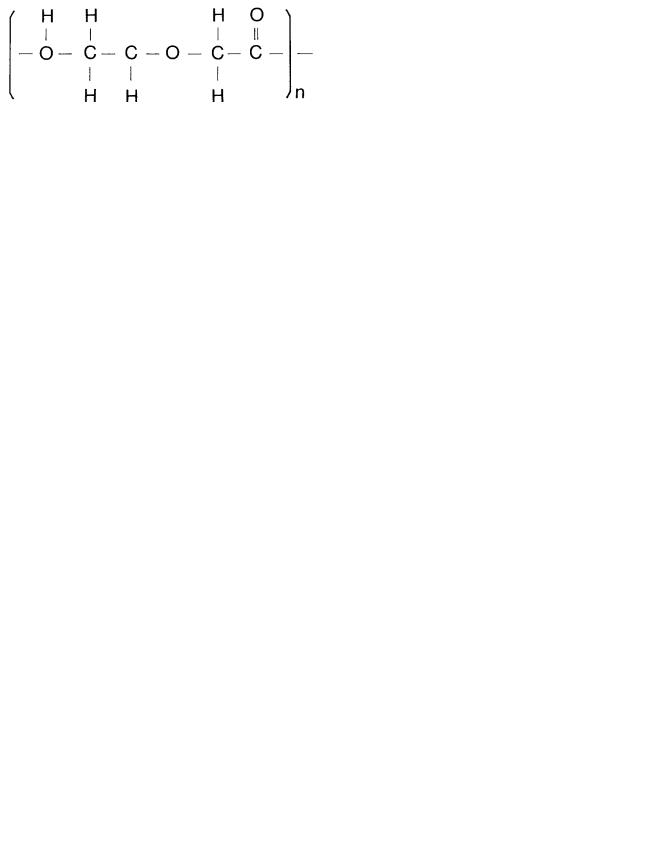
114 |
R. Suuronen and C. Lindqvist |
FIGURE 13.1 Polydioxanone (PDS).
Polylactic Acid (Polylactide, PLA)
Polylactic acid (Figure 13.3) is a pale, semicrystalline polymer with a melting point of 174°C and glass transition temperature of 57°C.8 It can exist in four forms, depending on the L and D configuration.36 It also is degraded in hydrolysis, its end products being H2O and CO2 (Figure 13.5). The strength retention time of PLA varies depending on the sterilization method and the properties of the material and implant, but it is considerably longer than PDS or PGA. The total resorption time of poly-L-lactide is several years.37,38 A copolymer of D- and L-lactide, poly-DL-lactide (PDLLA), seems to degrade somewhat faster.39 Cellular enzymes are supposed to enhance this reaction, too.31
PLA can be sterilized with ethylene oxide or steam40 and high-strength self-reinforced implants can be sterilized with gamma radiation.18 Gamma sterilization decreases significantly the molecular weight of PLA, and, hence, might enhance the degradation, resulting in shorter resorption time.18
Copolymers
PGA and PLA can be combined to form a copolymer. Its properties can be changed by varying the ratios of its components. By increasing the amount of PGA, the copolymer will degrade faster and vice versa. Today, a PLA/PGA copolymer suture material, polyglactin 910, is widely used (Vicryl®, Ethicon, Sollentuna, Sweden). Also, a copolymer of poly-L- lactide (PLLA) and poly-D-lactide (PDLA) can be manufactured, and by varying their ratio, the properties of this PDLLA can be changed.
FIGURE 13.3 Polylactide (PLA).
Self-Reinforcing Technique
The self-reinforcing (SR) technique developed by Törmälä et al.41 has enabled the manufacture of polylactide and polyglycolide implants strong enough for fracture fixation. In this technique, polymeric fibers are bound together with a matrix of the same polymer without any adhesion promoters (Figure 13.6). These implants have high initial strength values and, therefore, are suitable for fracture fixation.42
Biocompatibility and Tissue Reactions
In general, all these materials have been well tolerated in living tissue. Local tissue responses depend on the rate of degradation and the biocompatibility of the components and degradation products of the polymer.29 Cutright and Hunsuck43 found that resorption of PLA was accomplished by a peculiar phagocytic process that started at 4 weeks and was still
FIGURE 13.2 Polyglycolide (PGA). |
FIGURE 13.4 Degradation of PGA. End products are CO2 and H2O. |
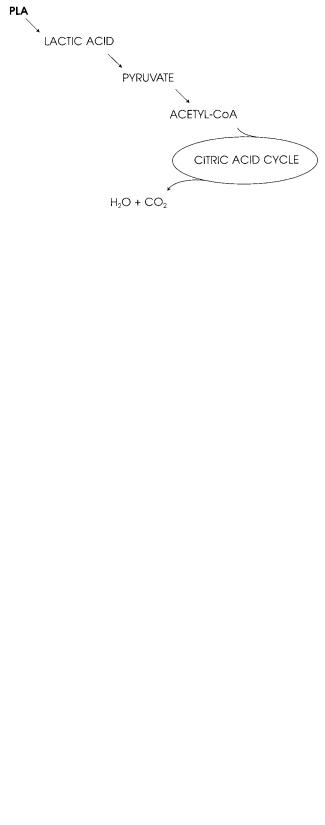
13. Bioresorbable Materials for Bone Fixation
FIGURE 13.5 Degradation of PLA. Final products are CO2 and H2O.
continuing at 38 weeks. Phagocytic cells, giant cells, and villous projections were involved in this process. PLA tissue compatibility was found to be very good. Majola et al.44 used SR-PLLA and SR-PDLLA/PLLA (40/60) rods on femoral osteotomies in rats and rabbits with follow-up times up to 2 years. Resorption of the implants started in the periphery and continued toward the center of the implant. Histologically, no evidence of inflammation or foreign body reaction could be seen. However, mild inflammatory reactions have been encountered surrounding the implant throughout the resorption period. Hatton et al.45 evaluated SR-PGA membranes in rat bone cell cultures. In 2 weeks, cells had colonized the surface of the membrane, and after 3 weeks, bone cells penetrated the weave of the membrane, forming calcified collagenous bonelike tissue. At the same time, evidence of
115
resorption of PGA could be noticed. The authors conclude that this study supports previous reports on the osteoconductive activity of this material.
PDS elicited minimal or slight foreign body reactions when implanted in subcutaneous tissue in rats.15 At 5 days, the reaction consisted primarily of macrophages and proliferating fibroblasts. At 91 days and later, no neutrophils were seen, and only macrophages and fibroblasts remained consistently present until the material was completely absorbed. After absorption, reactions were either absent or identified by the presence of a few enlarged macrophages or fibroblasts localized between otherwise normal muscle cells.
In humans, inflammatory foreign body reactions, which include a discharging sinus without infection, have been encountered in several clinical studies when PGA or PGA/PLA copolymer has been used.33,35,46–48 This is demonstrated by an uncomfortable swelling in the operation area after a fol- low-up period of approximately 12 weeks. In these cases, implants have been placed directly under the skin, in areas where there is very little subcutaneous tissue. After drainage, the swelling disappeared in 3 weeks.35 When the aspirate from the swelling was cytologically analyzed, mainly lymphocytes and some monocytes were found. The authors concluded that PGA is immunologically inert but induces mononuclear cell migration.49
Eitenmüller et al.50 reported a clinically manifest foreign body reaction to nonreinforced PLLA plates and screws after fixation of an ankle fracture. In 4 of 25 patients, small fragments of degraded plate were pushed through the overlying skin 6 to 9 months postoperatively. No signs of infection could be noticed. Bergsma et al.51 reported on a late tissue response on nonreinforced PLLA 3 years after fracture fixation in 6 of 10 patients. The plates and screws were placed directly under the skin at the frontozygomatic suture, with minimal subcutaneous tissue. The patients had clinically manifest intermittent swelling at the operation site. At reoperation, fragments of the polymer were removed and histological samples taken. In histological and electron microscopic evaluation, crystalline PLLA particles were found extracellularly and intracellularly together with numerous fibrocytes, macrophages, and giant cells. SR-PLLA pins have been used in the treatment of small-fragment fractures and osteotomies in 32 patients without signs of foreign body reaction 8 to 32 months postoperatively.52
FIGURE 13.6 The self-reinforcing technique, in which fibers and binding matrix are of the same chemical composition.
Experimental Fracture Fixation
Orbital Blowout
Cutright and Hunsuck6 used 1.5-mm-thick PLA sheets to repair experimental blowout fractures in 12 monkeys (Macaca mulatta). Bone was deposited immediately adjacent to the capsule surrounding the resorbing PLA. Healing was reported
116
normal, but residual PLA could still be detected after 38 weeks.
Rozema et al.53 studied the use of as-polymerized PLLA implants for the repair of blow-out fractures in 15 goats. The implants were concave, 0.4 mm thick and 30 mm in diameter, and they had perforations of 2 mm in diameter to allow tissue ingrowth. The artificial defects created in the bone and maxillary sinus epithelium were approximately 15 mm in diameter. Excess areas of each implant were trimmed using scissors. The implants were fixed to the infraorbital rim using one PGA suture. Follow-up times were up to 78 weeks, after which histological examinations were performed. After 3 weeks, the implants were totally covered by loose connective tissue. By 12 weeks, some growth into the perforations was evident. The epithelium at the roof of the maxillary sinus was normal. A thin layer of new bone was observable. After 19 weeks, a bony plate in apposition to the outer side of the connective tissue capsule was observed. By 78 weeks, new bone totally covered the antral and orbital sides of the implant and filled the perforations in the implant. No inflammatory reactions were seen. The authors concluded that the implant gave sufficient stability to the fracture for it to heal, but that a shorter absorption time (now estimated to be as long as 3.5 years) would be preferable.
Mandible
Sutures
In the beginning of the development of biodegradable materials, PLA sutures were used in the fixation of experimental mandibular midline fractures in Macaca mulatta.2 The suture consisted of three strands, twisted together to form a 0.35mm strand. Each 0.2-mm strand consisted of fibers of 28 mm in diameter. The healing was uneventful with a follow-up time up to 12 weeks.
Rods
Kulkarni et al.3 used 1/8-in. (3.2-mm) PDLLA extruded rods in the fixation of mandibular fractures in dogs. The healing rate was the same as in a control group, in which similar stainless steel pins were used. The rods changed color to a whitish shade after 2 weeks. At 6 weeks they were “worm-eaten.”
Plates and Screws
Getter et al.54 reported on having used four-hole plates and non–self-tapping screws in the fixation of mandibular fractures in 6 dogs. During surgery, the plates and screws were fused into a one-piece system with a warm soldering iron, which ensured the stability of the fixation and inhibited movement between the screws and the plate. The fractures healed with secondary callus formation in 4 to 6 weeks. By 32 to 40 weeks, the plates and screws were completely degraded.
Gerlach et al.55 used high-molecular-weight (800, 000) PLLA plates and screws to fix mandibular fractures in 12
R. Suuronen and C. Lindqvist
adult beagle dogs. The plates were of the Sherman type with a cross section of 4 9 15 mm and length of 45 mm. The thread diameter of the screw was 3 mm and that of the core 1.9 mm. Fracture healing was uncomplicated in all dogs, and the fractures consolidated after 4 weeks, although some screws broke. After 12 weeks, callus had disappeared.
Bos56 has published a PhD thesis on the use of high- molecular-weight PLLA plates and screws in mandibular fractures in sheep and dogs. In pilot studies in two sheep and six dogs, a special clamp was used to produce a “natural” fracture, that is, one with serrated edges of the alveolar process, buccal cortex, and inferior border of the mandibular body area. The plates were bent to match the underlying bone using a heat gun. The follow-up time was from 3 to 11 weeks. Bos used a Champy-like 4-hole plate with monocortical screws. The plate was, however, 2.0 mm thick, 37 mm long, and 8 mm wide. The screws used had a thread diameter of 2.7 mm. Bos fixed fractures on one side of the mandible and placed unloaded plates on the other side of the mandible. After the follow-up, the plates were removed, but it was impossible to remove the screws after 3 weeks because they broke in the area between the screw head and thread. The results indicated that the plates subjected to loading had lost more of their tensile strength (about 90%) in 11 weeks than those not under stress (about 80%). The fractures healed uneventfully. No callus formation was seen. The authors concluded that use of their PLLA plates and screws resulted in good stability over a sufficiently long period of time for normal bone healing to occur.
In their early studies, Törmälä et al.41,42,57 focused on developing SR-technique mainly for rod-shaped implants. The first clinical report on the use of biodegradable implants in weight-bearing fractures was that by Rokkanen et al.32 From the late 1980s biodegradable screws and plates have been used successfully for the fixation of experimental mandibular osteotomies in 87 sheep. They have been used in three different osteotomies: condylar and body osteotomies and sagittal split osteotomies. No intermaxillary fixation has been applied in any of these cases.7,58–64 These studies started by the fixation of condylar osteotomies with one SR-PLLA screw (Figures 13.7–13.9).58,63 Then a multilayer SR-PLLA plate was used in the edentulous area of the mandible (Figures 13.10 and 13.11).60,61 Finally, this multilayer plate was used with polylactide screws (Suuronen et al., unpublished data). SRPLLA screws were also used in the fixation of sagittal osteotomies.7,59 The results of these animal experiments were very encouraging, and today, SR-PLLA screws are routinely used in certain sagittal split osteotomies (Figure 13.12a–c).7
Clinical Fracture Fixation
The first clinical studies on the use of biodegradable materials in fracture surgery were carried out 20 years ago. In the beginning, the results were not very satisfying. Reports of repair of small numbers of zygomatic, mandibular, and orbital blow-
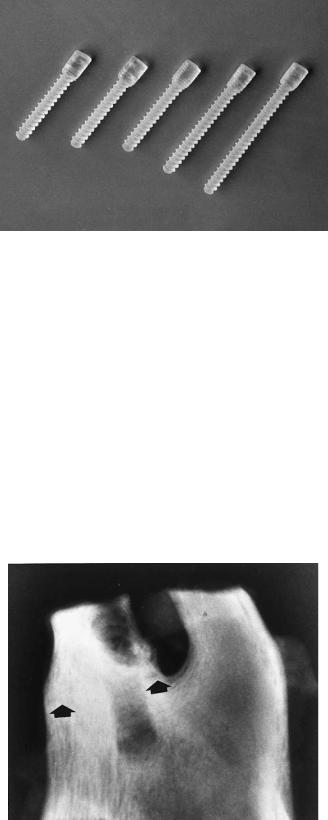
13. Bioresorbable Materials for Bone Fixation
FIGURE 13.7 SR-PLLA screws, core diameter 2.7 mm.
out fractures were published in the international literature. Intermaxillary immobilization was necessary in most cases.
Orbital Floor/Wall Fractures
Cantaloube et al.26 and Iizuka et al.27 have reported the use of PDS plates for reconstruction of orbital floor defects greater than 10 mm in size. The dimensions of the bowl-shaped plates were 28 28 1 mm. The plates were easy to cut to suitable sizes. They were placed over the defect subperiosteally. They were fixed to the orbital rim with one or two 0.35-mm steel wires or sutures. The PDS was well tolerated by the body and did not give rise to clinically detectable inflammatory reactions. New bone formation, even hypertrophic bone, was seen. The authors concluded that the material retained its structural integrity long enough for a sufficiently rigid scar to be formed,
117
preventing delayed herniation of the orbital contents. Because of the thickness of the plate, overcorrection is necessary. As the plate biodegrades, the globe attains a correct position.
Sasserath et al.65 reported the use of 0.15-mm-thick SR-PGA membranes in 20 blowout fractures. The sizes of the bony defects were not reported. The results were promising. Two patients complained of continuous infraorbital edema, which, according to the authors, was caused by poor residual drainage. The problem was solved by regular massage of the region.
Vert et al.66 reported the use of small (2-mm-thick) PLLA/ PGA composite plates reinforced with two-ply PGA fabric for repair of mandibular and skull fractures in 25 patients over a period of 2 years. The plates were warmed using an electric hair dryer and shaped to fit the bone. The plates were fixed using stainless steel screws. The first clinical results were good. There were no acute or chronic tissue reactions.
Zygoma
Gerlach67 used poly-L-lactide screws and plates in 15 patients with zygomatic fractures. The plates were 2 mm thick, 8 mm wide, and 26 mm long. The plates were fixed using four screws. The outer diameter of each screw was 2.7 mm and the core diameter 1.9 mm. The implants were sterilized using ethylene oxide. Stabilization was good, and no side effects were noted during a follow-up period of 20 months.
Bos et al.68 treated 10 patients with zygomatic fractures, using PLLA plates and screws. The plates had four holes, and they were slightly curved, 30 mm long, 6.7 mm wide, and 2 mm thick. The screws had a thread diameter of 2.7 mm. Shaping to the bone was achieved by heating the plate with a heat gun. Postoperative healing was good in all cases. However, after several years a foreign body reaction was observed in some patients.51
Mandible
Roed-Petersen4 treated two young patients (a 15-year-old girl and a 23-year-old man) with unfavorable, severely dislocated fractures of the angle using PGA (Dexon®) sutures. The need for intermaxillary fixation was evident, and it was undertaken for 6 weeks. Healing was uneventful. One year after operation, radiography showed that the bur holes at the sites of the sutures had filled in.
Niederdellmann and Bührmann5 used a PDS lag screw to fix a fracture of the mandibular angle. The screw core diameter was 3.2 mm and its thread diameter 4.5 mm. It is not stated whether or not MMF was applied.
Defect Repair and Membrane Technique
FIGURE 13.8 Mandibular condylar osteotomy fixed with SR-PLLA screw in sheep. The osteotomy line is barely visible (arrows). The screw is not radiopositive. Twelve weeks after the operation.
Biodegradable sheets and membranes can be used to prohibit faster-growing tissue (i.e., soft tissue) from intruding into bony defects, allowing the defect to be filled with new bone.
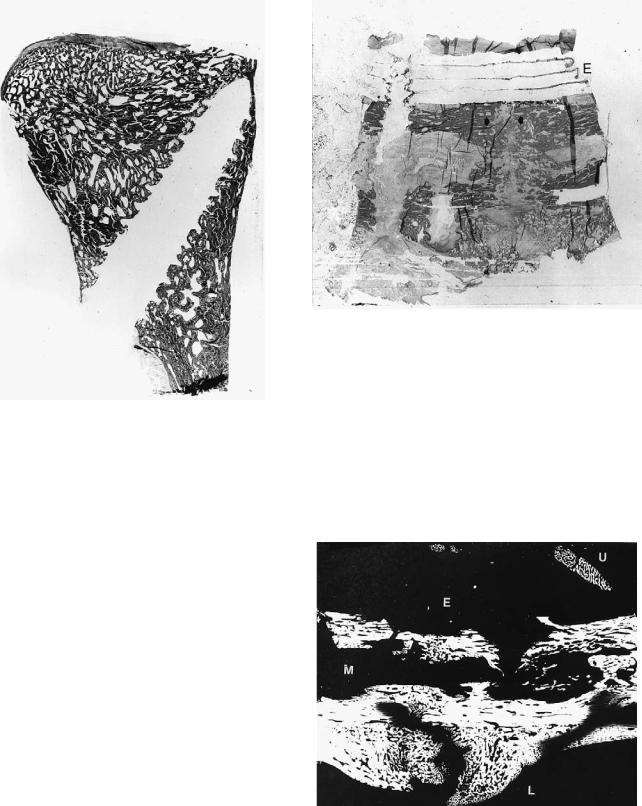
118 |
R. Suuronen and C. Lindqvist |
FIGURE 13.9 Histological section of mandibular condylar osteotomy 24 weeks postoperatively in sheep. The osteotomy cannot be detected. Degradation of the screw has not yet started.
For this purpose, PLA sheets have been used in the repair of oroantral fistulas.69 Also, PGA membranes have been used in implant surgery, when implants have been placed into fresh experimental sockets.70
PLA matrix has been successfully used as a wound stabilizing implant in reconstructive periodontal surgery. Supraalveolar circumferential periodontal defects were surgically created around mandibular premolars in beagle dogs. After 4 weeks of wound healing the use of PLA matrix significantly enhanced connective tissue repair. The authors conclude that the development of a biodegradable implant system aimed at stabilizing and supporting the healing wound seems a desirable direction for future research in regenerative periodontal procedures.
FIGURE 13.10 Histological section of a mandibular osteotomy fixed with SR-PLLA multilayer plate 6 weeks postoperatively. The 4-layer plate can be clearly seen (E). The osteotomy is still visible (arrows).
membranes prevent the ingrowth of soft tissue to bone defects, allowing new bone ingrowth. In implant surgery, the use of resorbable membrane simultaneously with the implant insertion immediately after extraction, other interventions, except for the abutment placement procedure, are avoided. These SR-PGA membranes have also been used in places where
Use of Materials Today
Guided Bone Growth
Both PLA/PGA (Vicryl®, Ethicon, Sollentuna, Sweden) and SR-PGA (Biofix®, Bioscience, Tampere, Finland) membranes (Figures 13.13 and 13.14) are currently used in periodontal reconstructive and oral surgery,71 as well as in orthognathic surgery (Le Fort I osteotomies). The biodegradable
FIGURE 13.11 Microradiograph of a mandibular osteotomy fixed with SR-PLLA multilayer plate 12 weeks postoperatively. A prominent callus can be seen on the lingual side (L) and inside the mandibular canal (M), as well as on top of the plate (U). The plate itself is not visible (E).
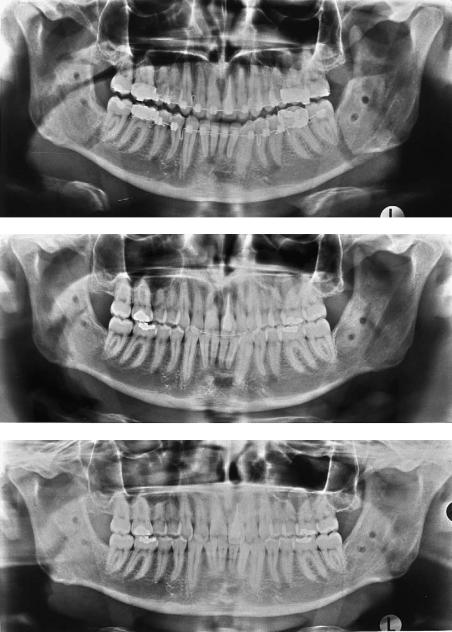
13. Bioresorbable Materials for Bone Fixation |
119 |
a
b
c
FIGURE 13.12 Sagittal osteotomy fixed with biodegradable SR-PLLA screws. (a) Postoperative ortopanthomogram; (b) 9 months postoperatively; (c) 15 months postoperatively.
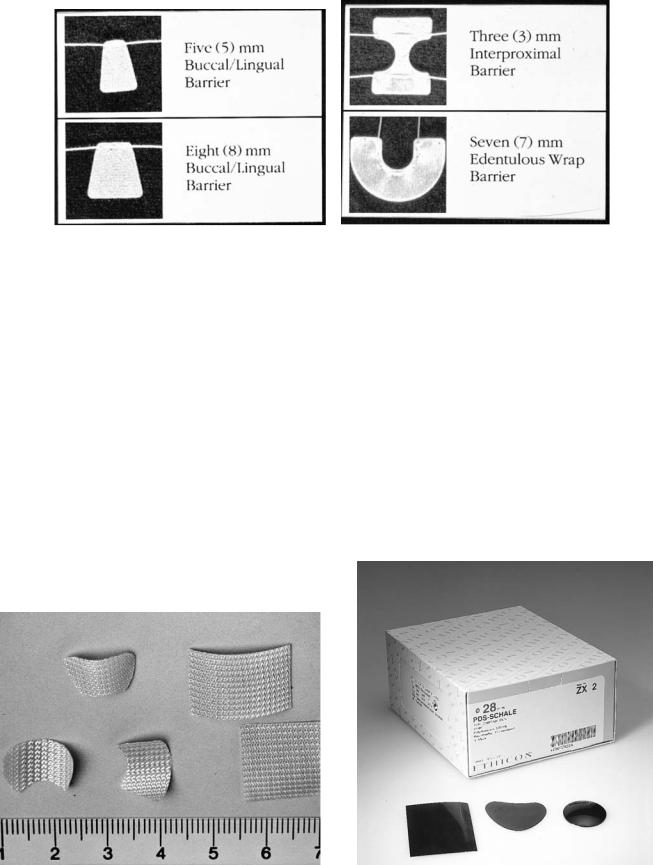
120 |
R. Suuronen and C. Lindqvist |
FIGURE 13.13 PLA/PGA membrane (Vicryl®).
bone is lost due to periodontitis, and in rescue procedures in infected bone resorption around implants.71
Fracture Fixation
The use of self-reinforced poly-L-lactide (SR-PLLA) implants in fracture fixation started in orthopedic surgery. It has been gradually extended to the field of oral and maxillofacial surgery. The implants used have to be strong enough to allow free movement of the jaws postoperatively. Only this can be considered the aim of research work, because the side effects caused by wiring the jaws together with intermaxillary fixation after open reduction of the fracture or osteotomy must be avoided whenever possible.
Orbital Floor/Wall Fractures
Today in clinical use are concave PDS plates and PGA sheets (Figures 13.14 and 13.15).27 The disadvantage of using these
plates is the thickness of the plate. When placed in the orbit, double vision is almost always a consequence for a couple of weeks. This situation is corrected when the plate degrades. Today, a thinner sheet is also available (PDS® Ethicon). In some patients the material has extruded through the skin when resorption starts. The reason for this phenomenon is still unknown.
SR-PGA membranes (Biofix®) have been used in blow-out fractures in a multicenter study in Europe. It was found that the membranesconformedwelltothetopographyoftheorbitalfloor and provided adequate initial support. The authors conclude that SR-PGA membrane appears to be a suitable alternative to traditional nonresorbable materials for orbital floor repair and may reduce the incidence of long-term complications associated with currently used alloplastic materials.65,72
FIGURE 13.14 PGA membrane (Biofix). |
FIGURE 13.15 PDS concave disc and sheet. |
13. Bioresorbable Materials for Bone Fixation
Sagittal Split Osteotomies
In our department, those sagittal osteotomies that do not have a bony gap between the buccal and lingual bone fragments are today fixed with SR-PLLA screws (Biofix®, Figure 13.12a–c). The screws are available in two sizes, with a core diameter of 2.7 or 2.0. With the longest follow-up in sagittal osteotomies now being more than 6 years, we believe that we probably can widen the range of use to other fractures and osteotomies of the facial skeleton, too.
Costochondral Arthroplasty
Biofix®-screws have been used to fix the rib transplant lateral to the mandibular ramus in costochondral arthroplasty (see Chapter 30).
Future Prospects
New Materials
New biodegradable materials for maxillofacial and orthopaedic surgery are constantly being studied. A copolymer of polylactide/poly-E-caprolactone seems to be a promising material for bone filling. Also an experimental polyorthoester (POE) has shown very good properties in tissue tolerance. However, these materials require extensive investigation before they can be used clinically.
New Applications: Bioactive Composites
Adding drugs to these biodegradable polymers has been studied for several years.73 However, these studies have dealt with controlled drug release only, without a simultaneous fracture fixation or tissue growth guidance. In the future, it might be possible to add, for example, antibiotics to these screws and membranes to inhibit bacterial ingrowth or growth factors to accelerate healing and bone ingrowth into the defect area.
References
1.Schmitt E, Polistina R. Polyglycolic acid prosthetic devices. US Patent 3 463 158: Aug. 26, 1969.
2.Cutright DE, Hunsuck E, Beasley JD. Fracture reduction using a biodegradable material, polylactic acid. J Oral Surg. 1971;29: 393–397.
3.Kulkarni RK, Moore EG, Hegyeli AF, Leonard F. Biodegradable poly(lactic acid) polymers. J Biomed Mater Res. 1971;5: 169–181.
4.Roed-Petersen B. Absorbable synthetic suture material for internal fixation of fractures of the mandible. Int J Oral Surg. 1974;3:133–136.
5.Niederdellmann H, Bührmann K. Resorbierbare Osteosyntheseschrauben aus Polydioxanon (PDS). Dtsch Z Mund Kiefer Gesichtschir 1983;7:399–400.
6.Cutright DE, Hunsuck E. The repair of fractures of the orbital floor using biodegradable polylactic acid. Oral Surg. 1972;33:28–34.
121
7.Suuronen R, Laine P, Pohjonen T, Lindqvist C. Sagittal ramus osteotomies fixed with biodegradable screws: A preliminary report. J Oral Maxillofac Surg. 1994;52:715–720.
8.Vert M, Christel P, Chabot F, Leray J. Bioresorbable plastic materials for bone surgery. In: Hastings GW, Ducheyne P, eds.
Macromolecular Biomaterials. Boca Raton, FL: CRC Press; 1984:119–142.
9.Handlos V. The hazards of ethylene oxide sterilization. Arch Pharm Chem Sci. 1979;7:147–157.
10.Handlos V. Kinetics of the aeration of ethylene oxide sterilized plastics. Biomaterials. 1980;1:149–157.
11.Gupta MC, Deshmukh VG. Radiation effects on poly(lactic acid). Polymer. 1983;24:827–830.
12.Gilding DK, Reed AM. Biodegradable polymers for use in surgery—polyglycolic/poly(lactic acid) homoand copolymers: 1. Polymer. 1979;20:1459–1465.
13.Lähde H, Pohjonen T, Heponen V-P, Vainionpää S, Rokkanen P, Törmälä P. In vitro degradation of poly-L-lactide fibres. In:
Abstract book of the IVth International Conference on Polymers in Medicine and Surgery. Leeuwenhorst, Holland; 1989:7/1–7/4.
14.Spenlehauer G, Vert M, Benoit JP, Boddaert A. In vivo and in vitro degradation of poly(D,L lactide/glycolide) type microspheres made by solvent evaporation method. Biomaterials. 1989;10:557–563.
15.Ray JA, Doddi N, Regula D, Williams JA, Melveger A. Polydioxanone (PDA), a novel monofilament synthetic absorbable suture. Surg Gynecol Obstet. 1981;153:497–507.
16.Craig PH, Williams JA, Davis KW, Magoun AD, Levy AJ, Bogdansky S, et al. A biologic comparison of polyglactin 910 and polyglycolic acid synthetic absorbable sutures. Surg Gynecol Obstet. 1975;141:1–10.
17.Ala-Kulju K, Verkkala K, Ketonen P, Heikkinen L, Harjola PT. Polydioxanone in coronary vascular surgery. J Cardiovasc Surg. 1989;30:754–756.
18.Vainionpää S, Rokkanen P, Törmälä P. Surgical applications of biodegradable polymers in human tissues. Prog Polym Sci. 1989;14:679–716.
19.Blatter G, Meier G. Augmentation der korakoklavikulären Bandnaht. Vergleich zwischen Drahtcerclage, Vicrylband und PDSKordel. Unfallchirurg. 1990;93:578–583.
20.Claes L, Burri C, Kiefer H, Mutschler W. Resorbierbare Implantate zur Refixierung von osteochondralen Fragmenten in Gelenkflächen. Akt Traumatol. 1986;16:74–77.
21.Gay B, Bucher H. Tierexperimentelle Untersuchungen zur Anwendung von absorbierbaren Osteosyntheseschrauben aus Polydioxanon (PDS). Unfallchirurg. 1985;88:126–133.
22.Greve H, Holste J. Refixation osteochondraler Fragmente durch resorbierbare Kunststoffstifte. Akt Traumatol. 1985;15:145–149.
23.Mäkelä EA. Healing of epiphyseal fracture fixed with a biodegradable polydioxanone implant or metallic pins. An experimental study on growing rabbits. Clin Mater. 1988;3:61–71.
24.Ochsner PE, Ilchmann TH. Zuggurtungsosteosynthesen mit resorbierbaren Kordeln bei proximalen Humerusmehrfragmentbrüchen. Unfallchirurg. 1991;94:508–510.
25.Plaga BR, Royster RM, Donigian AM, Wright GB, Caskey PM. Fixation of osteochondral fractures in rabbit knees. A comparison of Kirschner wires, fibrin sealant, and polydioxanone pins. J Bone Joint Surg. 1992;74-B:292–296.
26.Cantaloube D, Rives JM, Bauby F, Andreani JF, Dumas B. Utilisation de la cupule en PDS dans les fractures orbito-malares.
Rev Stomatol Chir Maxillofac. 1989;90:48–51.
122
27.Iizuka T, Mikkonen P, Paukku P, Lindqvist C. Reconstruction of orbital floor with polydioxanone plate. Int J Oral Maxillofac Surg. 1991;20:83–87.
28.Frazza EJ, Schmitt E. A new absorbable suture. J Biomed Mater Res Symp. 1971;1:43–58.
29.Hollinger JO, Battistone GC. Biodegradable bone repair materials. Syntheticpolymersandceramics.Clin Orthop. 1986;207:290–305.
30.Gerlach KL, Eitenmüller J. In vivo evaluation of 8 different polymers for use as osteosynthesis material in maxillo-facial surgery, In: Pizzoferrato A, Marchetti PG, Ravaglioni A, Lee ACJ, eds. Biomaterials and Clinical Applications. Amsterdam: Elsevier Science Publisher B.V., 1987:439–445.
31.Williams DF. Some observations on the role of cellular enzymes in the in-vivo degradation of polymers. In: Syrett BC, Acharya DA, eds. ASTM Special Technical Publications: Corrosion and Degradation of Implant Materials. Philadelphia: American Society for Testing and Materials; 1979:61–75.
32.Rokkanen P, Böstman O, Vainionpää S, Vihtonen K, Törmälä P, Laiho J, et al. Biodegradable implants in fracture fixation: Early results of treatment of fractures of the ankle. Lancet 1985; 1:1422–1424.
33.Hirvensalo E. Fracture fixation with biodegradable rods: fortyone cases of severe ankle fractures. Acta Orthop Scand. 1989; 60:601–606.
34.Partio EK, Böstman O, Hirvensalo E, Vainionpää S, Vihtonen K, Pätiälä H, et al. Self-reinforced absorbable screws in fixation of displaced ankle fractures: A prospective clinical study of 152 patients. J Orthop Trauma. 1992;6:209–215.
35.Böstman O, Hirvensalo E, Mäkinen J, Rokkanen P. Foreign body reactions to fracture fixation implants of biodegradable synthetic polymers. J Bone Joint Surg (Br). 1990;72-B: 592–596.
36.Holten CH. Lactic acid. In: Verlag Chemie. Weinheim; 1971: pp 221–231.
37.Suuronen R, Pohjonen T, Hietanen J. Long-term degradation of self-reinforced poly-L-lactide (SR-PLLA) plates in vivo and in vitro, in press.
38.Rozema FR, Bos RRM, Pennings AJ, Jansen HWB. Poly(L-lac- tide) implants in repair of defects of the orbital floor: An animal study. J Oral Maxillofac Surg. 1990;48:1305–1309.
39.Majola A, Vainionpää S, Mikkola HM, Törmälä P, Rokkanen
P.Absorbable self-reinforced polylactide (SR-PLA) composite rods for fracture fixation: Strength and strength retention in the bone and subcutaneous tissue of rabbits. J Mater Sci Mater Med. 1992;3:43–47.
40.Rozema FR. Resorbable poly(L-lactide) bone plates and screws. Tests and applications. PhD Thesis, University of Groningen, The Netherlands, 1991, 107 p.
41.Törmälä P, Rokkanen P, Laiho J, Tamminmäki M, Vainionpää
S.US Patent 4, 743, 257. May 10, 1988.
42.Törmälä P. Biodegradable self-reinforced composite materials; manufacturing structure and mechanical properties. Clin Mater. 1992;10:29–34.
43.Cutright DE, Hunsuck E. Tissue reaction to the biodegradable polylactic acid suture. Oral Surg. 1971;31:134–139.
44.Majola A, Vainionpää S, Vihtonen K, Mero M, Vasenius J, Törmälä P, et al. Absorption, biocompatibility and fixation properties of polylactic acid in bone tissue: an experimental study in rats. Clin Orthop. 1991;268:260–269.
45.Hatton PV, Walsh J, Brook IM. The response of cultured bone
R. Suuronen and C. Lindqvist
cells to bioresorbable polyglycolic acid and teflon reinforced silicone membranes for use in orbital floor fracture repair. Clin Mater. 1994;17:71–80.
46.Hoffmann R, Krettek C, Haas N, Tscherne H. Die distal radiusfraktur. Frakturstabilisierung mit biodegradablen Osteosyn- these-stiften (Biofix). Experimentelle Untersuchungen under erste klinische Erfahrungen. Unfallchirurg. 1989;92:430–434.
47.Böstman OM. Osteolytic changes accompanying degradation of absorbable fracture fixation implants. J Bone Joint Surg (Br). 1991;73-B:679–682.
48.Böstman OM. Intense granulomatous inflammatory lesions associated with absorbable internal fixation devices made of polyglycolide in ankle fractures. Clin Orthop. 1992;278:193– 199.
49.Santavirta S, Konttinen YT, Saito T, Grönblad M, Partio E, Kemppinen P, et al. Immune response to polyglycolic acid implants. J Bone Joint Surg (Br). 1990;72-B:597–600.
50.Eitenmüller J, David A, Muhr G. Treatment of ankle fractures with complete biodegradable plate and screws of high molecular weight polylactide. In: Proceedings of 92 Congrés Francais de Chirurgie. 1990; Paris.
51.Bergsma EJ, Rozema FR, Bos RRM, de Bruijn WC. Foreign body reactions to resorbable poly(L-lactide) bone plates and screws used for the fixation of unstable zygomatic fractures.
J Oral Maxillofac Surg. 1993;51:666–670.
52.Pihlajamäki H, Böstman O, Hirvensalo E, Rokkanen P, Törmälä P. Absorbable pins of self-reinforced poly-L-lactic acid for fixation of fractures and osteotomies. J Bone Joint Surg. 1992;74- B:853–857.
53.Rozema FR, Bos RRM, Pennings AJ, Jansen HWB. Poly(L-lac- tide) implants in repair of defects of the orbital floor: an animal study. J Oral Maxillofac Surg. 1990;48:1305–1309.
54.Getter L, Cutright DE, Bhaskar SN, Augsburg JK. A biodegradable intraosseus appliance in the treatment of mandibular fractures. J Oral Surg. 1972;30:344–348.
55.Gerlach KL, Krause HR, Eitenmüller J. Use of absorbable osteosynthesis material for mandibular fracture treatment of dogs. In: Pizzoferrato A, Marchetti PG, Ravagliori A, Lee ACJ, eds.
Biomaterials and Clinical Applications. Amsterdam: Elsevier Science Publisher B.V.; 1987:459–464.
56.Bos RRM. Poly(L-lactide) osteosynthesis. Development of bioresorbable bone plates and screws. Groningen, The Netherlands: University of Groningen; 1989. Thesis.
57.Törmälä P, Vainionpää S, Kilpikari J, Rokkanen P. The effects of fibre reinforcement and gold plating on the flexural strength of PGA/PLA copolymer materials in vitro. Biomaterials. 1987;8:42–45.
58.Suuronen R. Comparison of absorbable self-reinforced poly-L- lactide screws and metallic screws in the fixation of mandibular condyle osteotomies. Experimental study in sheep. J Oral Maxillofac Surg. 1991;49:989–995.
59.Suuronen R, Laine P, Sarkiala E, Pohjonen T, Lindqvist C. Sagittal split osteotomy fixed with biodegradable, self-rein- forced poly-L-lactide screws. Int J Oral Maxillofac Surg. 1992; 21:303–308.
60.Suuronen R, Pohjonen T, Vasenius J, Vainionpää S. Comparison of absorbable self-reinforced multilayer poly-L-lactide and metallic plates in the fixation of mandibular body osteotomies: An experimental study in sheep. J Oral Maxillofac Surg. 1992; 50:255–262.
13. Bioresorbable Materials for Bone Fixation
61.Suuronen R, Pohjonen T, Wessman L, Törmälä P, Vainionpää
S.New generation biodegradable plate for fracture fixation. Comparison of bending strengths of mandibular osteotomies fixed with absorbable self-reinforced multi-layer poly-L-lactide plates and metallic plates. An experimental study in sheep. Clin Mater. 1992;9:77–84.
62.Suuronen R. Biodegradable self-reinforced polylactide plates and screws in the fixation of osteotomies in the mandible. PhD Thesis. University of Helsinki, Finland, 1992.
63.Suuronen R, Törmälä P, Vasenius J, Wessman L, Mero M, Partio E, et al. Comparison of shear strength of osteotomies fixed with absorbable self-reinforced poly-L-lactide and metallic screws. J Mater Sci Mater Med. 1992;3:288–292.
64.Suuronen R, Manninen M, Pohjonen T, Laitinen O, Lindqvist
C.Mandibular osteotomy fixed with biodegradable plates and screws: an animal study. Br J Oral Maxillofac Surg. 1997;35:341–348.
65.Sasserath C, Van Reck J, Gitani J. Utilisation d’une membrane d’acide polyglycolique dans les reconstructions de plancher orbitaire et dans les pertes de substances osseuses de la sphère maxillo-faciale. Acta Stomatol Belg. 1991;88:5–11.
66.Vert M, Christel P, Chabot F, Leray J. Bioresorbable plastic materials for bone surgery. In: Hastings GW, Ducheyne P, eds. Ad-
123
vances in Biomaterials. Amsterdam: Elsevier Science Publishers; 1990;9:573–578.
67.Gerlach KL. Treatment of zygomatic fractures with biodegradable poly(L-lactide) plates and screws. Clinical implant materials. In: Heimke G, Soltész U, Lee ACJ, eds. Advances in Biomaterials. Amsterdam: Elsevier Science Publishers; 1990;9:573–578.
68.Bos RRM, Boering G, Rozema FR, Leenslag JW. Resorbable poly(L-lactide) plates and screws for the fixation of zygomatic fractures. J Oral Maxillofac Surg. 1987;45:751–753.
69.Gerlach KL. Absorbierbare Polymere in der Mundund Kieferchirurgie. Zahnärtzliche Mitteilungen. 1988;78:1020–1024.
70.Muhonen J, Suuronen R, Sarkiala E, Oikarinen VJ, Happonen RP. Effect of polyglycolic acid membrane on bone regeneration around titanium fixtures implanted in bone sockets. J Mater Sci Mater Med. 1994;5:40–42.
71.Pajarola GF, Sailer HF. SR-PGA membranes in dental surgery. In: Rokkanen P, Törmälä P, eds. Self-Reinforced Biodegradable Polymeric Composites in Surgery. Boca Raton: CRC Press, in press.
72.McVicar I, Hatton PV, Brook IM. Self-reinforced polyglycolic acid membrane: a bioresorbable material for orbital floor repair.
Br J Oral Maxillofac Surg. 1995;33:220–223.
73.Heller J. Biodegradable polymers in controlled drug delivery.
CRC Crit Rev Ther Drug Carrier Syst 1985;1:39–90.