
- •Preface
- •Acknowledgments
- •Contents
- •Contributors
- •1. Introduction
- •2. Evaluation of the Craniomaxillofacial Deformity Patient
- •3. Craniofacial Deformities: Review of Etiologies, Distribution, and Their Classification
- •4. Etiology of Skeletal Malocclusion
- •5. Etiology, Distribution, and Classification of Craniomaxillofacial Deformities: Traumatic Defects
- •6. Etiology, Distribution, and Classification of Craniomaxillofacial Deformities: Review of Nasal Deformities
- •7. Review of Benign Tumors of the Maxillofacial Region and Considerations for Bone Invasion
- •8. Oral Malignancies: Etiology, Distribution, and Basic Treatment Considerations
- •9. Craniomaxillofacial Bone Infections: Etiologies, Distributions, and Associated Defects
- •11. Craniomaxillofacial Bone Healing, Biomechanics, and Rigid Internal Fixation
- •12. Metal for Craniomaxillofacial Internal Fixation Implants and Its Physiological Implications
- •13. Bioresorbable Materials for Bone Fixation: Review of Biological Concepts and Mechanical Aspects
- •14. Advanced Bone Healing Concepts in Craniomaxillofacial Reconstructive and Corrective Bone Surgery
- •15. The ITI Dental Implant System
- •16. Localized Ridge Augmentation Using Guided Bone Regeneration in Deficient Implant Sites
- •17. The ITI Dental Implant System in Maxillofacial Applications
- •18. Maxillary Sinus Grafting and Osseointegration Surgery
- •19. Computerized Tomography and Its Use for Craniomaxillofacial Dental Implantology
- •20B. Atlas of Cases
- •21A. Prosthodontic Considerations in Dental Implant Restoration
- •21B. Overdenture Case Reports
- •22. AO/ASIF Mandibular Hardware
- •23. Aesthetic Considerations in Reconstructive and Corrective Craniomaxillofacial Bone Surgery
- •24. Considerations for Reconstruction of the Head and Neck Oncologic Patient
- •25. Autogenous Bone Grafts in Maxillofacial Reconstruction
- •26. Current Practice and Future Trends in Craniomaxillofacial Reconstructive and Corrective Microvascular Bone Surgery
- •27. Considerations in the Fixation of Bone Grafts for the Reconstruction of Mandibular Continuity Defects
- •28. Indications and Technical Considerations of Different Fibula Grafts
- •29. Soft Tissue Flaps for Coverage of Craniomaxillofacial Osseous Continuity Defects with or Without Bone Graft and Rigid Fixation
- •30. Mandibular Condyle Reconstruction with Free Costochondral Grafting
- •31. Microsurgical Reconstruction of Large Defects of the Maxilla, Midface, and Cranial Base
- •32. Condylar Prosthesis for the Replacement of the Mandibular Condyle
- •33. Problems Related to Mandibular Condylar Prosthesis
- •34. Reconstruction of Defects of the Mandibular Angle
- •35. Mandibular Body Reconstruction
- •36. Marginal Mandibulectomy
- •37. Reconstruction of Extensive Anterior Defects of the Mandible
- •38. Radiation Therapy and Considerations for Internal Fixation Devices
- •39. Management of Posttraumatic Osteomyelitis of the Mandible
- •40. Bilateral Maxillary Defects: THORP Plate Reconstruction with Removable Prosthesis
- •41. AO/ASIF Craniofacial Fixation System Hardware
- •43. Orbital Reconstruction
- •44. Nasal Reconstruction Using Bone Grafts and Rigid Internal Fixation
- •46. Orthognathic Examination
- •47. Considerations in Planning for Bimaxillary Surgery and the Implications of Rigid Internal Fixation
- •48. Reconstruction of Cleft Lip and Palate Osseous Defects and Deformities
- •49. Maxillary Osteotomies and Considerations for Rigid Internal Fixation
- •50. Mandibular Osteotomies and Considerations for Rigid Internal Fixation
- •51. Genioplasty Techniques and Considerations for Rigid Internal Fixation
- •52. Long-Term Stability of Maxillary and Mandibular Osteotomies with Rigid Internal Fixation
- •53. Le Fort II and Le Fort III Osteotomies for Midface Reconstruction and Considerations for Internal Fixation
- •54. Craniofacial Deformities: Introduction and Principles of Management
- •55. The Effects of Plate and Screw Fixation on the Growing Craniofacial Skeleton
- •56. Calvarial Bone Graft Harvesting Techniques: Considerations for Their Use with Rigid Fixation Techniques in the Craniomaxillofacial Region
- •57. Crouzon Syndrome: Basic Dysmorphology and Staging of Reconstruction
- •58. Hemifacial Microsomia
- •59. Orbital Hypertelorism: Surgical Management
- •60. Surgical Correction of the Apert Craniofacial Deformities
- •Index
11
Craniomaxillofacial Bone Healing, Biomechanics, and Rigid Internal Fixation
Frederick J. Kummer
The basic orthopedic principles of bone healing and fixation biomechanics are applicable to craniomaxillofacial reconstructive surgery. In general, however, the emphasis is less on providing mechanical stability to resist high levels of applied physiological forces than on establishing rigid immobilization both to obtain proper, stable anatomic configuration and to promote rapid healing.1 For each particular surgical application, there exists a variety of fixation techniques to achieve these goals. This chapter discusses fixation methods in general, as well as their biomechanical aspects as they influence bone healing, and relates these principles to several bone-specific clinical applications.
Principles of Bone Healing
The basic AO/ASIF principle of craniomaxillofacial surgery is rigid internal fixation achieved by functionally stable fixation of bone surfaces through the use of an appropriate device and its correct surgical application.2–5 At present, related to the hardware system in current use in the craniomaxillofacial region, the process of stress shielding seems to be of little or no concern. The frequently observed process of bone resorption in the management of fractures in the human skeleton is now more often believed to be the result of interference with the vascular supply than the mechanical influences attributed to stress shielding.
Currently there exists controversy over whether completely rigid fixation is the optimal condition for bone healing. Although gross motion between two or more bone fragments usually leads to nonunion and fibrocartilage tissue formation, a low level of displacement (micromotion) appears to aid healing by providing a mechanical signal that stimulates the biological repair process.6 The optimal frequency, waveform, and total number of cycles of this signal have yet to be determined.
Bone healing in the presence of a gap with minimal movement passes through several stages of repair with concurrent increases in mechanical strength: hematoma and inflamma-
tion, callus formation, replacement by woven bone, and finally remodeling into lamellar or trabecular bone (Figures 11.1 and 11.2). When there is direct bone apposition and compression of a rigidly fixed small gap, healing occurs more rapidly because the initial repair stages are minimized or eliminated. Perren has related this phenomenon to the concept of interfragmentary strain, where the local strain in the healing region (change in gap size divided by original gap size) influences the nature of the tissues formed.7
Healing also requires an adequate blood supply. In terms of operative technique, this means preserving the vascular supply of the bone and providing conditions for early revascularization (soft tissue preservation). Numerous studies have demonstrated a direct relationship between the quantity and quality of microvascular structures in the healing region and the rate of formation and mechanical properties of new bone.5
Fixation Methods and Devices
Wires, staples, pins, plates, and screws are the devices commonly used to achieve fixation. All are typically made of stainless steel (316L), titanium (or Ti-6A1-4V), or less commonly, cobalt-chromium alloy.8 A renewed interest in biodegradable polymers such as polylactic acid, first proposed for these applications more than 20 years ago,9 has recently led to their clinical use.10 Research also continues into the use of various glues and adhesives for bone fixation.11,12 The advantages and disadvantages of these materials and their relative strength, modulus (stiffness), corrosion resistance, and ease of imaging (MRI, CT) are discussed in a following chapter.
Wire
Wire fixation used as cerclage or a bone suture is less common in maxillofacial reconstruction. In either case, multiple wires are required to provide more rigid positional fixation. This necessitates achieving equal tension during tightening,
101
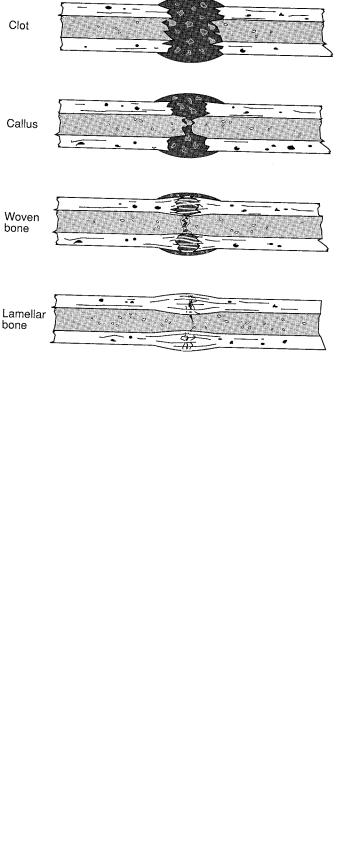
102 |
F.J. Kummer |
FIGURE 11.1 Stages of bone healing.
as loosening at one or more sites can provide a locus for motion and possible nonunion or malpositioning can result. Problems with wire fixation include the necessity and surgical complexity of making a hole in the bone and passing the wire through it, breakage during tightening or afterward due to fatigue (cyclic loading), and cut-through of the bone. In cerclage applications, there is some concern about compromise of the periosteal blood supply and resulting increased healing time required for revascularization.
Recent developments include wire tensioning/twisting instruments and the use of crimping systems to avoid the prob-
FIGURE 11.2 Mechanical behavior of healing bone.
lems with twisting or knot tying. Oriented polymers (e.g., Spectra) that do not stretch as do traditional suture materials can be used with a suture anchor system to eliminate the difficulty of looping a suture through bone. Wire fixation alone, however, does not provide functionally stable fixation.
Staples
Staples usually do not provide sufficient mechanical stability for permanent fixation, and their use often requires predrilling holes for the staple legs. Pneumatically driven staples can be used to rapidly tack fragments prior to a more rigid fixation, but insertion driving force must be carefully controlled to prevent untoward damage to the bone. Some staple designs can effect compression during insertion, such as prebent staple legs or fabrication from nitinol (an alloy that changes shape when heated to body temperature).
Pins
Kirschner wires, normally used to hold fragments prior to rigid fixation and for percutaneous pinning, in general lack sufficient mechanical stability for use as primary fixation. At least two should be used for each bone fragment, and they should not be inserted in a parallel manner to prevent “pistoning” of the fragment (Figure 11.3). Threaded pins provide additional stability because they minimize sliding of bone fragments; their removal, however, is more difficult. Occasionally, pinning is used in combination with a suture looped around the pin ends. This “tension band” technique provides significantly increased mechanical stability.
Screws
The major intrinsic factors that influence screw-holding power are the screw’s outer thread diameter, configuration, and length; the extrinsic factors are bone quality, bone type, and screw insertion orientation and driving torque.7,13 The two basic types of screw are cortical and cancellous, distinguished by thread design. Cancellous screws exhibit a greater distance between adjacent threads (pitch) and a higher ratio of outer thread diameter to body diameter (Figure 11.4). A screw’s inherent holding power is a function of outer thread diameter multiplied by the length of threads within the bone. When used to hold two bone fragments together, screws are commonly used in a lag modality in which the proximal portion of the screw remains free within one fragment; this is accomplished either by using a screw design that has no proximal threads or by enlarging the hole in the proximal fragment, preferably with a washer under the screw head for adequate support. Insertion torque determines the force with which bone fragments are held together and creates the friction that inhibits their motion. Control of torque by use of a torquelimiting screwdriver is important to prevent stripping of the bone and screw-head failure.
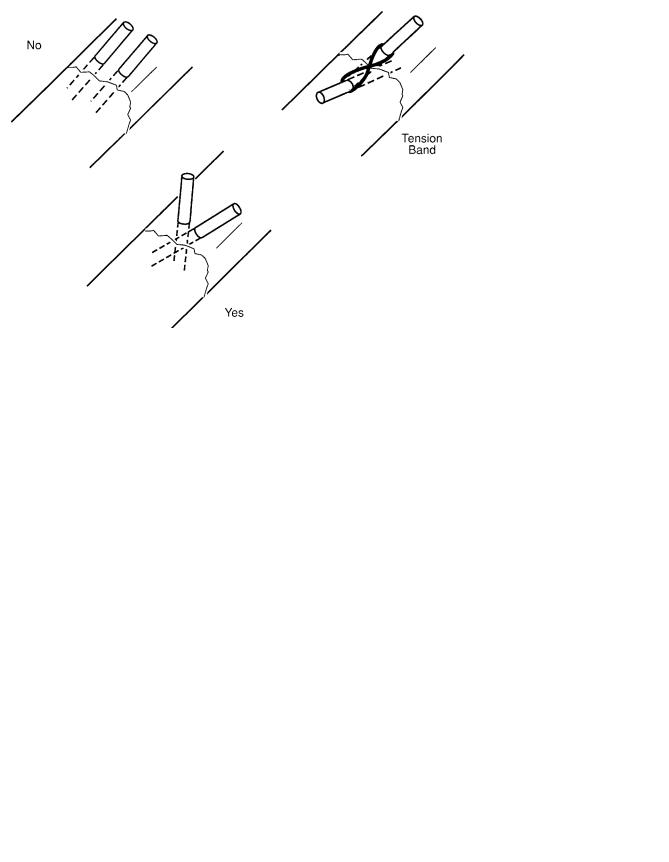
11. Craniomaxillofacial Bone Healing, Biomechanics, and Rigid Internal Fixation |
103 |
FIGURE 11.3 Pinning techniques for optimal fixation stability.
Because of anatomic constraints or surgical exposure, screws cannot always be inserted perpendicular to the bone axis, or the orientation of the ends of the bone fragments may not be perpendicular to the screw axis. In such cases, the screw’s holding power is decreased, and a shear component of the holding force is created that acts to destabilize alignment (Figure 11.5). With the size of screws usually used in craniomaxillofacial surgery, self-tapping screws are in the range of 1.0 to 2.4 mm and pretapping is unnecessary. However, for screws 2.7 mm or longer, pretapping is still recommended. Pretapping of screws is usually not necessary and has been shown to have minimal effect on their holding ability; many screws are self-tapping by virtue of a modified design of the leading threads. Usually, two or more screws are required for proper function, although one screw has been suggested for some applications if sufficient interfragment ap-
proximation can be achieved to create mechanical stability between the bone surfaces.14,15
Bone type and quality greatly influence screw-holding ability. Cortical bone is approximately 10 times stronger than cancellous bone.16 The thickness of the cortex and degree of osteopenia (bone density) are thus critical for fixation strength and dictate the number of screws required for adequate stability.17 Using screws in a biocortical manner appreciably increases the strength of fixation, although this is not possible for some maxillofacial reconstructions.18
FIGURE 11.4 Types of bone screws. |
FIGURE 11.5 Forces created by lag screw fixation. |
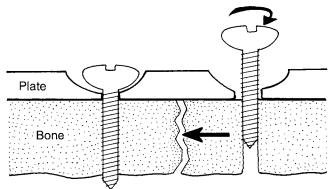
104
Plates
Because anatomic constraints limit the number of screws that can be applied in a given region, screws are often combined with plates to achieve adequate stability and increased strength of fixation. Plates for maxillofacial reconstruction are often four holed. Unlike their counterparts in orthopedic applications, they are usually applied to only one side of the bone. Because of anatomic constraints such as soft tissue thickness, the plates are relatively thin, just thick enough to possess stiffness—a function of width multiplied by the height squared—sufficient to prevent motion due to flexion.
Plate screws should be inserted with a torque driver and their tightness double-checked after insertion of all screws. Some plates incorporate a countersunk screw hole slot to accommodate the screw head; the slot is eccentrically situated so that interfragmentary compression is achieved as the screw is tightened (Figure 11.6). An alternative strategy is to prebend the plate so that when the screws are tightened, the bone fragments are approximated. Some plates have threaded holes to engage the screws; in these designs, bicortical screw insertion is not essential for maximum stability.
Plates can also be used to span gaps created by severe fractures or tumor surgery, frequently with the help of bone grafts.19 Unless the graft is an exact fit between the bone ends, the plate will bear the entire load across the defect. The bending moment on the plate, screw, and bone at the point of fixation linearly increases with defect size, requiring additional stabilization, particularly at the proximal end of the plate. Thus, as a general rule, at least three screws are needed at each end of the plate for this application. Using multiple-holed plates in this application allows one to select the best osseous sites for screw purchase and permits anchoring of the graft by additional screws.
Potential drawbacks to plate fixation are that it requires a larger exposure during surgery and that plate application may compromise periosteal blood supply. Some plate designs incorporate inferior feet or ridges to minimize the risk of the latter possibility. More flexible polymeric plates, currently in
FIGURE 11.6 Screw hole mechanism of the self-compressing plate.
F.J. Kummer
use, permit a greater degree of micromotion that may accelerate bone healing.
Surgical Applications
Among the several factors that must be weighed to determine the optimal fixation method for a specific application, two are fundamental. Mechanical considerations include the types (tension, bending, and/or torsion) and magnitude of forces to which the fixation will be subjected and whether these forces will be cyclic (e.g., chewing), in which case additional strength of fixation is required to compensate for possible fatigue of the supporting bone. Bone quality determines the strength available to support the fixation device. Other factors include surgical and anatomic considerations. For example, the exposure (possible scarring, vascular compromise), whether the device will fit adequately within the soft tissues, and whether neurovascular structures are at risk.
Evaluation of fixation strength can be accomplished by laboratory testing of implants in cadaver bone. One difficulty of such testing is to adequately simulate in the test model the complex forces to which the device or fixation technique would be subjected in vivo as well as the biological repair processes that would act to stabilize the fixation. Cadaver studies can also be used to determine those anatomic structures that would be at risk.
The alternative method of evaluating the efficacy of a particular fixation method is by means of a prospective study using clinical trials. In this case, proper study design must be observed, including specifying an appropriate number of patients, ensuring adequate follow-up, and selecting suitable techniques of data quantification so that the several parameters of interest can be statistically analyzed in a proper manner.
Mandibular Osteotomies
Osteotomies and fixation of the mandible represent the most highly mechanically loaded situation for maxillofacial reconstruction. Bending loads during mastication, created by the action of several muscles, are significant. By modeling these muscle forces and analyzing them mathematically, a resultant force (vector sum of separate forces) can be calculated and used for the design of experiments to test fixation stability in the laboratory.20 Similar mathematical analyses can be used to ascertain optimal location and type of osteotomy. The location, in turn, determines the magnitude and direction of force to be applied to the fixation, while the type determines the degree of approximation of bone surfaces acting to augment the stability achieved by the fixation device itself.
Mandibular osteotomies are fixed by a variety of techniques: wiring, single or multiple screws, and miniplates. Laboratory studies have demonstrated that screw orientation, size, and insertion technique are critical for stability.21 Three 2.7- mm-diameter screws along the superior border and two plates
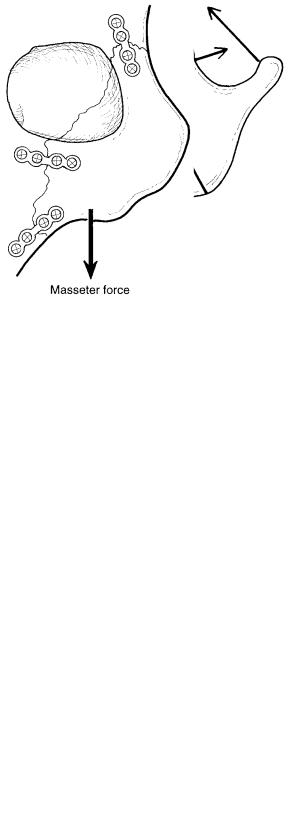
11. Craniomaxillofacial Bone Healing, Biomechanics, and Rigid Internal Fixation |
105 |
FIGURE 11.7 Optimal placement of screws for mandibular osteotomy showing muscle and reaction forces acting on the fixation (arrows not to scale).
have been shown to provide maximum fixation stability (Figure 11.7).
Orbitozygomatic Reconstruction
The principal aim of orbitozygomatic repair or reconstruction is to restore the anatomic configuration of the orbit. Loss of fixation stability can lead to nonunion or osseous displacement with severe sequelae (e.g., optic nerve damage).22,23 Biomechanically, several muscles, particularly the masseter, will act upon the fixation, resulting in a tendency toward inferior bone fragment displacement. The original type of fixation used transcutaneous Kirschner wires. Clinical failure us-
FIGURE 11.8 Optimal placements of plates for orbitozygomatic fracture fixation (adapted from Rohrich and Watumull25).
ing this method led to the use of two-point interosseous wiring and, later, threeand four-point wiring for increased stability. A more recent innovation has been the use of miniplates and screws for fixation (Figure 11.8). Laboratory cadaver studies comparing the stability achieved by these techniques demonstrated that the plating systems provide the most rigid fixation.24,25 The major problem with plate fixation is the larger surgical exposure required and greater profile (thickness) of the plate beneath the soft tissue.
References
1.Rudderman RH, Mullen RL. Biomechanics of the facial skeleton. Clin Plast Surg. 1992;19:11–29.
2.Gruss JS, Phillips JH. Complex facial trauma: the evolving role of rigid fixation and immediate bone graft reconstruction. Clin Plast Surg. 1989;16:93.
3.Hobar PC. Methods of rigid fixation. Clin Plast Surg. 1992; 19(1):31–39.
4.LaTrenta GS, McCarthy JG, Breitbard AS, et al. The role of rigid skeletal fixation in bone-graft augmentation of the craniofacial skeleton. Plast Reconstr Surg. 1989;84:578.
5.Phillips JH, Forrest CR, Gruss JS. Current concepts in the use of bone grafts in facial fractures. Basic science considerations. Clin Plast Surg. 1992;19(1):41–58.
6.Lin KY, Bartlett SP, Yaremchuk MJ, et al. An experimental study on the effect of rigid fixation on the developing craniofacial skeleton. Plast Reconstr Surg. 1991;87:229.
7.Perren SM, Cordey J, Baumgart F, Rahn BA, Schatzker J. Technical and biomechanical aspects of screws used for bone surgery.
Int J Orthop Trauma. 1992;2:31–48.
8.Lemons JE, Bidez MW. Endosteal implant biomaterials and biomechanics. In: McKinney RV Jr., ed. Endosteal Dental Implants. St. Louis, MO: C.V. Mosby; 1991.
9.Cutright DE, Hunsuck EE. The repair of fractures of the orbital floor using biodegradable polylactic acid. Oral Surg. 1972;33: 28–34.
10.Leenslag JW, Pennings AJ, Bos RRM, Rozema FR, Boering G. Resorbable materials of poly (L-lactide). VI. Plates and screws for internal fracture fixation. Biomaterials. 1987;8:70–73.
11.Sierra DH, Nissen AJ, Welch J. The use of fibrin glue in in intracranial procedures: preliminary results. Laryngoscope. 1990;100(4):360–363.
12.Weber SC, Chapman MW. Adhesives in orthopedic surgery. A review of the literature and in vitro bonding strengths of bonebonding agents. Clin Orthop. 1984;191:249–261.
13.Schatzker J, Sanderson R, Murnaghan JP. The holding power of orthopedic screws in vivo. Clin Orthop. 1975;108:115–126.
14.Foley WL, Frost DE, Paulin WB, et al. Internal screw fixation: comparison of placement pattern and rigidity. J Oral Maxillofac Surg. 1989;47:720.
15.Shetty V, Caputo A. Biomechanical validation of the solitary lag screw technique for reducing mandibular angle fractures.
J Oral Maxillofac Surg. 1992;50(6):603–607.
16.Bidez MW, Misch CE. Issues in bone mechanics related to oral implants. Implant Dent. 1992;1(4):289–294.
17.Misch CE. Density of bone: effect on treatment plans, surgical approach, healing, and progressive bone loading. Int J Oral Implantol. 1990;6:23–31.
106
18.Ellis JA Jr., Laskin DM. Analysis of seating and fracturing torque of bicortical screws. J Oral Maxillofac Surg. 1994;52(5): 483–486 (disc 487–488).
19.Raveh J, Sutter F. Titanium coated hollow screw reconstruction plate system for bridging lower jaw defects: biomechanical aspects. Int J Oral Maxillofac Surg. 1988;17:267–274.
20.van Eijden TMGJ. Three-dimensional analyses of human biteforce magnitude and moment. Arch Oral Biol. 1991;36(7): 535–539.
21.Foley WL, Beckman TW. In vitro comparison of screw versus plate fixation in the sagittal split osteotomy. Int J Adult Orthod Orthognath Surg. 1992;7(3):147–151.
F.J. Kummer
22.Jackson IT. Classification and treatment of orbitozygomatic and orbitoethmoid fractures—the place of bone grafting and plate fixation. Clin Plast Surg. 1989;16:77.
23.Rohrich RJ, Hollier LH, Watumull D. Optimizing the management of orbitozygomatic fractures. Clin Plast Surg. 1992; 19(1):149–165.
24.Davidson J, Nickerson D, Nickerson B. Zygomatic fractures: comparison of methods on internal fixation. Plast Reconstr Surg. 1990:25–32.
25.Rohrich RJ, Watumull D. The superiority of rigid plate fixation in the management of zygoma fractures and a long-term followup clinical study. Plast Reconstr Surg. 1991;18:58–61.