
- •Contents
- •Preface
- •Chapter 1 Introduction (K. Fujimoto)
- •Chapter 2 Small antennas (K. Fujimoto)
- •Chapter 3 Properties of small antennas (K. Fujimoto and Y. Kim)
- •Chapter 4 Fundamental limitation of small antennas (K. Fujimoto)
- •Chapter 5 Subjects related with small antennas (K. Fujimoto)
- •Chapter 6 Principles and techniques for making antennas small (H. Morishita and K. Fujimoto)
- •Chapter 7 Design and practice of small antennas I (K. Fujimoto)
- •Chapter 8 Design and practice of small antennas II (K. Fujimoto)
- •Chapter 9 Evaluation of small antenna performance (H. Morishita)
- •Chapter 10 Electromagnetic simulation (H. Morishita and Y. Kim)
- •Chapter 11 Glossary (K. Fujimoto and N. T. Hung)
- •Acknowledgements
- •1 Introduction
- •2 Small antennas
- •3 Properties of small antennas
- •3.1 Performance of small antennas
- •3.1.1 Input impedance
- •3.1.4 Gain
- •3.2 Importance of impedance matching in small antennas
- •3.3 Problems of environmental effect in small antennas
- •4 Fundamental limitations of small antennas
- •4.1 Fundamental limitations
- •4.2 Brief review of some typical work on small antennas
- •5 Subjects related with small antennas
- •5.1 Major subjects and topics
- •5.1.1 Investigation of fundamentals of small antennas
- •5.1.2 Realization of small antennas
- •5.2 Practical design problems
- •5.3 General topics
- •6 Principles and techniques for making antennas small
- •6.1 Principles for making antennas small
- •6.2 Techniques and methods for producing ESA
- •6.2.1 Lowering the antenna resonance frequency
- •6.2.1.1 SW structure
- •6.2.1.1.1 Periodic structures
- •6.2.1.1.3 Material loading on an antenna structure
- •6.2.2 Full use of volume/space circumscribing antenna
- •6.2.3 Arrangement of current distributions uniformly
- •6.2.4 Increase of radiation modes
- •6.2.4.2 Use of conjugate structure
- •6.2.4.3 Compose with different types of antennas
- •6.2.5 Applications of metamaterials to make antennas small
- •6.2.5.1 Application of SNG to small antennas
- •6.2.5.1.1 Matching in space
- •6.2.5.1.2 Matching at the load terminals
- •6.2.5.2 DNG applications
- •6.3 Techniques and methods to produce FSA
- •6.3.1 FSA composed by integration of components
- •6.3.2 FSA composed by integration of functions
- •6.3.3 FSA of composite structure
- •6.4 Techniques and methods for producing PCSA
- •6.4.2 PCSA employing a high impedance surface
- •6.5 Techniques and methods for making PSA
- •6.5.2 Simple PSA
- •6.6 Optimization techniques
- •6.6.1 Genetic algorithm
- •6.6.2 Particle swarm optimization
- •6.6.3 Topology optimization
- •6.6.4 Volumetric material optimization
- •6.6.5 Practice of optimization
- •6.6.5.1 Outline of particle swarm optimization
- •6.6.5.2 PSO application method and result
- •7 Design and practice of small antennas I
- •7.1 Design and practice
- •7.2 Design and practice of ESA
- •7.2.1 Lowering the resonance frequency
- •7.2.1.1 Use of slow wave structure
- •7.2.1.1.1 Periodic structure
- •7.2.1.1.1.1 Meander line antennas (MLA)
- •7.2.1.1.1.1.1 Dipole-type meander line antenna
- •7.2.1.1.1.1.2 Monopole-type meander line antenna
- •7.2.1.1.1.1.3 Folded-type meander line antenna
- •7.2.1.1.1.1.4 Meander line antenna mounted on a rectangular conducting box
- •7.2.1.1.1.1.5 Small meander line antennas of less than 0.1 wavelength [13]
- •7.2.1.1.1.1.6 MLAs of length L = 0.05 λ [13, 14]
- •7.2.1.1.1.2 Zigzag antennas
- •7.2.1.1.1.3 Normal mode helical antennas (NMHA)
- •7.2.1.1.1.4 Discussions on small NMHA and meander line antennas pertaining to the antenna performances
- •7.2.1.2 Extension of current path
- •7.2.2 Full use of volume/space
- •7.2.2.1.1 Meander line
- •7.2.2.1.4 Spiral antennas
- •7.2.2.1.4.1 Equiangular spiral antenna
- •7.2.2.1.4.2 Archimedean spiral antenna
- •7.2.2.1.4.3.2 Gain
- •7.2.2.1.4.4 Radiation patterns
- •7.2.2.1.4.5 Unidirectional pattern
- •7.2.2.1.4.6 Miniaturization of spiral antenna
- •7.2.2.1.4.6.1 Slot spiral antenna
- •7.2.2.1.4.6.2 Spiral antenna loaded with capacitance
- •7.2.2.1.4.6.3 Archimedean spiral antennas
- •7.2.2.1.4.6.4 Spiral antenna loaded with inductance
- •7.2.2.2 Three-dimensional (3D) structure
- •7.2.2.2.1 Koch trees
- •7.2.2.2.2 3D spiral antenna
- •7.2.2.2.3 Spherical helix
- •7.2.2.2.3.1 Folded semi-spherical monopole antennas
- •7.2.2.2.3.2 Spherical dipole antenna
- •7.2.2.2.3.3 Spherical wire antenna
- •7.2.2.2.3.4 Spherical magnetic (TE mode) dipoles
- •7.2.2.2.3.5 Hemispherical helical antenna
- •7.2.3 Uniform current distribution
- •7.2.3.1 Loading techniques
- •7.2.3.1.1 Monopole with top loading
- •7.2.3.1.2 Cross-T-wire top-loaded monopole with four open sleeves
- •7.2.3.1.3 Slot loaded with spiral
- •7.2.4 Increase of excitation mode
- •7.2.4.1.1 L-shaped quasi-self-complementary antenna
- •7.2.4.1.2 H-shaped quasi-self-complementary antenna
- •7.2.4.1.3 A half-circular disk quasi-self-complementary antenna
- •7.2.4.1.4 Sinuous spiral antenna
- •7.2.4.2 Conjugate structure
- •7.2.4.2.1 Electrically small complementary paired antenna
- •7.2.4.2.2 A combined electric-magnetic type antenna
- •7.2.4.3 Composite structure
- •7.2.4.3.1 Slot-monopole hybrid antenna
- •7.2.4.3.2 Spiral-slots loaded with inductive element
- •7.2.5 Applications of metamaterials
- •7.2.5.1 Applications of SNG (Single Negative) materials
- •7.2.5.1.1.2 Elliptical patch antenna
- •7.2.5.1.1.3 Small loop loaded with CLL
- •7.2.5.1.2 Epsilon-Negative Metamaterials (ENG MM)
- •7.2.5.2 Applications of DNG (Double Negative Materials)
- •7.2.5.2.1 Leaky wave antenna [116]
- •7.2.5.2.3 NRI (Negative Refractive Index) TL MM antennas
- •7.2.6 Active circuit applications to impedance matching
- •7.2.6.1 Antenna matching in transmitter/receiver
- •7.2.6.2 Monopole antenna
- •7.2.6.3 Loop and planar antenna
- •7.2.6.4 Microstrip antenna
- •8 Design and practice of small antennas II
- •8.1 FSA (Functionally Small Antennas)
- •8.1.1 Introduction
- •8.1.2 Integration technique
- •8.1.2.1 Enhancement/improvement of antenna performances
- •8.1.2.1.1 Bandwidth enhancement and multiband operation
- •8.1.2.1.1.1.1 E-shaped microstrip antenna
- •8.1.2.1.1.1.2 -shaped microstrip antenna
- •8.1.2.1.1.1.3 H-shaped microstrip antenna
- •8.1.2.1.1.1.4 S-shaped-slot patch antenna
- •8.1.2.1.1.2.1 Microstrip slot antennas
- •8.1.2.1.1.2.2.2 Rectangular patch with square slot
- •8.1.2.1.2.1.1 A printed λ/8 PIFA operating at penta-band
- •8.1.2.1.2.1.2 Bent-monopole penta-band antenna
- •8.1.2.1.2.1.3 Loop antenna with a U-shaped tuning element for hepta-band operation
- •8.1.2.1.2.1.4 Planar printed strip monopole for eight-band operation
- •8.1.2.1.2.2.2 Folded loop antenna
- •8.1.2.1.2.3.2 Monopole UWB antennas
- •8.1.2.1.2.3.2.1 Binomial-curved patch antenna
- •8.1.2.1.2.3.2.2 Spline-shaped antenna
- •8.1.2.1.2.3.3 UWB antennas with slot/slit embedded on the patch surface
- •8.1.2.1.2.3.3.1 A beveled square monopole patch with U-slot
- •8.1.2.1.2.3.3.2 Circular/Elliptical slot UWB antennas
- •8.1.2.1.2.3.3.3 A rectangular monopole patch with a notch and a strip
- •8.1.2.1.2.3.4.1 Pentagon-shape microstrip slot antenna
- •8.1.2.1.2.3.4.2 Sectorial loop antenna (SLA)
- •8.1.3 Integration of functions into antenna
- •8.2 Design and practice of PCSA (Physically Constrained Small Antennas)
- •8.2.2 Application of HIS (High Impedance Surface)
- •8.2.3 Applications of EBG (Electromagnetic Band Gap)
- •8.2.3.1 Miniaturization
- •8.2.3.2 Enhancement of gain
- •8.2.3.3 Enhancement of bandwidth
- •8.2.3.4 Reduction of mutual coupling
- •8.2.4 Application of DGS (Defected Ground Surface)
- •8.2.4.2 Multiband circular disk monopole patch antenna
- •8.2.5 Application of DBE (Degenerated Band Edge) structure
- •8.3 Design and practice of PSA (Physically Small Antennas)
- •8.3.1 Small antennas for radio watch/clock systems
- •8.3.2 Small antennas for RFID
- •8.3.2.1 Dipole and monopole types
- •8.3.2.3 Slot type antennas
- •8.3.2.4 Loop antenna
- •Appendix I
- •Appendix II
- •References
- •9 Evaluation of small antenna performance
- •9.1 General
- •9.2 Practical method of measurement
- •9.2.1 Measurement by using a coaxial cable
- •9.2.2 Method of measurement by using small oscillator
- •9.2.3 Method of measurement by using optical system
- •9.3 Practice of measurement
- •9.3.1 Input impedance and bandwidth
- •9.3.2 Radiation patterns and gain
- •10 Electromagnetic simulation
- •10.1 Concept of electromagnetic simulation
- •10.2 Typical electromagnetic simulators for small antennas
- •10.3 Example (balanced antennas for mobile handsets)
- •10.3.2 Antenna structure
- •10.3.3 Analytical results
- •10.3.4 Simulation for characteristics of a folded loop antenna in the vicinity of human head and hand
- •10.3.4.1 Structure of human head and hand
- •10.3.4.2 Analytical results
- •11 Glossary
- •11.1 Catalog of small antennas
- •11.2 List of small antennas
- •Index
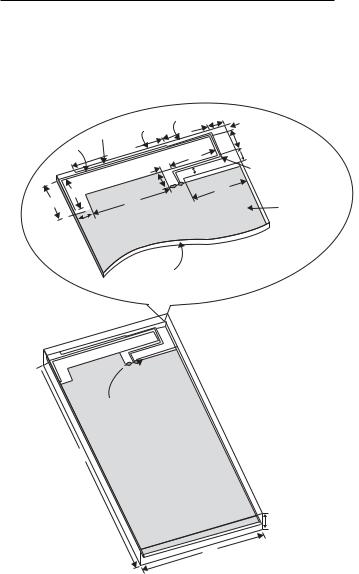
8.1 FSA (Functionally Small Antennas) |
299 |
|
|
Table 8.4 Measured peak and average gains and efficiency ([18], copyrightC 2010 IEEE)
Frequency (GHz) |
0.9 |
1.80 |
2.10 |
2.45 |
2.70 |
Peak Gain (dBi) |
1.12 |
3.13 |
2.26 |
1.82 |
3.21 |
Average Gain (dBi) |
−3.21 |
−4.87 |
−1.24 |
−0.83 |
−0.79 |
Efficiency (%) |
46.4 |
60.5 |
80.6 |
74.8 |
67.6 |
|
|
|
|
|
|
(a)
Gap2 = 1 0.5 |
|
1 |
||||
|
16 |
|||||
0.5 |
t= |
37 |
|
|
|
18 |
|
|
|
|
|||
C |
|
|
|
4 |
|
|
|
|
|
|
3 |
||
.5 |
|
= |
|
|
||
|
|
|
|
|||
=10 |
|
w |
5 |
|
|
A |
|
|
|
|
|||
d |
|
|
|
|
B |
|
15 |
|
24 |
|
|
|
|
|
|
|
|
|
||
D |
|
|
|
|
|
|
9 |
|
|
|
|
|
|
4 |
2 |
|
|
|
8 |
|
4 |
|
Gap1 = 0.5 |
21 |
|
Main ground on back side (60 × 100 mm2)
0.8-mm thick FR4 substrate as main circuit board
(b)B A
Feeding point
Shorting point, via to main ground
119
1-mm thick plastic housing
(εr = 3, σ = 0.02 S/m)
10
64
Figure 8.41 Geometry of the antenna in the mobile phone: (a) antenna structure and (b) antenna embedded on the ground plane ([19], copyright C 2010 IEEE).
8.1.2.1.2.1.4 Planar printed strip monopole for eight-band operation
A planar printed strip monopole with closely coupled parasitic shorted strips for eightband LTE/GSM/UMTS operation to be used in a mobile phone is described in [19]. Geometry of the antenna along with the dimensions are illustrated in Figure 8.41. The antenna has a simple structure comprised of a driven planar monopole (section A to C
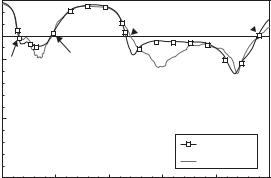
300 |
Design and practice of small antennas II |
|
|
Return loss (dB)
0
2910 1700 3:1 VSWR
970
10 665
20
Simulated
Measured
40 |
1000 |
1500 |
2000 |
2500 |
3000 |
500 |
Frequency (MHz)
Figure 8.42 Measured and simulated return loss ([19], copyright C 2010 IEEE).
in Figure 8.41) and a parasitic shorted strip (section B to D in Figure 8.41), both with comparable length and closely coupled to each other. An FR4 substrate with thickness of 0.8 mm and size of 115 × 60 mm is used as the systems circuit board. Point A of the driven strip monopole is the feeding point of the antenna, while point B of the parasitic strip is short-circuited to the top edge of the system ground plane through a via-hole in the system circuit board.
There are two gaps between the driven strip monopole and parasitic shorted strip: gap 1 of 0.5 mm in the front section and gap 2 of 1 mm in the remaining section of length t = 37 mm. These gaps provide capacitive coupling between the two strips, and adjustment of the coupling will make good impedance matching easy. The length of the driven strip monopole and parasitic shorted strips are both close to a quarter wavelength, although slightly different lengths. The two strips are designed to contribute to their lowest resonance modes, with one at frequencies lower than about 800 MHz and the other one at frequencies higher than about 800 MHz. Two resonance modes can be incorporated to produce a wide bandwidth to cover the frequency range of 698 to 960 MHz. The parasitic shorted strip can contribute to producing two higher resonance modes of about 1700 MHz and 2700 MHz and lead to wide upper band coverage of the frequency range from 1710 MHz to 2690 MHz by incorporating higher-order resonance modes of the driven strip monopole.
Measured and simulated return loss is given in Figure 8.42, which shows a wide bandwidth of 305 MHz (665–970 MHz), covering LTE 700/GSM 850/900 operations. The upper band shows a further wide bandwidth of 1210 MHz (1700–2910 MHz), which covers GSM 1800/1900/UMTS/LTE 2300/2500 operation.
The antenna exhibits gain of −0.4 to 1.1 dBi for the lower band (698–960 MHz) and 2.7 to 4.4 dBi for the higher band (1710–2690 MHz), and efficiency of 53%–76% and 52%–75% over the lower and higher bands, respectively.
Since the antenna is designed for installing on a mobile phone handset, SAR (Specific Absorption Rate) values pertaining to an operator’s head should be taken into
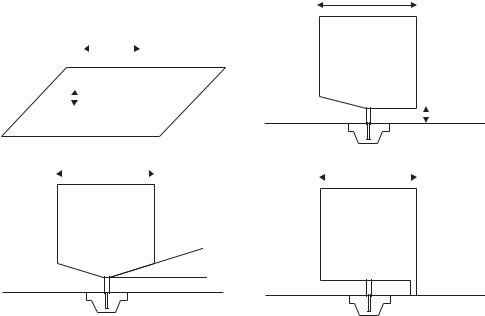
8.1 FSA (Functionally Small Antennas) |
301 |
|
|
L
|
|
|
|
|
|
|
|
|
|
|
|
|
|
|
|
L |
|
|
|
|
|
(a) |
|
|
|
|
|
|
(b) |
|||
|
h |
|
|
|
|
|
|
|
|
h |
|
|
|
|
|
|
|
|
|||
|
|
|
|
|
|
|
|
|
|
|
|
|
|
|
|
|
|
|
|
|
|
|
|
|
|
L |
|
|
|
L |
||
|
|
|
|
|
|
|
|
|||
|
|
|
|
|
|
|
|
|
|
|
(c) |
(d) |
α
Figure 8.43 Square planar monopole: (a) on the ground plane, (b) with asymmetric beveling,
(c) with symmetrical beveling, and (d) with a shorting post ([20], copyright C 2003 IEEE).
consideration as an important subject when the antenna is designed. The SAR values are calculated by using the simulator SEMCAD for a case where the proposed antenna is mounted at the bottom of the system circuit board. By using the input power of 24 dBm for the GSM 850/900 and 21 dBm for the GSM 1800/1900, UMTS, and LTE operations, the SAR values per gram of head tissue are obtained all well below the SAR value limit of 1.6 W/kg. This result indicates that the proposed antenna can be employed in mobile phone handsets without any concern over effect of radiation from the mobile phone.
8.1.2.1.2.2 Wideband antennas 8.1.2.1.2.2.1 Planar monopole antennas
A planar quarter-wavelength monopole is a simple wideband antenna, which is generally convenient to match to 50 with unbalanced feed, and does not require a balun. The planar monopole antenna was first described briefly in the literature in 1968 [20] and more detail was given in 1976 in [21], where wide impedance characteristics of a planar quarter-wave monopole were discussed. Subsequently studies on planar monopoles have continued, dealing with various types and shapes of antennas theoretically and experimentally. Among them, an example was that a disk-shaped planar monopole had shown return loss greater than 10 dB over an impedance bandwidth ratio in excess of 10 to 1 [22]. One of the simplest typical planar shapes is a square monopole vertically standing on the ground plane as shown in Figure 8.43, which depicts (a) the

302 |
Design and practice of small antennas II |
||||||||
|
|
|
|
|
|
|
|
|
|
|
|
|
|
|
|
|
|
|
|
|
|
|
|
|
|
|
|
|
|
|
|
|
|
|
|
|
|
|
|
a s l
h
w1 w2
wt
d
Figure 8.44 Geometry of a folded loop [27b].
basic geometry, (b) modified versions at its bottom side with asymmetrical beveling,
(c) with symmetrical beveling, and (d) with a shorting post. There have been various planar geometries such as rectangle, trapezoid, triangle, and so forth [23–25].
In practical applications, a conventional monopole structure, comprising an antenna standing vertically on the ground plane, is not preferable, because it usually requires an infinite or comparable ground plane, leading to increasing the antenna volume, contrary to the need to make the antenna small and compact. The recent trend is to use planar monopole structures, comprised of the antenna and the ground plane being placed in the same plane. By this means the antenna size can be reduced, granted that the size of ground plane is reduced. To maintain the wide bandwidth with reduced-size ground planes, various techniques have been developed. Antenna profiles can be all sorts of planar geometries; disk, rectangle, triangle, ellipse, and so forth. Some of them will be described later.
8.1.2.1.2.2.2 Folded loop antenna
The folded loop antenna was initially designed as an antenna having a balanced structure and fed by a balanced line to suppress the current flowing into the ground. This is important in portable devices in order to mitigate the body effect on the antenna performance, produced by the current on the ground plane, which is perturbed by the operator’s hand and head [26].
The antenna is fabricated by folding a long thin rectangular loop of total one wavelength to form a half-wavelength folded dipole, which appears as a folded two-wire transmission line as illustrated in Figure 8.44 [27a]. The antenna is effectively divided into two parts; a dipole structure and a two-wire transmission line. The dipole part essentially contributes to radiation while the two-wire transmission line plays a significant role for determining the antenna impedance [27b] (refer to Appendix I following this
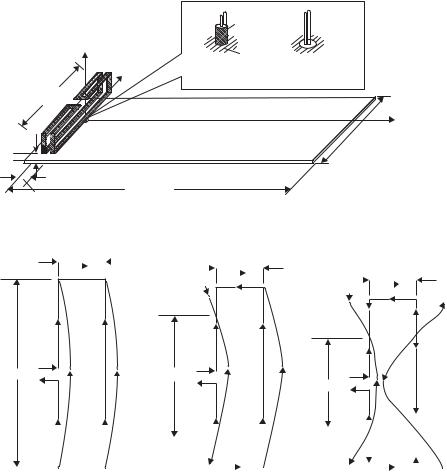
8.1 FSA (Functionally Small Antennas) |
303 |
|
|
|
coaxial |
two-wire |
|
|
cable |
line |
|
z |
|
|
|
y |
(b) unbalanced |
(a) balanced |
|
system |
system |
||
|
36.3
36.3
1
119.4 |
Unit [mm] |
2.1 |
|
Figure 8.45 Geometry of the folded loop placed on the ground plane [27b, 28].
d |
|
0 |
|
|
|
|
d |
|
0 |
|
|
|
|
|
|
||||
|
|
|
|
|
|||||
|
|
|
|
|
|
|
|
+ |
|
d |
|
0 |
|
|
+
λ1/2 |
+ |
+ |
λ2/2 |
+ |
+ |
λ3/2 + − |
|
|
|||||
|
|
|
|
|
|
|
|
|
|
|
|
|
|
|
+ |
|
|
|
|
|
|
|
+ |
|
|
|
|
|
|
|
|
|
|
|
|
|
|
|
|
|
|||
|
|
|
|
|
|
|
|
|
|
|
|
|
|
|
|
|||
|
|
|
|
|
|
|
|
|
|
|
|
|
|
|||||
|
|
|
|
|
|
|
|
|
|
|
|
|
||||||
|
|
|
|
|
|
|
|
|
|
|
||||||||
|
|
|
|
|
|
|
|
|
|
|
|
|
|
|
|
|
|
|
(a) f/f0 = 0.80 |
|
(b) f/f0 = 1.01 |
|
(c) f/f0 = 1.21 |
Figure 8.46 Current distributions for resonance on the folded loop element ([27b, 28], copyrightC 2001 IEICE).
section). The antenna is typically mounted on a ground plane in small equipment as shown in Figure 8.45, in which feeding structure is depicted in the inset, and dimensions are given as an example when the antenna is used for a typical mobile phone handset using finite ground plane [27c].
With this antenna structure, resonance occurs at several frequencies as several current modes are observed on the antenna elements, which are depicted in Figure 8.46. Resonances occur at (a) the first frequency f1 = 0.80 f0, (b) the second frequency f2 = 1.01 f0, and (c) the third frequency f3 = 1.21 f0, where f0 is the center frequency of the frequency band [27c]. It was found that a wideband performance was obtained as a result of combined excitation of three closely separated frequencies. Calculated and measured VSWR characteristics when the center frequency f0 is 3470 MHz are shown in Figure 8.47, which indicates a wide bandwidth covering from 0.8 to 1.2 f / f0 for VSWR
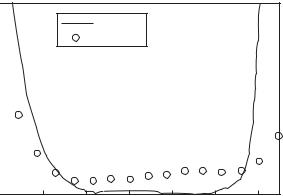
304 |
Design and practice of small antennas II |
|
|
|
10 |
|
|
|
|
|
|
|
9 |
|
|
|
|
|
|
|
8 |
|
|
|
|
|
|
VSWR |
7 |
|
|
|
|
||
6 |
|
|
|
|
|
|
|
|
5 |
|
|
|
|
|
|
|
4 |
|
|
|
|
|
|
|
3 |
|
|
|
|
|
|
|
2 |
|
|
|
|
|
|
|
1 |
|
|
|
|
|
|
|
0.65 |
Calculated
Measured
0.75 |
0.85 |
0.95 |
1.05 |
1.15 |
1.25 |
Relative frequency ( f/f0)
Figure 8.47 Calculated and measured VSWR (s = 20, h = 8, d = 1, w1 = w3 = 1, w2 = 4 [mm]) ([28], copyright C 2001 IEICE).
less than two. Current flows on the ground plane of a handset model are also calculated and shown to be very low as shown in Figure 8.48 [27, 28], illustrating almost all areas of the ground plane having about 30 dB lower values compared with that on the antenna element. Reduction of currents on the ground plane is also beneficial to mitigate the SAR (Specific Absorption Rate) value against the human head [29].
Having the balanced structure, another important feature is to bestow the antenna selfbalance property (refer to Appendix II following this section), with which a balun is not required and direct feeding with a coaxial line (unbalanced line) is allowable [27b, 30].
A folded loop can be modified to place sideward on the ground plane and further reduce the size in half as Figure 8.49 shows [31]. The figure illustrates a practical halfsize folded-loop antenna, hereafter referred to as a BFMA (Built-in Folded Monopole Antenna), being mounted on the ground plane of a mobile phone, along with dimensional parameters [31].
A PIFA and a BFMA, both resonating at the same frequency, are compared in terms of physical volume and bandwidth. It is assumed that a PIFA is mounted on the same size ground plane as a BFMA as Figure 8.50 shows, and the PIFA has the size (a = b = 19.5, d = 5, and h = 7 mm), by which the resonance frequency is the same as the center frequency 2250 MHz of the BFMA. Measured bandwidth for the PIFA and BFMA are 380 MHz and 340 MHZ, respectively, corresponding to 16.0% and 15.1% in the relative bandwidth with respect to the center frequency, and the physical volume of BFMA is estimated to be 42% of that of PIFA [31]. It can be said from this result that BFMA is smaller than PIFA, yet exhibits wider bandwidth.
8.1.2.1.2.3 Ultrawideband antennas
Ultrawideband (UWB) system is a recent hot topic in both industrial and academic fields. A UWB system is defined as a communication system that has either a bandwidth larger than 500 MHz or a relative bandwidth larger than 0.2 times the center frequency. The FCC allocated the frequency band between 3.1 GHz and 10.6 GHz for unlicensed systems
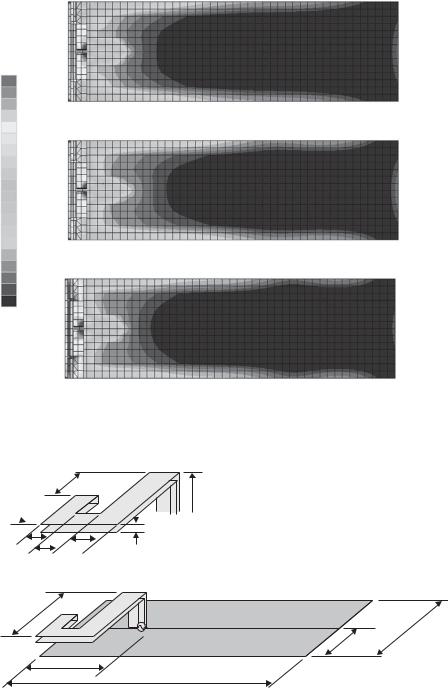
8.1 FSA (Functionally Small Antennas) |
305 |
|
|
0 dB |
|
−2 dB |
|
−4 dB |
|
−6 dB |
(a) f/f0 = 0.80 |
−8 dB |
|
−10 dB |
|
−12 dB |
|
−14 dB |
|
−16 dB |
|
−18 dB |
|
−20 dB |
|
−22 dB |
|
−24 dB |
|
−26 dB |
|
−28 dB |
|
−30 dB |
(b) f/f0 = 1.01 |
−32 dB |
|
−34 dB |
|
−36 dB |
|
−38 dB |
|
−40 dB |
|
(c) f/f0 = 1.21
Figure 8.48 Current distributions on the ground plane: (a) f/f0 = 0.80, (b) f/f0 = 1.01, and
(c) f/f0 = 1.21 ([27][28], copyright C 2001 and 2002 IEICE).
s
h
wt b
w1 |
w2 |
d |
|
18.85 |
|
|
36.3 |
|
17.4 |
15.0 |
Unit [mm] |
|
119.4 |
Figure 8.49 BFMA configuration and the antenna on the ground plane (w1 = w2 = 5, wt = 1.5, b = 0.5, h = 7, s = 12 [mm]) [27b].
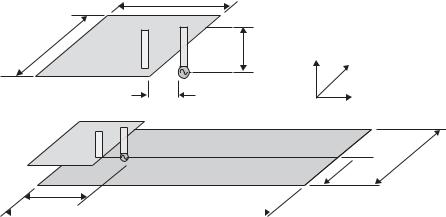
306 |
Design and practice of small antennas II |
|
|
|
a |
|
b |
h |
Z |
|
||
|
|
|
|
|
Y |
d |
|
X |
|
|
36.3 17.4
15.0 |
Unit (mm) |
119.4
Figure 8.50 PIFA for comparison (a = b = 19.5, d = 5, h = 7 [mm]) [27b].
operating with a power spectrum below –41.6 dBm. Since those systems operate with relatively low power, the communication link is limited to a short range and equipment is generally constructed in small size. Hence, antennas to be installed in such small equipment should accordingly be small. However, since the systems are designed for using signal transmissions that concern high-data-rate information, new challenges are imposed to develop small antennas yet having wideband.
Preferred antenna types to be used for UWB systems are planar ones with small dimensions, yet being simple, compact, low cost, and of course having appropriate performances that satisfy the system operation. In many cases electrically small antennas (ESA) with wideband features are required. Since conventional ESAs have generally narrow bandwidths, development of such small wideband antennas needs additional considerations beyond the techniques used to develop conventional ESAs. The simplest technique to produce wide bandwidth is full use of an area for the radiator, or generation of multiple resonances at closely spaced frequencies within a single antenna structure. For this purpose, a planar structure is fully used as a radiator, and current paths on the antenna surface are modified by modifying antenna shape and introducing slots/slits on the surface of the antenna. How to obtain a wide bandwidth with small-sized antennas is another serious problem. Reduction of the antenna size is attained not only by reduction of radiator size, but also reduction of the ground plane size. It should be noted that since the ground plane in small antennas often acts as a part of the radiator when the feeding system of the antenna is an unbalanced structure, and influences the radiation performance, special considerations to achieve required antenna performance are needed. An application of a DGS (Defected Ground Structure) concept can be one of the candidates. The DGS technique will be described in the next section, because it is a modified version of an EBG (Electromagnetic Band Gap) and exhibits bandgap properties and slow-wave effects that help to reduce the ground-plane size.
Planar structure is advantageous in applying antennas to very small equipment, as it can be easily embedded on a printed circuit board or integrated with other RF electronic
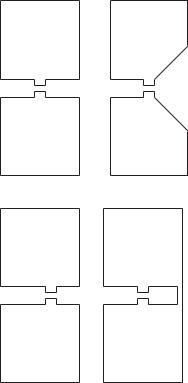
8.1 FSA (Functionally Small Antennas) |
307 |
|
|
Ant. A |
Ant. B |
Ant. C |
Ant. D |
Figure 8.51 Planar square dipole: (a) original dipole, (b) dipole with beveling at one-side,
(c) asymmetrically fed dipole, and (d) dipole with a shorting stub at one side [32].
devices. Also feed and matching structures play an important role for achieving wideband performance.
In UWB antennas, time response as well as frequency response usually is studied, as the UWB system was initially used for impulse communication systems. However, time response is not discussed here, because antennas described here are generally applied to ordinary wideband communication and other wireless wideband systems that are not concerned with such impulse systems.
There have been many papers treating UWB antennas. Various types of UWB antenna have been introduced; dipole types [32, 33], monopole types [34–42], shape modified antennas [35–38, 46, 47], antennas with reduced ground plane [39], slot/slit embedded antennas [40–45], and so forth.
The representative types of UWB antennas will be described here.
8.1.2.1.2.3.1 Dipole-type UWB antennas 8.1.2.1.2.3.1.1 Square patch dipoles
The simplest dipole antennas are illustrated in Figure 8.51, which shows A: a center-fed square dipole, B: a beveled center-fed square dipole, C: an offset-fed square dipole and D: a center-fed square dipole with a shorting pin [32].
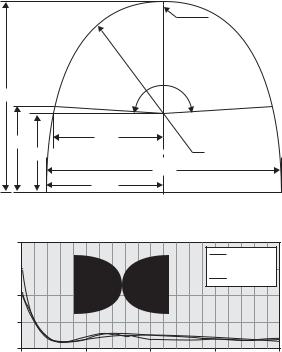
308 |
Design and practice of small antennas II |
|
|
(Gap = 0.08)
172.2°
6.80
3.07 |
3.99 |
|
2.80 R 4.00
8.40
4.20
(a)
VSWR
20
FE-BI 15 Measured
HFSS
10 |
|
|
|
|
5 |
|
|
|
|
0 |
0.4 |
0.6 |
0.8 |
1 |
0.2 |
Frequency (GHz)
(b)
Figure 8.52 Initial flare dipole (a) Geometry and dimensions (in inches) and (b) shaped dipole and frequency characteristics ([33], copyright C 2009 IEEE).
Impedance bandwidth for S11 < –10 dB of these antennas are: A: 3.60–8.21, B: 3.40– 10.17, C: 3.61–15.30, and D: 2.87–10.04 (in GHz). In terms of bandwidth, antenna C has widest, while antenna A has the narrowest. In addition, antenna C exhibits the most stable impedance response compared with other types. Antenna D introduces an additional resonance at about 2.7 GHz due to the shorting pin, which also provides inductive reactance below 2.7 GHz, while other types are capacitive.
8.1.2.1.2.3.1.2 A flare dipole with shape optimized using splines
A novel planar wideband antenna capable of operating from 190 MHz to 1000 MHz with gains greater than 0 dBi (having size λ/5 × λ/7 at 190 MHz) is introduced in [33a]. The initial flare dipole has the outer curve which draws a 172.2◦ arc with 4 inches radius, being connected to a trapezoid as is depicted in Figure 8.52(a), where dimensions in inches are given. Bandwidth characteristics of the initial flare dipole are also shown. The shaped dipole is depicted in Figure 8.52(b), which has dimensions of 13.68 inches long (including the gap) and 8.40 inches wide, corresponding to λ/3 × λ/5 at 280 MHz. Although the initial flare dipole has wide bandwidth of 3.5:1 (280 to 1000 MHz) as shown in Figure 8.52(b), further wide bandwidth of 5.25:1 (190 to 1000 MHz) is targeted and