
- •Contents
- •Preface
- •Chapter 1 Introduction (K. Fujimoto)
- •Chapter 2 Small antennas (K. Fujimoto)
- •Chapter 3 Properties of small antennas (K. Fujimoto and Y. Kim)
- •Chapter 4 Fundamental limitation of small antennas (K. Fujimoto)
- •Chapter 5 Subjects related with small antennas (K. Fujimoto)
- •Chapter 6 Principles and techniques for making antennas small (H. Morishita and K. Fujimoto)
- •Chapter 7 Design and practice of small antennas I (K. Fujimoto)
- •Chapter 8 Design and practice of small antennas II (K. Fujimoto)
- •Chapter 9 Evaluation of small antenna performance (H. Morishita)
- •Chapter 10 Electromagnetic simulation (H. Morishita and Y. Kim)
- •Chapter 11 Glossary (K. Fujimoto and N. T. Hung)
- •Acknowledgements
- •1 Introduction
- •2 Small antennas
- •3 Properties of small antennas
- •3.1 Performance of small antennas
- •3.1.1 Input impedance
- •3.1.4 Gain
- •3.2 Importance of impedance matching in small antennas
- •3.3 Problems of environmental effect in small antennas
- •4 Fundamental limitations of small antennas
- •4.1 Fundamental limitations
- •4.2 Brief review of some typical work on small antennas
- •5 Subjects related with small antennas
- •5.1 Major subjects and topics
- •5.1.1 Investigation of fundamentals of small antennas
- •5.1.2 Realization of small antennas
- •5.2 Practical design problems
- •5.3 General topics
- •6 Principles and techniques for making antennas small
- •6.1 Principles for making antennas small
- •6.2 Techniques and methods for producing ESA
- •6.2.1 Lowering the antenna resonance frequency
- •6.2.1.1 SW structure
- •6.2.1.1.1 Periodic structures
- •6.2.1.1.3 Material loading on an antenna structure
- •6.2.2 Full use of volume/space circumscribing antenna
- •6.2.3 Arrangement of current distributions uniformly
- •6.2.4 Increase of radiation modes
- •6.2.4.2 Use of conjugate structure
- •6.2.4.3 Compose with different types of antennas
- •6.2.5 Applications of metamaterials to make antennas small
- •6.2.5.1 Application of SNG to small antennas
- •6.2.5.1.1 Matching in space
- •6.2.5.1.2 Matching at the load terminals
- •6.2.5.2 DNG applications
- •6.3 Techniques and methods to produce FSA
- •6.3.1 FSA composed by integration of components
- •6.3.2 FSA composed by integration of functions
- •6.3.3 FSA of composite structure
- •6.4 Techniques and methods for producing PCSA
- •6.4.2 PCSA employing a high impedance surface
- •6.5 Techniques and methods for making PSA
- •6.5.2 Simple PSA
- •6.6 Optimization techniques
- •6.6.1 Genetic algorithm
- •6.6.2 Particle swarm optimization
- •6.6.3 Topology optimization
- •6.6.4 Volumetric material optimization
- •6.6.5 Practice of optimization
- •6.6.5.1 Outline of particle swarm optimization
- •6.6.5.2 PSO application method and result
- •7 Design and practice of small antennas I
- •7.1 Design and practice
- •7.2 Design and practice of ESA
- •7.2.1 Lowering the resonance frequency
- •7.2.1.1 Use of slow wave structure
- •7.2.1.1.1 Periodic structure
- •7.2.1.1.1.1 Meander line antennas (MLA)
- •7.2.1.1.1.1.1 Dipole-type meander line antenna
- •7.2.1.1.1.1.2 Monopole-type meander line antenna
- •7.2.1.1.1.1.3 Folded-type meander line antenna
- •7.2.1.1.1.1.4 Meander line antenna mounted on a rectangular conducting box
- •7.2.1.1.1.1.5 Small meander line antennas of less than 0.1 wavelength [13]
- •7.2.1.1.1.1.6 MLAs of length L = 0.05 λ [13, 14]
- •7.2.1.1.1.2 Zigzag antennas
- •7.2.1.1.1.3 Normal mode helical antennas (NMHA)
- •7.2.1.1.1.4 Discussions on small NMHA and meander line antennas pertaining to the antenna performances
- •7.2.1.2 Extension of current path
- •7.2.2 Full use of volume/space
- •7.2.2.1.1 Meander line
- •7.2.2.1.4 Spiral antennas
- •7.2.2.1.4.1 Equiangular spiral antenna
- •7.2.2.1.4.2 Archimedean spiral antenna
- •7.2.2.1.4.3.2 Gain
- •7.2.2.1.4.4 Radiation patterns
- •7.2.2.1.4.5 Unidirectional pattern
- •7.2.2.1.4.6 Miniaturization of spiral antenna
- •7.2.2.1.4.6.1 Slot spiral antenna
- •7.2.2.1.4.6.2 Spiral antenna loaded with capacitance
- •7.2.2.1.4.6.3 Archimedean spiral antennas
- •7.2.2.1.4.6.4 Spiral antenna loaded with inductance
- •7.2.2.2 Three-dimensional (3D) structure
- •7.2.2.2.1 Koch trees
- •7.2.2.2.2 3D spiral antenna
- •7.2.2.2.3 Spherical helix
- •7.2.2.2.3.1 Folded semi-spherical monopole antennas
- •7.2.2.2.3.2 Spherical dipole antenna
- •7.2.2.2.3.3 Spherical wire antenna
- •7.2.2.2.3.4 Spherical magnetic (TE mode) dipoles
- •7.2.2.2.3.5 Hemispherical helical antenna
- •7.2.3 Uniform current distribution
- •7.2.3.1 Loading techniques
- •7.2.3.1.1 Monopole with top loading
- •7.2.3.1.2 Cross-T-wire top-loaded monopole with four open sleeves
- •7.2.3.1.3 Slot loaded with spiral
- •7.2.4 Increase of excitation mode
- •7.2.4.1.1 L-shaped quasi-self-complementary antenna
- •7.2.4.1.2 H-shaped quasi-self-complementary antenna
- •7.2.4.1.3 A half-circular disk quasi-self-complementary antenna
- •7.2.4.1.4 Sinuous spiral antenna
- •7.2.4.2 Conjugate structure
- •7.2.4.2.1 Electrically small complementary paired antenna
- •7.2.4.2.2 A combined electric-magnetic type antenna
- •7.2.4.3 Composite structure
- •7.2.4.3.1 Slot-monopole hybrid antenna
- •7.2.4.3.2 Spiral-slots loaded with inductive element
- •7.2.5 Applications of metamaterials
- •7.2.5.1 Applications of SNG (Single Negative) materials
- •7.2.5.1.1.2 Elliptical patch antenna
- •7.2.5.1.1.3 Small loop loaded with CLL
- •7.2.5.1.2 Epsilon-Negative Metamaterials (ENG MM)
- •7.2.5.2 Applications of DNG (Double Negative Materials)
- •7.2.5.2.1 Leaky wave antenna [116]
- •7.2.5.2.3 NRI (Negative Refractive Index) TL MM antennas
- •7.2.6 Active circuit applications to impedance matching
- •7.2.6.1 Antenna matching in transmitter/receiver
- •7.2.6.2 Monopole antenna
- •7.2.6.3 Loop and planar antenna
- •7.2.6.4 Microstrip antenna
- •8 Design and practice of small antennas II
- •8.1 FSA (Functionally Small Antennas)
- •8.1.1 Introduction
- •8.1.2 Integration technique
- •8.1.2.1 Enhancement/improvement of antenna performances
- •8.1.2.1.1 Bandwidth enhancement and multiband operation
- •8.1.2.1.1.1.1 E-shaped microstrip antenna
- •8.1.2.1.1.1.2 -shaped microstrip antenna
- •8.1.2.1.1.1.3 H-shaped microstrip antenna
- •8.1.2.1.1.1.4 S-shaped-slot patch antenna
- •8.1.2.1.1.2.1 Microstrip slot antennas
- •8.1.2.1.1.2.2.2 Rectangular patch with square slot
- •8.1.2.1.2.1.1 A printed λ/8 PIFA operating at penta-band
- •8.1.2.1.2.1.2 Bent-monopole penta-band antenna
- •8.1.2.1.2.1.3 Loop antenna with a U-shaped tuning element for hepta-band operation
- •8.1.2.1.2.1.4 Planar printed strip monopole for eight-band operation
- •8.1.2.1.2.2.2 Folded loop antenna
- •8.1.2.1.2.3.2 Monopole UWB antennas
- •8.1.2.1.2.3.2.1 Binomial-curved patch antenna
- •8.1.2.1.2.3.2.2 Spline-shaped antenna
- •8.1.2.1.2.3.3 UWB antennas with slot/slit embedded on the patch surface
- •8.1.2.1.2.3.3.1 A beveled square monopole patch with U-slot
- •8.1.2.1.2.3.3.2 Circular/Elliptical slot UWB antennas
- •8.1.2.1.2.3.3.3 A rectangular monopole patch with a notch and a strip
- •8.1.2.1.2.3.4.1 Pentagon-shape microstrip slot antenna
- •8.1.2.1.2.3.4.2 Sectorial loop antenna (SLA)
- •8.1.3 Integration of functions into antenna
- •8.2 Design and practice of PCSA (Physically Constrained Small Antennas)
- •8.2.2 Application of HIS (High Impedance Surface)
- •8.2.3 Applications of EBG (Electromagnetic Band Gap)
- •8.2.3.1 Miniaturization
- •8.2.3.2 Enhancement of gain
- •8.2.3.3 Enhancement of bandwidth
- •8.2.3.4 Reduction of mutual coupling
- •8.2.4 Application of DGS (Defected Ground Surface)
- •8.2.4.2 Multiband circular disk monopole patch antenna
- •8.2.5 Application of DBE (Degenerated Band Edge) structure
- •8.3 Design and practice of PSA (Physically Small Antennas)
- •8.3.1 Small antennas for radio watch/clock systems
- •8.3.2 Small antennas for RFID
- •8.3.2.1 Dipole and monopole types
- •8.3.2.3 Slot type antennas
- •8.3.2.4 Loop antenna
- •Appendix I
- •Appendix II
- •References
- •9 Evaluation of small antenna performance
- •9.1 General
- •9.2 Practical method of measurement
- •9.2.1 Measurement by using a coaxial cable
- •9.2.2 Method of measurement by using small oscillator
- •9.2.3 Method of measurement by using optical system
- •9.3 Practice of measurement
- •9.3.1 Input impedance and bandwidth
- •9.3.2 Radiation patterns and gain
- •10 Electromagnetic simulation
- •10.1 Concept of electromagnetic simulation
- •10.2 Typical electromagnetic simulators for small antennas
- •10.3 Example (balanced antennas for mobile handsets)
- •10.3.2 Antenna structure
- •10.3.3 Analytical results
- •10.3.4 Simulation for characteristics of a folded loop antenna in the vicinity of human head and hand
- •10.3.4.1 Structure of human head and hand
- •10.3.4.2 Analytical results
- •11 Glossary
- •11.1 Catalog of small antennas
- •11.2 List of small antennas
- •Index

8Design and practice of small antennas II
8.1FSA (Functionally Small Antennas)
8.1.1Introduction
A Functionally Small Antenna (FSA) is an antenna system that has enhanced or improved performances without increasing the antenna dimensions. The FSA is constituted by
(1) integrating or combining either radiating or non-radiating components into an antenna system so as to improve or enhance the antenna performance, and (2) adding some function to an antenna so that the antenna will perform with newly added function. The Functionally Small Antenna system is not necessarily dimensionally small; however, it can be referred to equivalently as a small antenna, because enhanced performances or added functions compare to a larger antenna that could not be accomplished otherwise without enlarging the antenna dimensions.
Components to be integrated or combined into an antenna structure are electronic devices (either passive or active). There are many cases where antenna elements, regardless of either linear or planar, are combined with other antenna elements to constitute an integrated antenna. An antenna composed with integrated structure is referred to as an Integrated Antenna System (IAS). An IAS containing active components is referred to as an active IAS.
8.1.2Integration technique
There is a three-fold benefit of the integration techniques: (a) enhancement/improvement of antenna performances; (b) reduction of antenna dimensions; and (c) addition of some functions to an antenna system. The design target pertaining to (a) is enhancement/ improvement of bandwidth, including multiband as well as wideband performance, and of gain or efficiency. Factors in (b) lead to miniaturization, and those in (c) include addition of functions such as variable tuning facility, reconfigurable operation as well as amplification, frequency conversion, oscillation, and so forth, all integrated into an antenna system.
8.1.2.1Enhancement/improvement of antenna performances
When designing a small antenna, to keep the bandwidth at least the same or increase it without degradation in gain and efficiency is the prime factor to consider. The integration technique may be employed as one of the most useful approaches for this purpose.
8.1 FSA (Functionally Small Antennas) |
267 |
|
|
8.1.2.1.1 Bandwidth enhancement and multiband operation
Fundamentally, there are a few methods to accomplish wideband or multiband performance: (a) increase the radiation modes or number of resonance frequencies; (b) modify the antenna geometry; and (c) change substrate parameters used in the antenna structure. It is also important to design the matching circuitry for wideband operation. For this purpose, feeding structure, ground plane parameters, and application of metamaterials as well as networks consisting of several tuning circuits are considered. Radiation modes can be increased by combining two or more radiation sources that can be implemented by combining, for example, an electric source and a magnetic source, and by increasing the number of currents flowing on the antenna element, which interact with each other to increase the number of resonance frequencies, resulting in wider bandwidth. Wideband performance is also achieved by way of coupling between two resonators; for instance, a patch, which is a resonator by itself, may be coupled with another patch to function over a wider frequency band as a consequence of interaction between two closely separated resonant frequencies. A typical example of combining an electrical source with a magnetic source is a complementary antenna that is implemented by a monopole and a slot. Another example is an antenna of conjugate structure. An increase in the number of currents with different lengths of paths on the antenna element results in increase in the number of resonance frequencies; that produces wideband or multiband operation. For this purpose, an area of the radiator surface is expanded so that the current will flow over a wider area, taking different lengths of paths. A typical example is a bow-tie antenna. The coupling concept is realized by cutting slots or notches (or slits) on the planar antenna surface, by which the current flow will take circuitous routes around the slots or slits, resulting in the wideband or multiband operation. The term wideband is usually defined as the relative bandwidth of greater than 10% in terms of the –10 dB reflection coefficient or VSWR ≤ 2. Wideband antennas include antennas used in UWB systems, which operate in the frequency range from 3.1 GHz to 10.6 GHz.
There have been numerous papers introducing methods to accomplish wideband characteristics in small antennas. Wideband antennas are implemented practically by modification of antenna geometry, increasing area of planar antenna surface on which currents flow in different paths so that resonance occurs at different frequencies, creating wide bandwidth or producing multi-resonance. Various shaping of metallization such as circular, elliptical, rectangular, even irregular profiles have been explored. Arranging slots or slits (notches) on a planar antenna surface is another useful way to implement wideband or multiband antennas.
8.1.2.1.1.1 Modification of antenna geometry
The rectangular patch is an ordinal geometry of microstrip antennas. The shape can be modified to rectangle, triangle, circle, ellipse, and other forms such as L, E, and H shapes for obtaining wideband performance.
8.1.2.1.1.1.1 E-shaped microstrip antenna
For the purpose of bandwidth enhancement, two slots are incorporated in parallel into the antenna patch to form an E-shape, as shown in Figure 8.1, which illustrates (a) top view
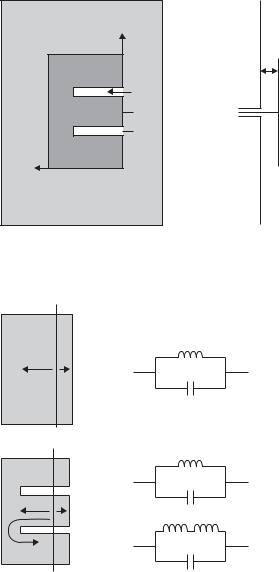
268 |
Design and practice of small antennas II |
|
|
(a) |
|
(b) |
|
|
X |
|
Patch |
h |
|
Ls |
|
|
Ws |
Slot |
L |
(Xf , Yf)· |
coax |
|
|
Ps |
|
|
Air |
Y |
W |
|
|
|
|
|
Ground plane |
Top view |
Side view |
Figure 8.1 Geometry of a wideband E-shaped patch antenna: (a) top view and (b) side view ([1], copyright C 2001 IEEE).
(a)
|
|
L |
|
J |
Patch |
C |
Equivalent circuit |
|
shape |
for patch |
L
(b) |
|
|
Equivalent circuit |
|
|
|
|
||
|
|
C |
for center part, |
|
J |
Patch |
|
high frequency |
|
L |
LS |
|||
|
shape |
|||
|
|
|
Equivalent circuit |
|
|
|
C |
for top and bottom parts, |
|
|
|
|
low frequency |
Figure 8.2 Principle of wideband operation: (a) an ordinary rectangular patch antenna, (b) an E-shaped patch antenna and two equivalent circuits, one for the center part and another for two side parts of the patch [1].
and (b) side view [1]. In the figure, antenna geometrical parameters are also provided; for the patch, the length L, the width W , and the height h, and regarding the slot, the length Ls and the width Ws, and the position Ps, distance from the center of the patch, and the feed position (Xf, Yf). The principle of the wideband operation is explained by using a model shown in Figure 8.2, in which (a) an ordinary rectangular patch and the equivalent LC circuit are shown for a comparison, along with (b) an E-shaped patch
8.1 FSA (Functionally Small Antennas) |
269 |
|
|
and the two equivalent LC circuits; one for the center part of the patch and another for the two side parts of the E-shaped patch. On the ordinary rectangular patch, current J flows from the feed point to the top and bottom edges of the patch and these current path lengths determine the inductance L and the capacitance C, as Figure 8.2(a) shows. On the other hand, a current on the E-shaped patch flows on the center part of the E-shape like an ordinary patch and other current flows into side parts of the E-shape taking the roundabout path, as Figure 8.2(b) shows. The center part of the E-shape patch can be modeled by a simple LC resonant circuit like the ordinary patch circuit, while the side part of the E-shape patch is modeled by a circuit with an additional series inductance Ls to L as shown in Figure 8.2(b). Hence, the equivalent circuit of the side part resonates at lower frequency than that of the center part. This means that a model of an E-shaped patch antenna changes from a single-resonance circuit to a dual-resonance circuit. Thus, coupling together of these circuits leads to wideband performance.
Essential parameters in the design of an E-shaped patch antenna are slot length Ls, slot position Ps, and slot width Ws. The slot length Ls is the important factor to characterize the resonance frequency; when the Ls is too short, the antenna has only one resonance frequency. As Ls increases, another lower resonance frequency appears. The longer Ls becomes, the lower the second resonance frequency will be. Ps is a parameter that can adjust matching conditions properly. When Ps becomes somewhat larger, the two resonance frequencies become distinct and a wideband match is obtained. However, as Ps further increases, the S11 between two resonance frequencies is larger than –10 dB. Then the antenna does not perform as a wideband antenna, but as a dual-band antenna. The third parameter Ws is a useful parameter to adjust coupling and achieve good matching.
An E-shaped patch antenna was developed for use in wireless communications operating at 1.9–2.4 GHz [1]. The antenna geometrical parameters are L = 70, W = 50, h = 15, Xf = 35, Yf = 6, Ls = 40, Ws = 6, and Ps = 10, where dimensions are all in mm. Calculated and measured S11 are shown in Figure 8.3, in which S11 of two other ordinary patch antennas (without slots) are provided for comparison. They have the same height and width as the E-shaped patch antenna, but the narrow patch antenna is one having the same length as the middle part of the E-shaped patch, and has a bandwidth about only 10%, while the wide patch is one with the same length as the E-shaped patch antenna and does not match well to 50 . On the contrary, the E-shaped patch antenna has shown resonance at two frequencies, 1.9 GHz and 2.4 GHz, and the bandwidth is 30.3%.
It should be noted that the ground plane (GP) often becomes a critical factor when a platform to mount an antenna is small. In this example [1], the size of GP is chosen to be 14 × 21 cm, which is about eight times that of the patch size. By observing the current distributions on the GP obtained by calculation, most currents flow under the patch, but a larger area is required for lower frequencies than higher frequencies. It was concluded that a 1λ (1.9 GHz) square plate is sufficient for proper operation of the antenna.
Directivity of the antenna is calculated to be 8.5 dB at 2.4 GHz and 6.7 dB at 1.9 GHz. The measured radiation patterns agree well with the calculated results. In the E-plane, the 3-dB beamwidth is 42◦ at 2.4 GHz and 63◦ at 1.9 GHz. The peak cross polarization at 2.4 GHz is –25 dB, and is lower than –15 dB at 1.9 GHz. In the H-plane, the radiation patterns are similar to those of the E-plane, and the 3-dB beamwidth is 60◦ at both
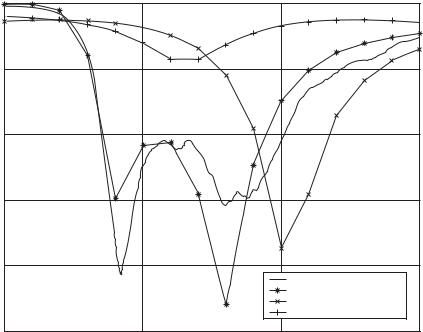
270 |
Design and practice of small antennas II |
|
|
0
–5
–10
S11 (dB)
–15
–20
E–shaped, measured E–shaped, calculated Narrow patch, calculated Wide patch, calculated
–25 |
2 |
2.5 |
3 |
1.5 |
Frequency (GHz)
Figure 8.3 S11 of the E-shaped patch antenna, compared with simple patch without slots ([1], copyright C 2001 IEEE).
frequencies. The peak cross polarization is –7 dB at 50◦, a rather high level that is due to the leaky radiation of the slots.
Another example shown in [1] is a wider band that was achieved by adjusting the parameters of the slots and the feeding point of an E-shaped patch antenna. The antenna has two distinct resonant frequencies at 2.12 GHz and 2.66 GHz and the frequency band ranges from 2.05–2.64 GHz, resulting in bandwidth as wide as 32.3%.
Another example of an E-shaped patch antenna introduced in [2] is designed to operate in the 800 MHz band and has wide bandwidth of 164 MHz. In order to obtain wider bandwidth, an LC circuit comprised of a small spiral, which produces an inductance, and a circular plate, which introduces a capacitance to compensate the inductance of the spiral and the feed line, thus producing a new resonance, is integrated into the feed circuit. This resonance frequency is very close to that of the E-shaped patch and thus wider bandwidth can be achieved.
An E-shaped patch antenna radiates a linearly polarized wave when currents on both side parts of the E-shaped surface are the same; however, when they are different as shown in Figure 8.4 [3], elliptically or circularly polarized (CP) waves are radiated. As can be observed in the figure, when the slot length varies, currents flowing on both sides of the E-shaped surface become unbalanced. When by this means, the x-directed current is made equal in magnitude to the y-directed current with a 90-degree phase difference,
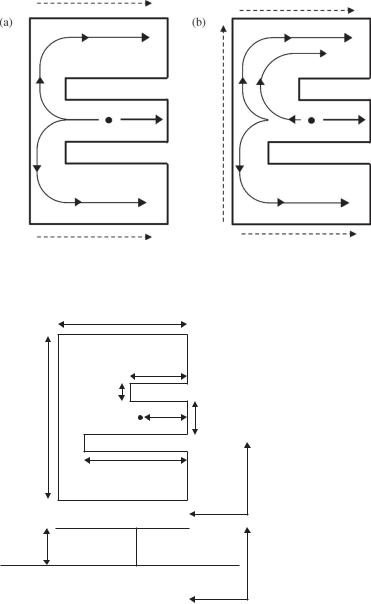
8.1 FSA (Functionally Small Antennas) |
271 |
|
|
Figure 8.4 Current flow across the E-shaped patch: (a) symmetrical flow and (b) asymmetrical flow ([2, 3], copyright C 2010 IEEE).
(a) |
L |
|
|
|
Ls1 |
|
Ws |
W |
F |
P |
|
|
x |
|
Ls2 |
|
y |
(b) |
z |
|
|
h |
|
|
y |
Figure 8.5 Geometry of a patch antenna with slots of different length: (a) top view and (b) side view ([2, 3], copyright C 2010 IEEE).
an E-shaped antenna radiates a CP wave. The parameters to be considered in the design of a CP antenna are: each length of its two slots, Ls1 and Ls2; the slot width Ws; the separation P between two slots and the location of the feed point F with a given patch size; length L; and width W . The antenna geometry is shown in Figure 8.5, (a) top and
(b) side views.

272Design and practice of small antennas II
Table 8.1 Dimensions of circularly polarized E-shaped patch
[mm]([3], copyright C 2010 IEEE)
εr |
h |
W |
L |
Ws |
Ls1 |
Ls2 |
P |
F |
1 |
10 |
77 |
47.5 |
7 |
19 |
44.5 |
14 |
17 |
2.2 |
6.7 |
63 |
33.5 |
4 |
27 |
6 |
20 |
10 |
|
|
|
|
|
|
|
|
|
The design procedure starts with an E-shape having the same length Ls1 and Ls2, then shortens either Ls1 or Ls2 to obtain circular polarization. Next change the probe position F to have improved AR (axial ratio) and S11, and align Ls2 or Ls1 for fine enhancement of AR as well as alignment with the S11 band. Finally, the design procedure ends when desired CP is obtained, otherwise, iterate back to the second step and repeat the steps again to satisfy the requirements. In the second step, make Ls1 shorter with Ls2 fixed, if left-handed (LH) CP is required, whereas if right-handed (RH) CP is desired, follow the opposite procedure. When making Ls1 shorter, the resonance frequency becomes higher and at the same time AR decreases from larger values showing linear polarization to smaller values to show CP, as the Ls1 changes electrical path length of the x-directed current. In this process, there is a state where Ls1 takes optimum values for the minimum AR as the phase difference between orthogonal currents approaches 90 degrees. Beyond this critical value, the phase difference between the orthogonal currents departs from 90 degrees. The design goal is to achieve desired AR bandwidth, which is defined as the frequency range across the 3-dB AR level, and to obtain required impedance and AR bandwidth together.
A CP E-shaped patch antenna to cover the IEEE 802.11b/g band (2.4–2.5 GHz) is proposed and antenna performances are introduced [3]. Dimensions of the antenna are provided in Table 8.1 (in mm). The metallic patch is fabricated via milling a thin copperclad substrate, which has εr = 2.2 and thickness of 0.787 mm. The patch is mounted above a ground plane of 200 × 95 mm by using a via of 0.75 mm radius, which is connected to the center pin of the 50connector. Simulated and measured S11 are shown in Figure 8.6. The measured impedance bandwidth is 9.27% (2.34–2.57 GHz) while simulated bandwidth is 10.27% (2.31–2.57 GHz). AR obtained by simulation and measurement is given in Figure 8.7, by which AR bandwidth is observed to be 8.1% (2.28–2.45 GHz) in simulation, while 16% (2.3–2.7 GHz) in measurement. Bandwidths for S11 and AR overlap in the range 2.34–2.57 GHz (9.27%). The maximum measured gain is 8.3 dBi with 15.5% 3-dB bandwidth. This can be observed in Figure 8.8, which depicts gain with respect to frequency. The bandwidth common to all of AR, gain, and S11 is 9.27%.
The E-shaped patch antenna may have great potential to be applied in various purposes such as reconfigurable antenna, diversity antenna, etc. For example, implementation of a reconfigurable antenna becomes feasible by inserting RF switches in appropriate locations across each slot and turning them on or off to make the two slot lengths unequal, thereby producing CP radiation as a consequence.
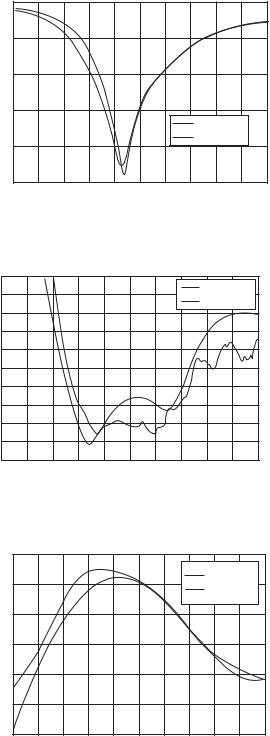
S11 (dB)
s-parameters 0
–5
–10
–15
Measured
HFSS
–20
–25 2 2.1 2.2 2.3 2.4 2.5 2.6 2.7 2.8 2.9 3
F(GHz)
Figure 8.6 Simulated and measured S11 of the CP E-shaped patch antenna ([3], copyright C 2010 IEEE).
|
10 |
Measured |
|
9 |
|
|
HFSS |
|
|
8 |
|
|
|
|
(dB) |
7 |
|
6 |
|
|
ratio |
|
|
5 |
|
|
Axial |
|
|
3 |
|
|
|
4 |
|
2
1
0 2 2.1 2.2 2.3 2.4 2.5 2.6 2.7 2.8 2.9 3
F(GHz)
Figure 8.7 Simulated and measured AR of the CP E-shaped patch antenna ([3], copyright C 2010 IEEE).
|
10 |
|
|
7.5 |
Measured |
|
HFSS |
|
(dB) |
|
|
5 |
|
|
gain |
2.5 |
|
Circular |
|
|
0 |
|
|
|
|
|
|
–2.5 |
|
–5 2 2.1 2.2 2.3 2.4 2.5 2.6 2.7 2.8 2.9 3
F(GHZ)
Figure 8.8 Simulated and measured gain of the E-shaped patch antenna ([3], copyright C 2010 IEEE).