
- •Contents
- •Preface
- •Chapter 1 Introduction (K. Fujimoto)
- •Chapter 2 Small antennas (K. Fujimoto)
- •Chapter 3 Properties of small antennas (K. Fujimoto and Y. Kim)
- •Chapter 4 Fundamental limitation of small antennas (K. Fujimoto)
- •Chapter 5 Subjects related with small antennas (K. Fujimoto)
- •Chapter 6 Principles and techniques for making antennas small (H. Morishita and K. Fujimoto)
- •Chapter 7 Design and practice of small antennas I (K. Fujimoto)
- •Chapter 8 Design and practice of small antennas II (K. Fujimoto)
- •Chapter 9 Evaluation of small antenna performance (H. Morishita)
- •Chapter 10 Electromagnetic simulation (H. Morishita and Y. Kim)
- •Chapter 11 Glossary (K. Fujimoto and N. T. Hung)
- •Acknowledgements
- •1 Introduction
- •2 Small antennas
- •3 Properties of small antennas
- •3.1 Performance of small antennas
- •3.1.1 Input impedance
- •3.1.4 Gain
- •3.2 Importance of impedance matching in small antennas
- •3.3 Problems of environmental effect in small antennas
- •4 Fundamental limitations of small antennas
- •4.1 Fundamental limitations
- •4.2 Brief review of some typical work on small antennas
- •5 Subjects related with small antennas
- •5.1 Major subjects and topics
- •5.1.1 Investigation of fundamentals of small antennas
- •5.1.2 Realization of small antennas
- •5.2 Practical design problems
- •5.3 General topics
- •6 Principles and techniques for making antennas small
- •6.1 Principles for making antennas small
- •6.2 Techniques and methods for producing ESA
- •6.2.1 Lowering the antenna resonance frequency
- •6.2.1.1 SW structure
- •6.2.1.1.1 Periodic structures
- •6.2.1.1.3 Material loading on an antenna structure
- •6.2.2 Full use of volume/space circumscribing antenna
- •6.2.3 Arrangement of current distributions uniformly
- •6.2.4 Increase of radiation modes
- •6.2.4.2 Use of conjugate structure
- •6.2.4.3 Compose with different types of antennas
- •6.2.5 Applications of metamaterials to make antennas small
- •6.2.5.1 Application of SNG to small antennas
- •6.2.5.1.1 Matching in space
- •6.2.5.1.2 Matching at the load terminals
- •6.2.5.2 DNG applications
- •6.3 Techniques and methods to produce FSA
- •6.3.1 FSA composed by integration of components
- •6.3.2 FSA composed by integration of functions
- •6.3.3 FSA of composite structure
- •6.4 Techniques and methods for producing PCSA
- •6.4.2 PCSA employing a high impedance surface
- •6.5 Techniques and methods for making PSA
- •6.5.2 Simple PSA
- •6.6 Optimization techniques
- •6.6.1 Genetic algorithm
- •6.6.2 Particle swarm optimization
- •6.6.3 Topology optimization
- •6.6.4 Volumetric material optimization
- •6.6.5 Practice of optimization
- •6.6.5.1 Outline of particle swarm optimization
- •6.6.5.2 PSO application method and result
- •7 Design and practice of small antennas I
- •7.1 Design and practice
- •7.2 Design and practice of ESA
- •7.2.1 Lowering the resonance frequency
- •7.2.1.1 Use of slow wave structure
- •7.2.1.1.1 Periodic structure
- •7.2.1.1.1.1 Meander line antennas (MLA)
- •7.2.1.1.1.1.1 Dipole-type meander line antenna
- •7.2.1.1.1.1.2 Monopole-type meander line antenna
- •7.2.1.1.1.1.3 Folded-type meander line antenna
- •7.2.1.1.1.1.4 Meander line antenna mounted on a rectangular conducting box
- •7.2.1.1.1.1.5 Small meander line antennas of less than 0.1 wavelength [13]
- •7.2.1.1.1.1.6 MLAs of length L = 0.05 λ [13, 14]
- •7.2.1.1.1.2 Zigzag antennas
- •7.2.1.1.1.3 Normal mode helical antennas (NMHA)
- •7.2.1.1.1.4 Discussions on small NMHA and meander line antennas pertaining to the antenna performances
- •7.2.1.2 Extension of current path
- •7.2.2 Full use of volume/space
- •7.2.2.1.1 Meander line
- •7.2.2.1.4 Spiral antennas
- •7.2.2.1.4.1 Equiangular spiral antenna
- •7.2.2.1.4.2 Archimedean spiral antenna
- •7.2.2.1.4.3.2 Gain
- •7.2.2.1.4.4 Radiation patterns
- •7.2.2.1.4.5 Unidirectional pattern
- •7.2.2.1.4.6 Miniaturization of spiral antenna
- •7.2.2.1.4.6.1 Slot spiral antenna
- •7.2.2.1.4.6.2 Spiral antenna loaded with capacitance
- •7.2.2.1.4.6.3 Archimedean spiral antennas
- •7.2.2.1.4.6.4 Spiral antenna loaded with inductance
- •7.2.2.2 Three-dimensional (3D) structure
- •7.2.2.2.1 Koch trees
- •7.2.2.2.2 3D spiral antenna
- •7.2.2.2.3 Spherical helix
- •7.2.2.2.3.1 Folded semi-spherical monopole antennas
- •7.2.2.2.3.2 Spherical dipole antenna
- •7.2.2.2.3.3 Spherical wire antenna
- •7.2.2.2.3.4 Spherical magnetic (TE mode) dipoles
- •7.2.2.2.3.5 Hemispherical helical antenna
- •7.2.3 Uniform current distribution
- •7.2.3.1 Loading techniques
- •7.2.3.1.1 Monopole with top loading
- •7.2.3.1.2 Cross-T-wire top-loaded monopole with four open sleeves
- •7.2.3.1.3 Slot loaded with spiral
- •7.2.4 Increase of excitation mode
- •7.2.4.1.1 L-shaped quasi-self-complementary antenna
- •7.2.4.1.2 H-shaped quasi-self-complementary antenna
- •7.2.4.1.3 A half-circular disk quasi-self-complementary antenna
- •7.2.4.1.4 Sinuous spiral antenna
- •7.2.4.2 Conjugate structure
- •7.2.4.2.1 Electrically small complementary paired antenna
- •7.2.4.2.2 A combined electric-magnetic type antenna
- •7.2.4.3 Composite structure
- •7.2.4.3.1 Slot-monopole hybrid antenna
- •7.2.4.3.2 Spiral-slots loaded with inductive element
- •7.2.5 Applications of metamaterials
- •7.2.5.1 Applications of SNG (Single Negative) materials
- •7.2.5.1.1.2 Elliptical patch antenna
- •7.2.5.1.1.3 Small loop loaded with CLL
- •7.2.5.1.2 Epsilon-Negative Metamaterials (ENG MM)
- •7.2.5.2 Applications of DNG (Double Negative Materials)
- •7.2.5.2.1 Leaky wave antenna [116]
- •7.2.5.2.3 NRI (Negative Refractive Index) TL MM antennas
- •7.2.6 Active circuit applications to impedance matching
- •7.2.6.1 Antenna matching in transmitter/receiver
- •7.2.6.2 Monopole antenna
- •7.2.6.3 Loop and planar antenna
- •7.2.6.4 Microstrip antenna
- •8 Design and practice of small antennas II
- •8.1 FSA (Functionally Small Antennas)
- •8.1.1 Introduction
- •8.1.2 Integration technique
- •8.1.2.1 Enhancement/improvement of antenna performances
- •8.1.2.1.1 Bandwidth enhancement and multiband operation
- •8.1.2.1.1.1.1 E-shaped microstrip antenna
- •8.1.2.1.1.1.2 -shaped microstrip antenna
- •8.1.2.1.1.1.3 H-shaped microstrip antenna
- •8.1.2.1.1.1.4 S-shaped-slot patch antenna
- •8.1.2.1.1.2.1 Microstrip slot antennas
- •8.1.2.1.1.2.2.2 Rectangular patch with square slot
- •8.1.2.1.2.1.1 A printed λ/8 PIFA operating at penta-band
- •8.1.2.1.2.1.2 Bent-monopole penta-band antenna
- •8.1.2.1.2.1.3 Loop antenna with a U-shaped tuning element for hepta-band operation
- •8.1.2.1.2.1.4 Planar printed strip monopole for eight-band operation
- •8.1.2.1.2.2.2 Folded loop antenna
- •8.1.2.1.2.3.2 Monopole UWB antennas
- •8.1.2.1.2.3.2.1 Binomial-curved patch antenna
- •8.1.2.1.2.3.2.2 Spline-shaped antenna
- •8.1.2.1.2.3.3 UWB antennas with slot/slit embedded on the patch surface
- •8.1.2.1.2.3.3.1 A beveled square monopole patch with U-slot
- •8.1.2.1.2.3.3.2 Circular/Elliptical slot UWB antennas
- •8.1.2.1.2.3.3.3 A rectangular monopole patch with a notch and a strip
- •8.1.2.1.2.3.4.1 Pentagon-shape microstrip slot antenna
- •8.1.2.1.2.3.4.2 Sectorial loop antenna (SLA)
- •8.1.3 Integration of functions into antenna
- •8.2 Design and practice of PCSA (Physically Constrained Small Antennas)
- •8.2.2 Application of HIS (High Impedance Surface)
- •8.2.3 Applications of EBG (Electromagnetic Band Gap)
- •8.2.3.1 Miniaturization
- •8.2.3.2 Enhancement of gain
- •8.2.3.3 Enhancement of bandwidth
- •8.2.3.4 Reduction of mutual coupling
- •8.2.4 Application of DGS (Defected Ground Surface)
- •8.2.4.2 Multiband circular disk monopole patch antenna
- •8.2.5 Application of DBE (Degenerated Band Edge) structure
- •8.3 Design and practice of PSA (Physically Small Antennas)
- •8.3.1 Small antennas for radio watch/clock systems
- •8.3.2 Small antennas for RFID
- •8.3.2.1 Dipole and monopole types
- •8.3.2.3 Slot type antennas
- •8.3.2.4 Loop antenna
- •Appendix I
- •Appendix II
- •References
- •9 Evaluation of small antenna performance
- •9.1 General
- •9.2 Practical method of measurement
- •9.2.1 Measurement by using a coaxial cable
- •9.2.2 Method of measurement by using small oscillator
- •9.2.3 Method of measurement by using optical system
- •9.3 Practice of measurement
- •9.3.1 Input impedance and bandwidth
- •9.3.2 Radiation patterns and gain
- •10 Electromagnetic simulation
- •10.1 Concept of electromagnetic simulation
- •10.2 Typical electromagnetic simulators for small antennas
- •10.3 Example (balanced antennas for mobile handsets)
- •10.3.2 Antenna structure
- •10.3.3 Analytical results
- •10.3.4 Simulation for characteristics of a folded loop antenna in the vicinity of human head and hand
- •10.3.4.1 Structure of human head and hand
- •10.3.4.2 Analytical results
- •11 Glossary
- •11.1 Catalog of small antennas
- •11.2 List of small antennas
- •Index
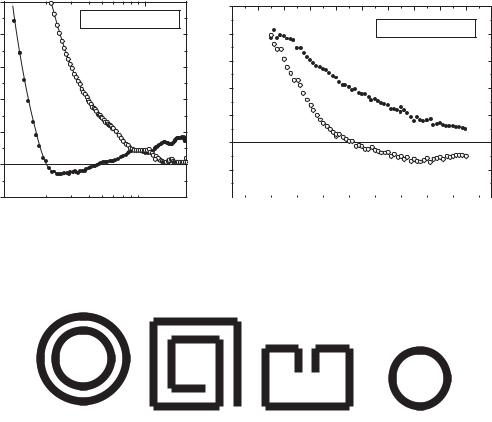
220 |
Design and practice of small antennas I |
|
|
Permeability μ
5
4
3
2
1
0
−1 1
|
BaFe9(Ti0.5Co0.5)3O19 |
|
5 |
|
|
|
|
Permalloy composite |
|
|
|
|
4 |
|
|
|
|
|
|
|
|
μ |
3 |
|
|
μr′′ |
|
|
|
|
Permeability |
2 |
μr′′ |
|
μr′ |
|
|
||
|
1 |
|
||
|
|
μr′ |
||
|
|
0 |
|
|
|
|
|
|
|
|
|
|
−1 |
|
|
|
|
−2 |
|
|
|
|
|
|
|
|
|
|
|
|
|
|
|
|
|
|
|
|
|
|
|
|
10 |
0 |
2 |
4 |
6 |
8 10 12 14 16 18 20 |
||||||||||||||||||||||
Frequency (GHz) |
|
|
|
|
|
|
|
|
|
|
|
Frequency (GHz) |
|||||||||||||||
(a) |
|
|
|
|
|
|
|
|
|
|
|
|
|
(b) |
Figure 7.208 Dispersion characteristics of magnetic composite materials; (a) BaFe material and
(b) Permalloy composite material ([108], copyright C 2008 IEEE).
|
|
|
|
|
|
|
|
|
|
|
|
|
|
|
|
|
|
|
|
|
|
|
|
|
|
|
|
|
|
|
|
|
|
|
|
|
|
|
|
|
|
|
|
|
|
|
|
|
|
|
|
|
|
|
|
(a) |
(b) |
(c) |
(d) |
Figure 7.209 Examples of MNG material unit: (a) SRR, (b) CLL, (c) SR, and (d) omega-shaped structure [101,109,110 and 111].
Artificial MNG materials can be implemented by other means; four examples are illustrated in Figure 7.209, (a) SRR (Split Ring Resonator) [101], (b) capacitive-loaded loop (CLL) [109], (c) spiral rings (SR) [110], and (d) omega shaped structure [111]. Meanwhile, artificial ENG (epsilon negative) material, as is mentioned above, can be constituted from an array of thin wires (Figure 6.18) [102]. Meander lines arranged in planar structure and helical windings are used as inductor materials, which can be a substitute for ENG materials for the purpose of space resonance or matching [109]. Applications of these artificial MMs to small antennas will be shown in the next section.
7.2.5.1Applications of SNG (Single Negative) materials
7.2.5.1.1 Mu-Negative (MNG) metamaterials (MM) 7.2.5.1.1.1 Circular patch antenna
Implementation of a miniaturized circular patch antenna loaded with MNG MM has been introduced in [112 a, b], in which a thorough theoretical analysis of the magnetic field distribution underneath the patch, and the design of magnetic inclusions related
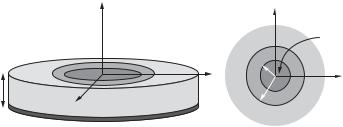
7.2 Design and practice of ESA |
221 |
|
|
zˆ |
yˆ |
ε2, μ2 ε1, μ1
MNG |
yˆ |
|
|
a |
xˆ |
||
d |
|
1 MNG |
|
DPS |
a |
||
xˆ |
|||
|
|||
|
|
DPS |
|
(a) |
|
(b) |
Figure 7.210 Geometry of a circular patch antenna loaded with an MNG MM [112a and b].
with their location, arrangement, and alignment, are shown. To realize the MNG MM, multiple spiral ring resonators (MSRR) (Figure 7.209(c)) are employed as an appropriate selection, having the dimensions that fit with the limited thickness of the space between the patch and the ground plane, while their required resonance frequency is kept despite their small electrical size. The MSRR are aligned according to the expected preferred direction of the magnetic field at their location. Geometry of the patch antenna loaded with an MNG MM is illustrated in Figure 7.210, showing (a) the 3D view and (b) the top view of the antenna, which is partially loaded with an MNG MM underneath the patch. With this arrangement, resonant modes can be excited on the patch, even though the dimensions are significantly smaller than the operating wavelength. Even with the small dimensions, the MNG MM must provide an effective negative permeability in its complex near-field interaction with the feed and the patch. As the MNG MM has dispersion property, the permeability μ1 is given by assuming the Drude dispersion relation as
μ1 = μ0(1 − ω2p/(ω2 − jωδ)) |
(7.72) |
where μ0 is the free space permeability, ωp is the magnetic plasma frequency, and δ is the damping factor. Here the MNG media required to design an antenna shown in Figure 7.210 for operating at 0.47 GHz is assumed to have the dispersion characteristic as shown in Figure 7.211, where the variation of the relative permeability μ1/μ0 with respect to the frequency is given [112a]. In this case, the geometrical parameters of the antenna used are: radius of the substrate a = 20 mm, radius of the MNG media a1 = 12 mm, thickness of the substrate d = 5 mm, and δ = 0.01 GHz. Then the required value of the relative permeability Re[μ1/μ0] = –2.17 at 0.47 GHz. Geometrical sketch of the MNG integrated patch is depicted in Figure 7.212(a). In the figure, (b) illustrates arrangement of the MSR (Multiple Split Ring), viewing from the top of the patch. With this MSR arrangement, almost uniform field distributions are obtained, thus exciting the desired TM11 mode effectively all over the patch. The presence of the MSR highly affects the current distributions underneath the patch, closing itself in an electrically small resonant loop, which produces the desired radiation patterns and gain [112a]. The return-loss characteristic is depicted in Figure 7.213, which shows matching features of the antenna at two frequencies. The antenna operates at higher frequencies (around 2.44 GHz, determined by the electrical size of the patch) as well as lower frequencies. At higher frequencies, the substrate behaves as a homogeneous material having the
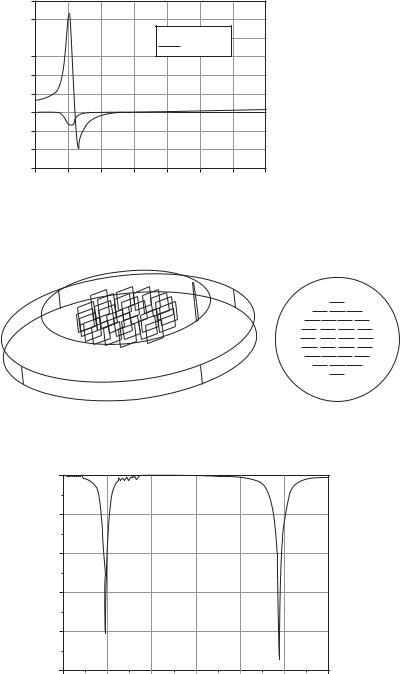
222 |
Design and practice of small antennas I |
|
|
Relative permeability
30
25
20 Re [ μ1]/μ0
Im [ μ1]/μ0
15
10 |
|
|
|
|
|
|
|
5 |
|
|
|
|
|
|
|
0 |
|
|
|
|
|
|
|
−5 |
|
|
|
|
|
|
|
–10 |
|
|
|
|
|
|
|
–15 |
|
|
|
|
|
|
|
0.3 |
0.4 |
0.5 |
0.6 |
0.7 |
0.8 |
0.9 |
1.0 |
Frequency (GHz)
Figure 7.211 Dispersion characteristic model of the MNG MM required to design the antenna of Figure 7.210 ([112a], copyright C 2008 IEEE).
Figure 7.212 Geometry of SR implementation inside the cylindrical core cavity underneath the patch: (a) 3D view and (b) top view of the patch, ([112a], copyright C 2008 IEEE).
Return loss (dB)
0 dB
–5 dB
–10 dB
–15 dB
–20 dB
–25 dB
0.0 |
0.5 |
1.0 |
1.5 |
2.0 |
2.5 |
3.0 |
Frequency (GHZ)
Figure 7.213 Return-loss characteristics ([112a], copyright C 2008 IEEE).
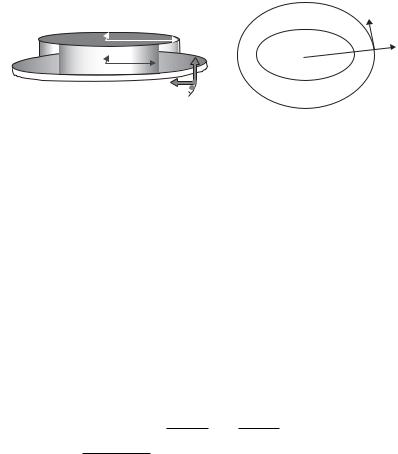
7.2 Design and practice of ESA |
223 |
|
|
|
|
|
|
μ2, ε2 |
DPS |
ηˆ |
|
|
|
|
|
||
|
b2 |
a2 |
|
μ1, ε1 |
MNG |
ˆ |
d |
b1 |
z |
|
ξ |
||
|
a1 |
|
ξ = ξ1 |
|
||
|
|
|
|
|||
|
|
|
x |
|
|
|
|
|
|
y |
|
ξ = ξ2 |
|
Figure 7.214 Geometry of an elliptical patch antenna partially loaded with an MNG MM core surrounded by a DPS shell ([113], copyright C 2010 IEEE).
constitutive parameters ε = ε2 = 2.33 ε0 and μ = μ2 = μ0. The simulated resonance frequencies obtained by using the full-wave commercial code CST Microwave Studio are very close to the predictions using the theoretical cavity model, where the radius of the circular patch antenna is only 20 mm. Radiation patterns are nearly the same as that of an ordinary circular patch antenna. The ground plane in this model has a radius of 40 mm. Simulated gains and efficiencies at the two resonance frequencies, 0.47 GHz and 2.44 GH respectively, are 3.1 dBi, 6.3 dBi and 0.67, 0.92.
7.2.5.1.1.2 Elliptical patch antenna
An elliptical patch antenna loaded with MNG material was studied and showed the possibility of miniaturization of the antenna size and advantages of using the elliptical geometry compared to the use of circular geometry [113]. The antenna geometry is illustrated in Figure 7.214, which shows an elliptically shaped MNG material core of semi-axes a1 and b1 partially loaded underneath the elliptical patch and surrounded by an elliptically shaped DPS shell of semi-axes a2 and b2. The elliptical shape parameters are
the semi-focal length F = a12 − b12 = a22 − b22, and the eccentricity of the elliptical
patch e = 1 − (b2/a2)2 = 1/cosh(ξ 2)(0 ≤ e < 1). Here the Cartesian coordinates x and y are related with the elliptical system coordinates ξ and η by
x = F cos ξ cos η
(7.73)
y = F sin ξ sin η.
Another parameter is the filling ratio Г = (volume of the material core)/(overall volume underneath the patch) (0 < Г < 1).
Based on the full-wave simulation, the antenna performances are analyzed. The parameters used are: surface area of the patch A = 4π cm2, Г = 0.35, e = 0.7, ε1 = ε2 = ε0, and μ2 = μ0. Here, ε1, μ1, and ε2, μ2, respectively, are the constitutive parameters of the MNG and the DPS materials. Since the material has dispersive characteristic, different modes can be excited by the different set of magnetic plasma frequencies. By the selection of ωmp = 1.286 GHz, the odd mode is excited around the design frequency 0.5 GHz, while by the selection of ωmp = 0.707 GHz, the even mode at the same resonance frequency is excited. Simulated return loss S11 is given in Figure 7.215, which indicates that near the design frequency, resonances are obtained in two cases with even and odd mode properties. In the figure, dual-band effect is observed in the odd-mode