
- •VOLUME 1
- •CONTRIBUTOR LIST
- •PREFACE
- •LIST OF ARTICLES
- •ABBREVIATIONS AND ACRONYMS
- •CONVERSION FACTORS AND UNIT SYMBOLS
- •ABLATION.
- •ABSORBABLE BIOMATERIALS.
- •ACRYLIC BONE CEMENT.
- •ACTINOTHERAPY.
- •ADOPTIVE IMMUNOTHERAPY.
- •AFFINITY CHROMATOGRAPHY.
- •ALLOYS, SHAPE MEMORY
- •AMBULATORY MONITORING
- •ANALYTICAL METHODS, AUTOMATED
- •ANALYZER, OXYGEN.
- •ANESTHESIA MACHINES
- •ANESTHESIA MONITORING.
- •ANESTHESIA, COMPUTERS IN
- •ANGER CAMERA
- •ANGIOPLASTY.
- •ANORECTAL MANOMETRY
- •ANTIBODIES, MONOCLONAL.
- •APNEA DETECTION.
- •ARRHYTHMIA, TREATMENT.
- •ARRHYTHMIA ANALYSIS, AUTOMATED
- •ARTERIAL TONOMETRY.
- •ARTIFICIAL BLOOD.
- •ARTIFICIAL HEART.
- •ARTIFICIAL HEART VALVE.
- •ARTIFICIAL HIP JOINTS.
- •ARTIFICIAL LARYNX.
- •ARTIFICIAL PANCREAS.
- •ARTERIES, ELASTIC PROPERTIES OF
- •ASSISTIVE DEVICES FOR THE DISABLED.
- •ATOMIC ABSORPTION SPECTROMETRY.
- •AUDIOMETRY
- •BACTERIAL DETECTION SYSTEMS.
- •BALLOON PUMP.
- •BANKED BLOOD.
- •BAROTRAUMA.
- •BARRIER CONTRACEPTIVE DEVICES.
- •BIOCERAMICS.
- •BIOCOMPATIBILITY OF MATERIALS
- •BIOELECTRODES
- •BIOFEEDBACK
- •BIOHEAT TRANSFER
- •BIOIMPEDANCE IN CARDIOVASCULAR MEDICINE
- •BIOINFORMATICS
- •BIOLOGIC THERAPY.
- •BIOMAGNETISM
- •BIOMATERIALS, ABSORBABLE
- •BIOMATERIALS: AN OVERVIEW
- •BIOMATERIALS: BIOCERAMICS
- •BIOMATERIALS: CARBON
- •BIOMATERIALS CORROSION AND WEAR OF
- •BIOMATERIALS FOR DENTISTRY
- •BIOMATERIALS, POLYMERS
- •BIOMATERIALS, SURFACE PROPERTIES OF
- •BIOMATERIALS, TESTING AND STRUCTURAL PROPERTIES OF
- •BIOMATERIALS: TISSUE-ENGINEERING AND SCAFFOLDS
- •BIOMECHANICS OF EXERCISE FITNESS
- •BIOMECHANICS OF JOINTS.
- •BIOMECHANICS OF SCOLIOSIS.
- •BIOMECHANICS OF SKIN.
- •BIOMECHANICS OF THE HUMAN SPINE.
- •BIOMECHANICS OF TOOTH AND JAW.
- •BIOMEDICAL ENGINEERING EDUCATION
- •BIOSURFACE ENGINEERING
- •BIOSENSORS.
- •BIOTELEMETRY
- •BIRTH CONTROL.
- •BLEEDING, GASTROINTESTINAL.
- •BLADDER DYSFUNCTION, NEUROSTIMULATION OF
- •BLIND AND VISUALLY IMPAIRED, ASSISTIVE TECHNOLOGY FOR
- •BLOOD BANKING.
- •BLOOD CELL COUNTERS.
- •BLOOD COLLECTION AND PROCESSING
- •BLOOD FLOW.
- •BLOOD GAS MEASUREMENTS
- •BLOOD PRESSURE MEASUREMENT
- •BLOOD PRESSURE, AUTOMATIC CONTROL OF
- •BLOOD RHEOLOGY
- •BLOOD, ARTIFICIAL
- •BONDING, ENAMEL.
- •BONE AND TEETH, PROPERTIES OF
- •BONE CEMENT, ACRYLIC
- •BONE DENSITY MEASUREMENT
- •BORON NEUTRON CAPTURE THERAPY
- •BRACHYTHERAPY, HIGH DOSAGE RATE
- •BRACHYTHERAPY, INTRAVASCULAR
- •BRAIN ELECTRICAL ACTIVITY.
- •BURN WOUND COVERINGS.
- •BYPASS, CORONARY.
- •BYPASS, CARDIOPULMONARY.
28.Fung YC. Biomechanics—Circulation 2nd ed. New York: Springer-Verlag; 1997.
29.Pedley TJ. Pulmonary Fluid Dynamics. Ann Rev Fluid Mech 1977;9:229–274.
30.Ferry JD. Viscoelastic Properties of Polymers. 3rd ed. New York: John Wiley; 1980.
31.Whitemore RL. Rheology of the Circulation. New York: Pergamon Press; 1968.
32.Tran-Son-Tay R. Techniques for Studying the Effects of Physical Forces on Mammalian Cells and for Measuring Cell Mechanical Properties. In: Frangos JA, editor. Physical Forces and the Mammalian Cell. New York: Academic Press; 1993;p. 1–59.
33.Tran-Son-Tay R, Beaty BB, Acker DN, Hochmuth RM. Magnetically Driven, Acoustically Tracked Translating Ball Rheometer for Small, Opaque Samples. Rev Sci Instru 1988; 59:1399–1404.
34.Tran-Son-Tay R. A Microrheometer for Studying the Rheological Properties of Sickle Cell Suspensions and Hemoglobin. Proceedings of The Fourth China-Japan-USA-Singapore Conference on Biomechanics. In: Yang G, Hayashi K, Woo SL-Y, Goh JCH, editors. International Academic Publishers; 1995; p. 429–432.
35.Waugh RE, Hochmuth RM. Mechanics and Deformability of Hematocytes. In: Schneck DJ, Bronzino JD, editors. Biome- chanics—Principles and Applications. New York: CRC Press; 2202; 227–239.
36.Linderkamp O, Ruef P, Zilow EP, Hoffmann GF. Impaired deformability of erythrocytes and neutrophils in children with newly diagnosed insulin-dependent diabetes mellitus. Diabetologia 1999;42:865–869.
37.Perrault CM, et al. Altered Rheology of Lymphocytes in the Diabetic Mouse. Diabetologia 2004;47:1722–1726.
38.Tsai MA, Frank RS, Waugh RE. Paasive Mechanical Behavior of Human Neutrophils: Effect of Cytochalasin B. Biophys J 1994;66:2166.
39.Thomas SJ, et al. Effects of X-Ray Radiation on the Rheological Properties of Platelets and Lymphocytes. Transfusion 2003;43:502–508.
40.Evans EA,, Yeung A. Apparent viscosity and Cortical Tension of Blood Granulocytes Determined by Micropipet Aspiration. Biophys J 1989;56:151.
41.Hochmuth RM, et al. Viscosity of Passive Neutrophils Undergoing Small Deformations. Biophy J 1993;64:1596–1601.
42.Needham D, Hochmuth RM. Rapid Flow of Passive Neutrophils into a 4 mm Pipet and Measurement of Cytoplasmic Viscosity. J Biomech Eng 1990;112:269.
43.Tran-Son-Tay R, Needham D, Hochmuth RM. Recovery of Passive Neutrophils after Large Deformation: Liquid Drop Model. Proceedings of the ASME, Adv Bioeng 1991;20:421–424.
44.Kan H-C, et al. Effects of Nucleus on Leukocyte Recovery. Ann Biomed Eng 1999;27(5):648–655.
45.Shyy W, et al. Moving Boundaries in Micro-Scale Biofluid Dynamics. Appl Mecha Rev 2001;54:405–453.
46.Schmid-Schoenbein H, et al. A Counter-Rotating ‘‘Rheoscope Chamber for the Study of the Microrheology of Blood Cell Aggregation by Microscopic Observation and Microphotometry. Microvasc Res 1973;6:366–376.
47.Tran-Son-Tay R, Sutera SP, Rao PR. Determination of RBC Membrane Viscosity from Rheoscopic Observations of TankTreading Motion. Biophy J 1984;46:65–72.
48.Tran-Son-Tay R, Sutera SP, Zahalak GI, Rao PR. Membrane Stress and Internal Pressure in Red Blood Cells Freely Suspended in Shear Flow. Biophys J 1987;51:915–924.
49.Glover S, et al. Phosphorylation of Tyrosine 397 Critically Mediates Gastrin-Releasing Peptide’s Morphogenic Properties. J Cellular Physiol 2004;199:77–88.
BLOOD, ARTIFICIAL |
511 |
50.Voldman J, Gray ML, Schmidt MA. Microfabrication in biology and medicine. Annu Rev Biomed Eng 1999;1:401–425.
51.Shyy W, Tran-Son-Tay R, N’Dri N. Micro-Nano Coupling in Biological Systems. In: Harik VM, Luo LS, Salas M, editors. Nano-Scale Mechanics of Solid and Liquid Materials Systems. The Netherlands: Kluwer Academic; 2003.
52.Maddou M. Fundamentals of Microfabrication: The Science of Miniaturization. 2nd ed. Washington (DC): CRC Press; 2001.
53.Xia Y, Whitesides GM. Soft lithography. Ann Rev Mater Sci 1998;28:153–184.
54.Branham ML, et al. Rapid Prototyping of Micropatterned Substrates Using Conventional Laser Printers. J Materials Res 2002;17(7):1559–1562.
Reading List
Adjizian JC, et al. Clinical applications to the Ektacytometer. Clin Hemorheol 1984;4:245–254.
Chien S, et al. Effects of hematocrit and plasma proteins of human blood rheology at low shear rates. J Appl Physiol 1966;21:81–87.
See also CELL COUNTERS, BLOOD; HEMODYNAMICS.
BLOOD, ARTIFICIAL
BRIAN WOODCOCK
University of Michigan
Ann Arbor, Michigan
INTRODUCTION
Blood has such a multitude of physiological functions; it provides a circulating volume to transport substrate and metabolites, and it transports the most valuable of substrates, oxygen. It is an organ intimately involved in the immune system, delivering antibodies and cellular elements to sites of infection. It carries the instruments for coagulation. It is the communication highway for the endocrine system. It is a metabolic organ containing enzyme systems to convert molecules to active and inactive forms. Blood is intimately involved in temperature regulation. The manufacture of an artificial substitute to fulfill all those purposes is beyond the capability of current science. However, several of the functional capabilities of blood have been incorporated into various blood substitutes.
The most basic function of blood is to provide a circulating volume for transportation of substrate and metabolites. Supplementation of intravascular volume with crystalloid and colloid fluids has been a part of medical practice for a century. Recent progress has concentrated on the development of substitute solutions that can transport oxygen. These solutions are known as oxygen therapeutics or red cell substitutes.
Currently, the only available oxygen therapeutic is typed and cross-matched allogeneic human blood. This is made available in the civilian setting by the Red Cross, the American Blood Centers, and the blood banking system. Blood shortages, due to increasing blood usage and declining blood donations (1), are one of the factors driving the

512 BLOOD, ARTIFICIAL
search for alternatives. In the past two decades there has also been an increasing concern regarding the infectious risks of blood borne pathogens (2,3). Awareness of the potential significance of the problem occurred with the increasing risk of human immunodeficiency virus (HIV) transmission from blood transfusion during the 1980s. Improved screening for HIV in the 1990s saw a dramatic improvement in blood safety so that now the risk of contracting HIV from a unit of blood is approaching 1/1,000,000. But there are still substantial concerns regarding not only the risk of contracting acquired immune deficiency syndrome (AIDS) or hepatitis, and also of other infectious diseases newly recognized as possibly being transmissible by transfusion, such as bovine spongiform encephalopathy (mad cow disease) and West Nile virus.
Another concern leading to the need for blood substitutes is that some groups Jehovah’s Witnesses, have religious beliefs that cause them to refuse all blood products.
Banked blood is often wasted while active bleeding continues in the surgical setting. While hemorrhage continues, blood products administered are rapidly lost through the site of bleeding. Blood substitutes could be used as a resuscitation bridge until bleeding is controlled (4).
Initial research on artificial blood was lead by the U.S. military, which needed a ready supply of a substitute that could be stored easily and indefinitely in the field and not require typing or cross-matching. These considerations would also make a blood substitute valuable to the emergency medical services in ambulance and helicopter transfers.
All these concerns have stimulated efforts to develop red cell substitutes for use in the routine clinical setting. In an initial approach, prior to World War II, the defense department sought a hemoglobin solution that could be stored indefinitely at room temperature, preferably in a powdered form to be dissolved in normal saline, and that could be transfused without a need for cross-matching. Although the development of a reconstitutable powder has not been feasible, there are several hemoglobin-based products that are in various stages of clinical testing (5).
Other molecules apart from hemoglobin have been assessed for the function of oxygen transportation. Most success has been achieved with emulsions of perfluorochemicals.
Perfluorochemicals are inert liquids, which have a solubility for oxygen and carbon dioxide 20 times that of water. These liquids are immiscible in water and an emulsion form is required to allow them to mix with the recipient’s blood after administration. A comparison of the advantages and disadvantages of perfluorocarbon and hemoglobin solutions is shown in Table 1.
Emulsions of perfluorochemicals have completed animal testing and have been investigated in the clinical setting. However, difficulties in their use have been observed, to date they have not been made available for routine use.
CURRENT RISKS OF BANKED BLOOD
Risks of Transfusion
Blood transfusion is safer today than it has ever been, with a death rate for each blood transfusion of 1 in 300,000 (6).
Table 1. Comparison of Perfluorocarbon and Hemoglobin
Solutions
|
Advantages |
Disadvantages |
|
|
|
Perfluorocarbon |
High O2 Solubility |
Requires high PO2 |
Emulsions |
Inert |
Long tissue life |
|
Ample supply |
Short vascular life |
|
|
Toxicities |
|
|
|
Hemoglobin |
Carry O2 at normal PaO2 |
Vasoconstriction |
Solutions |
Unloads like RBCs |
Supply |
|
May be stored dry? |
Short vascular life |
|
|
Toxicities |
|
|
|
Two-thirds of these deaths are due to clerical errors (i.e., the wrong blood given to the wrong patient). Other risks associated with blood transfusion include infection and immune reactions (7,8). However, 20 million blood transfusions are administered each year in the United States, with an impressive safety record (9).
Infection
The risk of transmission of infection includes viral agents, other exotic infectious agents, and the risk of bacterial contamination of blood products. Blood donors with a history of risk factors are excluded from donation. Screening donated units and elimination of units that contain known infectious agents eliminates most of the remaining risk of infection.
HIV
The risk of HIV transmission from blood transfusion has caused the most public concern, though it is difficult to accurately assess the true risk of transmission because it is small and cases can be determined only after a significant period. Initial testing for HIV consisted of antibody testing alone, but this was felt to leave a risk of HIV from individuals who were infected, but had not yet sero-converted. In March 1996, HIV antigen p24 testing was instituted in the United States and only 3 out of 18 million units were identified as being antibody negative and antigen positive over the next 18 months (10). Blood donations are now tested for HIV-1 and HIV-2 (11). The apparent risk of HIV transmission is currently 1 in 1,000,000.
Hepatitis
Hepatitis has a higher prevalence; 1:60,000 for hepatitis B, and 1:103,000 for hepatitis C, but is much less feared by the general public. Antigen screening tests have reduced the risk of post-transfusion hepatitis (B or C) to < 1 in 34,000 (12). Hepatitis G has more recently been recognized, and has a high incidence worldwide of 1 (13)–7% (14). Approximately 2% of blood donors and 15–20% of intravenous drug abusers in the United States have detectable hepatitis G (15). It may be identified by the polymerase chain reaction test, but this has not been implemented as a routine screening test. Fortunately, it appears that the hepatitis G virus is not responsible for non-A, non-B, non-C post-
transfusion hepatitis and the results of infection appear to be minimal (16,17), although there is a weak link between hepatitis G and fulminant hepatitis in rare cases (18).
Other Viruses
Creutzfeldt–Jakob disease (vCJD) can be transmitted by transfer of central nervous system tissue (or extract). However, no cases have been definitively linked to blood transfusion (19).
In the United Kingdom an outbreak of bovine spongiform encephalopathy (BSE) or ‘‘Mad Cow Disease’’ has led to concern that a new variant vCJD could be transferred to the human population through consumption of contaminated beef products. Transmission of BSE by blood transfusion can occur in sheep (20). In the United Kingdom, blood products for transfusion are leucodepleted, which is thought to reduce the risk of transmission of vCJD. The possibility that infection might occur has led the U.S. Food and Drug Administration (FDA) to institute a policy ‘‘deferring’’, that is, declining, blood donations from anyone who has lived in the United Kingdom for a cumulative period of more than 6 months during the years 1980– 1996 (21).
West Nile virus transmission has occurred in four patients who received solid organ donations from an infected donor. The organ donor had received blood transfusions from 63 donors, and follow up of those donors showed that one of them was viremic at the time of donation (22).
Bacterial contamination of stored blood is rare (1:500,000), but has a mortality rate of 25–80%. The most common infectious contaminants in red cells are gramnegative species such as Pseudomonas or Yersinia (23). Platelets are stored at room temperature, allowing rapid bacterial proliferation, and there is a risk of 1:3000–7000 of bacterial infection with these units. Bacterial contamination of platelets is typically with Gram-positive staphylococci.
Immune Reactions
Minor immune reactions, such as febrile reactions, are common and may be discomforting to the patient, but are not associated with significant morbidity, though the transfusion may have to be stopped and the product discarded. The risk of serious immune reactions is small, but present. Most acute hemolytic reactions are due to clerical errors, because cross-matching should predict and prevent these events. However, immune reactions remain the most common cause of fatality associated with transfusions.
Graft versus host disease may occur rarely after transfusion, most commonly after transfusion of nonirradiated blood components to patients with immunodeficiency. Transfusion-associated graft versus host disease has a high mortality and is rapidly fatal. Immunodeficienct patients should receive irradiated units. Immunocompetent individuals may develop graft versus host disease if common histocompatibility leukocyte antigen haplotypes between the donor and recipient prevent destruction of stem cells transfused. This can occur between first-degree family members and therefore, relative-to-patient-directed
BLOOD, ARTIFICIAL |
513 |
donations, which are often preferred by patients because of a perceived reduction in risk of infection, may in fact carry an increased risk of initiating transfusion-associated graft versus host disease.
Transfusion-related immunomodulation has been recognized since the mid-1970s, but is not well quantified. Exposure to allogeneic blood can cause both allosensitization and immunosuppression. Studies have demonstrated a beneficial effect of allogeneic blood transfusion on transplant organ survival, but increases in the rates of cancer recurrence and postoperative infection have also been noted (23,24). Leukocyte depletion and removal of plasma may ameliorate the effects of TNFsuppression and interleukin induction (25).
The risk of infection and concerns regarding immune reactions and immunomodulation have been a large incentive to the development of oxygen-carrying colloids.
PERFLUOROCHEMICAL EMULSIONS
Perfluorochemicals (PFCs) are chemically inert liquids with a high solubility for gases. The PFCs that have been used as blood substitutes are 8–10-carbon atom structures that are completely fluorinated. The PFCs are chemically inert, clear, odorless liquids with a density nearly twice that of water. The solubility of PFCs for oxygen is nearly 20 times that of water.
In 1965, Clark and Gollan (26) performed an experiment to see whether an animal could survive if it breathed liquid PFC equilibrated with 1 atm of oxygen. A rat could be submerged beneath this liquid for 30 min and be retrieved in good condition. Respiration of liquid PFC allowed oxygen absorption from the lungs together with CO2 excretion.
An intravenous injection of PFC is immediately lethal because the injectate is immiscible with water and forms a liquid embolus. An emulsion of an immiscible liquid can, however, mix with water or blood. In 1968, Gehes (27) produced a microemulsion (particle size, 0.1 mm) of a PFC in normal saline. An exchange transfusion could be done, eliminating all normal blood elements, and a rat with a hemoglobin of 0 could survive breathing 100% oxygen.
Because of the inert nature of these compounds, they are not metabolized, but are cleared from the vascular space by the reticulo-endothelial system (RES), and ultimately collected in the liver and spleen. Eventually, the PFC slowly leaves the body as vapor in the respiratory gas.
Perfluorochemical Oxygen Content
Because PFCs transport oxygen by simple solubility, the amount of oxygen they carry is directly proportional to the percentage of PFC in the bloodstream and to the PaO2.
Hemoglobin carries most of the oxygen in whole blood and does so in a nonlinear fashion. The plot of oxygen content against PaO2 for hemoglobin, known as the oxygen dissociation curve, is seen in Fig. 1. Perfluorochemicals (PFCs) carry oxygen by direct solubility, as does plasma. Because of the shape of the oxygen dissociation curve, hemoglobin is fully saturated and carries little or no more oxygen above a PO2 of 90 mmHg (11.99 kPa). Because oxygen content dissolved in plasma, or carried by PFCs,
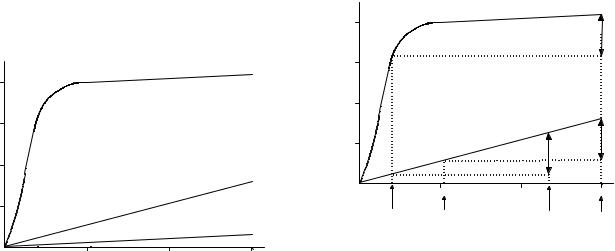
514 BLOOD, ARTIFICIAL
is linearly related to PO2, additional oxygen is dissolved in the plasma phase or by PFC as oxygen tension increases.
Arterial content of oxygen (CaO2) is defined as the volume of oxygen in milliliters (mL) carried by each 100 mL of blood and is defined as follows:
CaO2 ¼ ðHb 1:34 SaO2Þ þ ð0:003 PaO2Þ
ð1Þ
20 mL=100 mL
(Hb ¼ hemoglobin: SaO2, arterial oxygen saturation: PaO2, arterial oxygen tension)
With a normal 15 g of hemoglobin and normal PaO2 and SaO2 values of 90 mmHg (11.99 kpa) and 97%, respectively, an arterial oxygen content of 20 mL dL 1 is obtained.
When blood contains an oxygen carrying PFC, the oxygen content equation requires a third term to represent the contribution from perfluorocarbon.
CaO2 ¼ ðHb 1:34 SaO2Þ þ ð0:003 PaO2Þ
þð0:057 Fct=100 PaO2Þ ð2Þ
where Fct ¼ fluorocrit, which is the fraction of the blood volume that is PFC (analogous to the Hct).
Note that the solubility factor of PFC in the third term should be 0.06, that is, 20 times that of the solubility factor for oxygen in plasma (0.003), however, it is reduced by the amount of the plasma solubility factor to account for the plasma displaced by the presence of PFC.
The PFCs carry much more oxygen than plasma, but hemoglobin itself is able to carry much more than any PFC. Figure 1 shows that blood with a Hct of 45% will have a CaO2 of 20 mL/100 mL at a PO2 of 100 mmHg (13.33 kPa), but a solution with a Fct of 45% would have an oxygen content of 2.7 mL/100 mL. Because the PFC carries oxygen by direct solubility, it also releases it in direct proportion to the PO2, unlike the cooperative binding effect of Hg, with which the PO2 has to fall below the elbow of the curve for
|
20 |
Blood Hct 45% |
|
|
|
|
|
|
|
2 mL |
15 |
|
|
|
C mL/100 |
10 |
|
|
|
O |
|
|
|
|
a |
|
|
|
|
|
|
PFC |
45% |
|
|
5 |
|
|
|
|
|
|
|
|
|
|
Plasma |
|
|
|
100 |
200 |
300 |
|
|
|
PO2 mmHg |
|
Figure 1. Oxygen content plotted against PO2 for whole blood, plasma, and a perfluorochemical emulsion, with a 45% content of PFC. Hemoglobin saturates at a PO2 of 100 mmHg (13.33 kPa), but the curve continues to rise because of the dissolved oxygen in plasma, so the whole blood and plasma lines are parallel above a PO2 of 100. The line for perflubron is similar to plasma, but has a higher slope because of the greater affinity for oxygen (Hct ¼ hemocrit).
oxygen release to occur. The potential contribution of PFCs to oxygen transport can be assessed by looking at the oxygen consumption required by tissues, the Fct and the PO2 required to allow this quantity of oxygen to be released in the tissues (28).
Mixed venous blood has an oxygen content of 15 mL/ 100 mL; therefore 5 mL/100 mL of oxygen is consumed in the periphery.
If we assume a mixed venous oxygen tension (PvO2) level of 40 mmHg (5.33 kPa) and an oxygen extraction of 5 mL/dL, a bloodless animal could survive with a Fct of 45% with a PaO2 of 235 mmHg (31.33 kPa). Any increase in PaO2 above this value would raise the PvO2 by the same amount (Fig. 2). The elevated PvO2 in these circumstances could have the beneficial effect of increasing the pressure gradient for oxygen diffusion from the vascular space into the tissues and cells, theoretically increasing tissue oxygenation.
It is, however, difficult to manufacture a 45% emulsion of PFC. In the late 1970s, the Green Cross Corporation in Japan developed a product called Fluosol DA 20%. This solution contains only 10% PFC (Fluosol DA 20% is 20% by weight, 10% by volume). To supply 5 mL/100 mL of oxygen consumption and a PvO2 of 40 mmHg (5.33 kPa), a bloodless animal with a fluocrit of 10% would require a PaO2 of 920 mmHg (122.65 kPa).
In practice, this would make it difficult to completely replace the blood with PFC emulsion. Although the emulsion is cleared from the vascular space within 24 h, the long tissue half-life of a PFC in the body (months to years), make it unfeasible to continuously redose the patient.
|
20 |
Blood Hct 45% |
|
|
|
|
|
|
|
2 mL |
|
|
|
5 mL |
15 |
|
|
|
|
C mL/100 |
|
|
|
|
|
|
|
|
|
O |
|
|
|
|
a |
|
|
|
|
|
10 |
|
|
|
|
|
|
45% |
|
|
5 |
Perflubron |
5 mL |
|
|
|
5 mL |
||
|
|
|
|
|
|
|
100 |
200 |
300 |
|
PvO2 |
PO2 |
mmHg |
PaO2 |
|
PvO2 |
PaO2 |
Figure 2. At an arterial PO2 (PaO2) of 300 mmHg (13.33 kPa) blood has an oxygen content of 21 mL/100 mL. If 5 mL/100 mL are extracted the venous PO2 (PvO2) will be < 50 mmHg. A PFC with a Fct of 45% could carry enough oxygen at a PaO2 of 235 to deliver 5 mL and give a similar PvO2. Increasing the PaO2 to 300 mmHg (39.99 kPa), increases the oxygen carried by the PFC so the PvO2 will be > 100 mmHg (13.33 kPa) after delivery of 5 mL O2. (Adapted, with permission, from Woodcock BJ, Tremper KK. Red Blood Cell Substitutes. In: Evers AS, Maze M, editors. Anesthetic Pharmacology, Physiological Principles and Clinical Practice: A Companion to Miller’s Anesthesia. Philidelphia: Churchill Livingstone; 2004.)

|
CF2 |
|
|
|
|
CF2 |
CF2 |
||
CF3 |
|
CF2 |
CF2 |
CF |
CF2 |
||||
|
|
|
|
|
|
|
|
|
|
CF2 |
|
N |
CF2 |
CF |
CF2 |
||||
CF3 |
CF2 |
|
CF3 |
|
|
CF2 |
CF2 |
||
|
(a) |
|
|
|
|
(b) |
|
|
Figure 3. Structures of the constituents of Fluosol DA; perfluorodecalin (a) and perfluortripropylamine (b).
CLINICAL STUDIES WITH PFCS
Fluosol DA
Fluosol DA 20% was developed in the 1970s and was an emulsion composed of two perfluorochemicals; perfluorodecalin, which has emulsion stability and perfluorotripropylamin, which has a shorter half-life (29) (Fig. 3). The surfactant used to maintain this emulsion of Fluosol DA was Pluronic F68, an emulsifier used in other medical products. The product needed to be frozen to maintain stability until used.
Initial clinical studies were in patients who were actively bleeding, required surgery, and refused blood transfusion. A 20-mL kg 1 body weight of Fluosol DA 20% PFC emulsion was transfused preoperatively with a maximum of one additional dose postoperatively (29,30). The patients achieved a maximal fluorocrit of < 3% with an intravascular half-life of 19 h. Subsequent studies confirmed the oxygen-carrying contribution of the PFC, but could not show a beneficial effect on patient outcome (31).
Fluosol DA 20% gained FDA approval, not for use as a red cell substitute but for intracoronary infusion in patients undergoing angioplasty. Fluosol is no longer being manufactured because of low demand.
Perflubron
Second generation PFC emulsions have been developed. One of these, Oxygent, employs perfluoro-octylbromide or perflubron (32) (Fig. 4). This PFC has one bromine replacing fluorine, making it radiopaque. The emulsion (Oxygent: Alliance Pharmaceutical, San Diego, CA) contains 60% PFC by weight, or 30% by volume. It is emulsified with lecithin (egg yolk phospholipids) and is stable at room temperature for > 6 years.
Because of a short intravascular half-life and the need for a high FiO2, it has been suggested that these PFC solutions should be used in conjunction with acute normovolemic hemodilution. In this setting, patients who are expected to have significant surgical blood loss should have two to four units of blood removed immediately before surgery. Initial volume expansion is with crystalloid or
CF3 |
CF2 |
|
CF2 |
CF2 |
|
CF2 |
CF2 |
CF2 |
CF2Br |
Figure 4. Structure of perfluoro-octylbromide (Perflubron, Oxygent).
BLOOD, ARTIFICIAL |
515 |
colloid. As surgical bleeding continues, the PFC is administered at the first transfusion trigger, allowing for adequate oxygen delivery at low hemoglobin levels. When surgical bleeding stops, the patient would receive the previously harvested autologous blood.
The European Perflubron Emulsion Study Group looked at this technique of normovolemic hemodilution in orthopedic surgery. Perflubron was administered in response to transfusion triggers of tachycardia, hypotension, increased cardiac, and decreased mixed venous PO2. The triggers for transfusion were at least as effectively reversed by perflubron, with 100% oxygen ventilation, as by autologous transfusion or colloid administration (33). Patients who received perflubron had higher mixed venous PO2 levels, which continued for longer than the other treatment groups. Allogeneic blood transfusion requirements were not reduced by perflubron administration.
A phase III cardiac surgery study of perflubron was voluntarily suspended in 2001 because of an increased incidence of stroke, but it has not been determined if this was due to PFC administration.
A phase III study of perflubron in noncardiac surgery, again from the European Perflubron Emulsion Study Group (34), in 492 patients showed an intraoperative reduction in blood transfusion requirement, but there was no significant reduction by time of the postoperative period. Of the patients in the intent-to-treat population, 33% experienced low (< 20 mL kg 1) blood loss. When these patients were excluded to leave a protocol defined target population, the patients treated by acute normovolemic hemodilution (ANH) and PFC administration experienced a reduction in transfusion that remained significant throughout the study (3.4 vs. 4.9 units). The PFC group also had a significantly greater avoidance of transfusion (26 vs. 16%). The clinical relevance of these findings is hard to elucidate. The control group did not undergo ANH and had higher transfusion triggers during the operative period, it is difficult to tell if the benefit accrued was due to the PFC or the hemodilution technique (35).
Microcirculation and Oxygen-Carrying Colloids
Oxygen-carrying colloids differ from red cells in terms of size. Free hemoglobin molecules in solution range in size from 68,000 to 500,000 Da, and the fluorochemical emulsion particles are 0.2 mm. These sizes are within the range of many of the plasma proteins, and like plasma proteins, PFCs are able to cross the capillary basement membrane and participate in extravascular circulation. Animal data have shown that these oxygen-carrying colloids leave the intravascular space and rejoin it through lymphatic circulation (36). Thus, these colloids may be able to provide increased oxygen delivery to the extravascular space (37,38). In addition, studies on microcirculation have shown that plasma flow continues through capillaries even when the capillary is closed to red blood cells. Oxygen delivery to tissues can be provided, even though red cells are not present in the capillaries, through the circulation of oxygen-carrying colloids.
This potential application of blood substitutes, as ‘‘therapeutic oxygenating agents’’ for ischemic tissue, has been
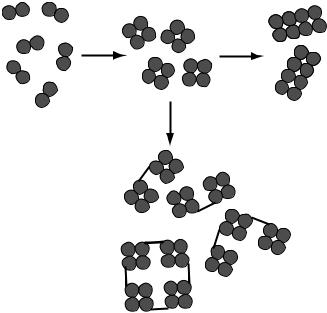
516 BLOOD, ARTIFICIAL
investigated for myocardial ischemia (39–41) and for enhancing the effectiveness of radiation therapy and chemotherapy of ischemic tumors by rendering the tumor hyperoxic (42,43).
Perfluourochemicals have been used in other circumstances as oxygen-carrying molecules. The PFCs have been used for cardiplegia (44) and preservation of transplanted organs (45–47). The PFCs have also been studied in models of myocardial and cerebral infarcts to minimize infarct size (45,48,49).
Liquid Ventilation with PFC
Perfluorochemicals have been used for liquid ventilation in the treatment of acute respiratory distress syndrome (ARDS). The PFCs bind oxygen and carbon dioxide avidly. Perflubron (LiquiVent, Alliance Pharmaceutical Corp., San Diego, CA) can be instilled into the endotracheal tube of a ventilated patient with ARDS until a fluid level is seen outside the patient. The ET is then connected to the normal ICU ventilator and sufficient gas transfer occurs across the PFC–gas interface to allow oxygenation of the patient and CO2 clearance (50,51). This PFC has excellent surfactant properties and is possibly able to stent open alveoli (leading it to be termed ‘‘liquid PEEP’’ or ‘‘PEEP in a bottle’’) (52). There are also benefits of increased secretion clearance and possible antiinflammatory effects (53,54). Studies to date have not shown any benefit over conventional ventilation (55).
Future of PFCs
The PFCs have inherent limitations of a short endovascular half-life and the requirement for high inspired oxygen. These problems limit the use of PFC emulsions to acute settings in which supplemental oxygen is readily available. It is yet to be seen whether PFCs can have a useful role as blood substitutes.
HEMOGLOBIN SOLUTIONS
Hemoglobin Solutions: Oxygen Content
When a solution of free hemoglobin (FHb) has the same P50 as blood (27 mmHg 3.59 kPa), there is no difference between the hemoglobin solution oxygen-content curve and the curve for normal whole blood.
CaO2 ¼ ðFHb 1:34 FSaO2Þ
þ ðHb 1:34 SaO2Þ þ ð0:003 PaO2Þ ð3Þ
[FHb is the concentration of free hemoglobin solution in blood (g dL 1), and FSaO2, is the saturation of FHb.]
In clinical practice, arterial content of oxygen can be calculated using a single term for hemoglobin and saturation. A spectrophotometrically measured total hemoglobin should be used, which will measure total hemoglobin present, whereas the hematocrit only measures red blood cell hemoglobin. The saturation measured by an oximeter gives a mean saturation of both forms of hemoglobin because the device measures the amount of oxyhemoglobin and divides it by total hemoglobin to achieve the calculated saturation.
Formulation of Hemoglobin Solutions
A solution of hemoglobin from lyzed human red cells is unusable as a blood substitute for a variety of reasons. Hemoglobin outside the red cell membrane loses its tetrameric form and breaks down into dimers. The abundance of dimers in plasma has a pronounced oncotic effect creating an excessively high colloid oncotic pressure (41). This would draw fluid from the extracellular space and would cause an increase in circulating blood volume. The dimers have a molecular weight of 32,000 Da and are able to cross the renal glomerular basement membrane leading to a potent osmotic diuretic effect. The loss of oxygenated hemoglobin in the urine gave the early solutions the reputation of being ‘‘red mannitol’’.
The loss of 2,3-DPG, which is normally maintained inside the RBC, reduces the P50 of hemoglobin to 12–14 mmHg (1.59–1.86 kPa) from a normal level of 26. This shifts the oxygen dissociation curve markedly to the left, meaning that the free hemoglobin will avidly bind oxygen during passage through the lungs but will not release it in the peripheral tissues unless the PO2 is extremely low.
The early attempts at making a hemoglobin solution used resuspended hemoglobin filtered from lyzed, outdated, human blood. This solution caused a high incidence of renal failure, which proved not to be due to the free hemoglobin, but due to the ‘‘stroma’’ of residual red blood cell elements left after cell lysis (56).
Several types of hemoglobin solution have been developed, and have taken different approaches to these problems. One product is produced from modified polymerized bovine hemoglobin (Hemopure, HBOC-201, Biopure, Cambridge, USA) (57,58). The dimer form is polymerized to form a larger roughly octomeric molecules (Fig. 5). It is
(a) |
(b) |
(c)
Figure 5. Hemoglobin dimer subunits can be polymerized (a) to form tetramers. These can be further polymerized (b) to form octamers or larger units, or can be cross-linked (c) to increase molecular size.
|
|
|
BLOOD, ARTIFICIAL |
517 |
Table 2. Hemoglobin Solutions in Clinical Trials |
|
|
|
|
|
|
|
|
|
Product (Manufacturer) |
Configuration |
P50 |
Status |
|
|
|
|
|
|
|
Bovine Hemoglobins |
|
|
|
HBOC201 Hemopure (Biopure) |
Polymerized with |
34 mmHg |
Licensed in South Africa and |
|
|
glutaraldehyde |
|
for veterinary use. FDA review; |
|
|
|
|
further studies required |
|
PEG-hemoglobin (Enzon) |
PEG (polyethylene |
20 mmHg |
Phase 1 melanoma studies |
|
|
glycol) conjugated |
|
completed Now discontinued |
|
|
noncross-linked, |
|
|
|
|
encapsulated |
|
|
|
|
HumanHemoglobins |
|
|
|
Polyheme (Northfield) |
Polymerized, |
30 mmHg |
Phase 3 study, before |
|
|
tetramer-free |
|
FDA review |
|
Hemolink (Hemosol) |
Polymerized with |
32 mmHg |
Under review United |
|
|
o-raffinose |
|
Kingdom and Canada |
|
DCLHb (Baxter) |
Cross-linked with |
32 mmHg |
Discontinued |
|
|
diaspirin |
|
|
|
PHP (Curacyte) |
Pyridoxylated |
|
Studied in SIRS |
|
|
Recombinant Hemoglobins |
|
|
|
Optro (Somatogen) |
Genetically fused |
17 mmHg |
Discontinued |
|
|
|
|
|
|
then filtered to remove the smaller molecular weight tetrameric hemoglobin molecules. This reduces the oncotic pressure and prevents passage of the molecule though the glomerulus into the urine. Polymerization also increases the P50 into the normal range and allows the hemoglobin solution to release oxygen in the tissues (41).
Other products have been developed from outdated human blood: Cross-linking hemoglobin molecules with pyridoxal-5-phosphate (Fig. 5), which acts as an artificial 2, 3-DPG, can increase the P50. This technique is used in PolyHeme polymerized hemoglobin solution (Northfield Pharmaceutical, Chicago, IL) (59–61), and pyridoxylated hemoglobin polyoxyethylene conjugate or PHP hemoglobin (62). Diaspirin Cross-Linked Hemoglobin, DCLHb (Baxter Healthcare, Chicago, IL) (63,64) and Hemolink (Hemosol, Toronto, Ontario, Canada) also have a P50 within the normal range and have an increased size due to crosslinking or polymerization.
The size of the hemoglobin molecule can also be increased by attaching the dimer to a large non-hemoglobin molecule, for example, polyethylene glycol used in PEG– hemoglobin (Enzon, Piscataway, NJ). A final method of increasing molecular size is to encapsulate hemoglobin in phospholipid vesicles or liposomes (65).
Hemoglobin cannot only be obtained from outdated human blood, but also from bovine blood. There is a tremendous supply of bovine blood, since nearly 1 million units/day are produced as a byproduct of meat production. Bovine hemoglobin does not normally require 2, 3-DPG, and maintains a P50 in the range of 32 mmHg (4.26 kPa), even as a dimer (66). Concern over the spread of BSE or variant vCJD may impact the development of bovine products (67). The FDA has restricted the import of bovine products from Europe because of the outbreak of Mad Cow disease there (68).
Recombinant hemoglobin has been used as an alternative source of hemoglobin. Optro (Somatogen), was engineered to have a P50 in the normal range. However, manufacture has been discontinued (67).
The hemoglobin solutions that have undergone clinical trial are listed in Table 2. Since these solutions are produced from different hemoglobin sources, and have different sizes and different P50 values, each needs to be evaluated as a separate drug with its own effectiveness and toxicity profile.
All of these products have a relatively short intravascular half-life compared to hemoglobin contained in red blood cells, and are cleared from the vascular space by the reticuloendothelial system with a half-life of24 h.
Hemodynamic Effects of Hemoglobin Solutions
Pulmonary and systemic hypertension have been observed in studies in animals (63,69–72) and human clinical studies (36,38,57,64,73–78). The cause of this appears to be related to the nitric oxide (NO) scavenging properties of hemoglobin. Endothelium derived relaxant factor (EDRF) was identified as NO in the 1990s and its role in controlling vascular resistance was elucidated. Nitric oxide is produced in the endothelial cells of blood vessel walls, and produces smooth muscle relaxation, thereby causing vasodilatation. As blood flow increases, nitric oxide is carried away, reducing its concentration and causing vasoconstriction (79). Binding of nitric oxide to hemoglobin plays an important role in its removal, and thereby the control of vascular tone. Free hemoglobin binds nitric oxide more avidly than hemoglobin within the red cell and nitric oxide clearance is increased (80). This leads to hypertension in the pulmonary and systemic vascular beds. Smaller hemo-
518 BLOOD, ARTIFICIAL
globin molecule size appears to increase NO clearance, and pulmonary hypertension becomes more problematic. The severity of this side effect varies with the different forms and preparations of hemoglobin.
The observed vasoconstriction with hemoglobin solutions led to the evaluation of DCLHb solution as an ‘‘all- in-one’’ therapeutic strategy in vasodilated shock states (38) as a vasopressor in critically ill patients with septic shock. In septic shock or systemic inflammatory response syndrome, the vasodilated state may be due to excessive synthesis of NO. The scavenging effect of the hemoglobin solution may be beneficial in restoring vascular tone in this setting (37). Tissue perfusion may be improved because of the small size of the hemoglobin molecules compared to red cells, improving oxygen delivery through the microcirculation. In a mouse model of gram-negative sepsis, hemoglobin solution was associated with increased circulating tumor necrosis factor and increased lethality (81). In pigs with septic shock, DCLHb administration restored blood pressure and allowed a reduction in dopamine infusion being used for resuscitation (82). Further work is required to determine the utility of hemoglobin solutions in critical illness (83).
Although NO scavenging may play a major role in the vasoconstriction seen with hemoglobin solutions it may not explain the whole picture. Hemoglobin molecules with similar rates of NO binding may have strikingly different degrees of vasoconstriction. The fall in PO2 in terminal arterioles may play a large role in the autoregulation of microvascular circulation by causing vasoldilation. Hemoglobin solutions may deliver excess oxygen to the terminal capillaries causing vasoconstriction and paradoxically reducing oxygen delivery to the tissue (84).
This proposed effect on microcirculation has led to the adoption of a ‘‘counterintuitive’’ approach to hemoglobin solution development. An anemic but hyperoncotic solution with a very low P50 could delay oxygen release and prevent vasoconstriction (85). Such a solution has been developed in MalPEG-Hb (maleimide-activated polyethylene glycol conjugated hemoglobin). This MalPEG-Hb solution has a P50 of 5.5 mmHg and has been shown to improve microvascular circulation in hypovolemic shock in hamsters (86).
Duration of Action of Hemoglobin Solutions
Just as free hemoglobin is scavenged following intravascular hemolysis, the RE system scavenges hemoglobin solution from the blood stream. The effective intravascular life of the hemoglobin solution is 12–48 h, depending on the dose (66). Therefore, hemoglobin solutions, like the PFCs, will not have a role in maintaining oxygen-carrying capacity in chronically anemic patients. They may be used in acute, limited blood-loss situations as resuscitative fluids, especially in combination with perioperative autologous hemodilution to minimize loss of the patients own blood (87,88).
Hematopoietic Effect of Hemoglobin Solutions
Hemoglobin solutions may have a profound effect in stimulating erythropoiesis. Studies in which animals are hemodiluted to a low Hct, and given a transfusion of
hemoglobin solution, demonstrate a pronounced level of red blood cell production exceeding that expected even with the administration of exogenous erythropoietin (36).
Free iron liberated when hemoglobin solutions are broken may stimulate erythropoiesis, reducing the requirement for subsequent red cell transfusion (89,90). Transfusion of diaspirin cross-linked hemoglobin (DCLHb) to transfuse cardiac surgery patients in the postbypass period resulted in 19% of patients not requiring packed red blood cell transfusion, although the DCLHb was rapidly cleared from the circulation (77). After acute normovolemic hemodilution (ANH) with HBOC-201 in human volunteers there were increases in serum iron, ferritin, and erythropoietin that did not occur following ANH with Ringer’s lactate solution (91).
Other Uses of Hemoglobin Solutions
Hemoglobin solutions have an oxygen delivery curve similar to that of hemoglobin in red blood cells. At low temperatures the curve is shifted to the left, preventing release of the bound oxygen, they therefore may not be of any benefit as a constituent of cardioplegia solutions or organ preservatives used for transplanted organs.
Artifical oxygen carriers have a potential for abuse by elite athletes, and international sporting federations have already added the class of agent to their banned substance lists (92).
Clinical Trials: Hemoglobin Solutions
HBOC-201 (Hemopure). The bovine product HBOC-201 (Hemopure, Biopure, Cambridge, MA) is produced from modified polymerized bovine hemoglobin and has been studied in patients undergoing abdominal aortic aneurysm surgery. Treatment with the hemoglobin solution produced an increase in pulmonary and systemic vascular resistance and an associated decrease in cardiac output (57). In another study in aortic surgery HBOC-201, 27% of patients did not need blood transfusion, but the median transfusion requirement was not decreased. Mean arterial blood pressure increased by 15% in this study (75). Vasoconstriction and hypertension have been noted in many studies with HBOC-201. A study looking at preoperative hemodilution with bovine HBOC-201 before liver resection showed an increase in systemic vascular resistance and a fall in cardiac output (73). More recently, a study by Wahr et al. (36) found this product to be useful in reducing the amount of allogeneic blood in operative patients. Increases in pulmonary or systemic resistance were not seen, but a mild increase in blood pressure occurred.
In postoperative cardiac surgery patients, administration of up to 1000 mL HBOC-201 prevented packed red blood cell administration in 34% of patients who would otherwise have received it (93). Although the HBOC-201 had a short duration in the circulation, Hct was restored rapidly, perhaps due to a hematinic effect. The HBOC-201 patients had a slightly greater increase in blood pressure after transfusion.
HBOC-201 has been administered to patients with sickle cell anemia and may have a role in the treatment of vasoocclusive or aplastic crises in this disease (94,95).
The HBOC-201 can be used instead of PRBCs to transfuse patients with severe autoimmune hemolytic anemia, the hemoglobin solution does not have the surface antigens associated with red blood cells (96). In the presence of a Hct of 4.4% the hemoglobin solution reversed both lactic acidosis and myocardial ischemia.
In South Africa, HBOC-201 (Hemopure) has been licensed to treat anemia in surgical patients; this is the first time a hemoglobin solution has reached the market in humans. It is also licensed, as Oxyglobin, for veterinary use in the United States and Europe. The FDA approval for human use in the United States has been delayed pending a request for further information and animal testing.
Diaspirin Cross-Linked Hemoglobin, DCLHb
The DCLHb (Baxter Healthcare, Chicago, IL) has been developed from outdated human blood. Clinical studies to date have had varying results with DCLHb.
The DCLHb effectively scavenges NO and has been noted to increase pulmonary and systemic vascular resistance in the hemodilution model in animals to the point of reducing cardiac index and oxygen delivery relative controls (63). Pulmonary and systemic hypertension with rises in SVR and PVR also occurred in human studies of DCLHb given after cardiac surgery, and this led to decreased cardiac output compared to patients given red cell transfusion (77). Another study (76) showed that DCLHb could be used to reduce the number of patients requiring perioperative packed red blood cell (PRBC) transfusions following vascular, orthopedic, and abdominal surgery compared with patients randomized to PRBC transfusions. However, the total PRBC and other blood product requirements of the two groups were similar over the subsequent week. Side effects of hypertension, jaundice and hemoglobinuria were noted. One death from respiratory distress syndrome was attributed to DCLHb, and the study was terminated early.
This solution was also investigated in a randomized study of patients with acute ischemic stroke (64). Patients receiving the hemoglobin solution had more deaths, serious adverse outcomes, and worse outcome scale scores.
Studies in patients with hemorrhagic shock in the United States and Europe were discontinued because of increased mortality in the U.S. study, and a lack of benefit with DCLHb administration in the European study (97).
Because of these results Baxter has discontinued further work with DCLHb. He subsequently acquired Somatogen, manufacturer of Optro, a first generation recombinant hemoglobin, development of which has since been discontinued.
PolyHeme
PolyHeme (Northfield Pharmaceutical, Chicago, IL) has been used in trials treating acute trauma patients. Gould et al. administered 1–20 units of PolyHeme to 171 patients with urgent blood loss; 81 patients received 5 or more units and 34 received 10–20 units (98). Overall there was a mortality of 10.5 compared to 16% of historical controls who declined blood transfusion because of religious reasons during surgery, and had Hb levels < 8 g/dL. However, there was no comparison with controls receiving transfusion of
BLOOD, ARTIFICIAL |
519 |
allogeneic packed red blood cells. An earlier study, also by Gould, randomly assigned 44 trauma patients to either receive blood (23 patients) or up to 6 units of PolyHeme (21 patients) (60). There were no adverse effects on pulmonary and systemic vascular resistance or cardiac output from the administration of PolyHeme. There were no differences in patient outcomes, although there was a reduced need for red blood cells in the PolyHeme group at day 1, which was no longer seen by day 3. The lack of effect of PolyHeme on systemic and peripheral vascular resistance is attributed to its manufacturing process, which filters out the smaller tetrameric hemoglobin (59,60). It is speculated that the smaller size hemoglobin elements can defuse through the vessel wall, increasing NO scavenging and producing vasoconstriction.
Studies have found an intravascular half-life of 24 h of PolyHeme, which is longer than that found in previous studies that found a halflife in the range of 9–12 h. It may be that the half-life of these products is dose dependent; studies showing a larger dose produces a longer half-life.
PolyHeme is currently being assessed in a large multicenter study compared to saline for trauma patients. An application to the FDA for approval may follow this study and PolyHeme could have a role in future clinical practice.
Hemolink
Hemolink (Hemosol, Toronto, Ontario, Canada) is an O-raffinose cross-linked human hemoglobin, which has been shown to cause vasoconstriction in animals (70). As with other cross-linked hemoglobin solutions, the hemodynamic effects are less pronounced than with unmodified hemoglobin solutions (99) but exceed that of polymerized hemoglobin solutions.
A Phase I study in healthy human volunteers showed the solution was well tolerated apart from some moderate to severe abdominal pain, which occurred in all subjects at higher doses. Blood pressure rose by 14% following administration, and with higher doses this elevation lasted 24 h (78). The findings of a Phase II study in coronary artery bypass (CAB) surgery (74) reported a 7–10% increase in blood pressure, which was not statistically significant, and a reduction in the number of patients requiring red cell transfusion from 57 to 10%. A further report by the same group using intraoperative autologous donation and volume replacement with Hemolink or pentastarch in CAB surgery abolished the need for intraoperative transfusion (0 vs. 17% in the pentastarch group) (100). The reduction in transfusion requirement continued at 1 day (7 vs. 37%) and 5 days (10 vs. 47 %) after surgery. Adverse effects included hypertension (43 vs. 17%) and atrial fibrillation (37 vs. 17%).
A similar study in CAB patients using intraoperative autologous blood donation (IAD) and volume replacement with Hemolink or pentastarch showed a reduction in transfusion from 76% in the pentastarch group to 56% with Hemolink (101). This was compared to an historical group of patients in whom IAD was not used, who required transfusion in 95% of operations. Hypertension was again noted in the Hemolink treated group.
520 BLOOD, ARTIFICIAL
Hemolink is under review for approval by the drug agencies of the United States, Canada, and the United Kingdom.
PEG Hemoglobin
The PEG Hemoglobin (Enzon, Piscataway, NJ) is a bovine hemoglobin conjugated with polyethylene glycol. This increases the molecular size without using cross-linking between molecules. Retention in the circulation is increased as the conjugated molecule does not cross the glomerular basement membrane. Administration in dogs resulted in no elevation of blood pressure and it was well tolerated (102).
The PEG hemoglobin has been used to sensitize tumors to chemotherapy (103) and radiotherapy in rodents (104). The small molecular size, compared to red cells, improves microvascular oxygenation, and tumors that are hypoxic become more responsive to chemotherapy and radiotherapy.
The PEG hemoglobin has also been used in rabbits, in the preservative perfusate, and for protection of transplanted hearts during the ischemic period with improvement in cardiac function post-transplant (105).
PHP
Pyridoxilated hemoglobin polyoxyethylene conjugate or PHP (Curacyte, Chapel Hill, NC) is a human hemoglobin solution, cross-linked by pyridoxal-5-phosphate, which is being developed as a NO scavenger for use in septic shock and systemic inflammatory response syndrome (62). A Phase II study has been completed and a Phase III study is in progress looking at PHP for the treatment of NO induced shock.
Encapsulated Hemoglobins
The problems of small hemoglobin molecule size, leading to NO scavenging, high oncotic pressures, and osmotic diuresis can be countered by encapsulating the hemoglobin. This mimics the natural presentation of hemoglobin in whole blood and is sometimes referred to as ‘‘neo red cells’’ (65). Most work has used liposome encapsulated hemoglobin (LEH), but biodegradable polymer microcapsules have also been used (106). Circulation time of the hemoglobin is increased by encapsulation and can be increased, from 18 to 65 h, by polyethylene glycol (PEG) modification (107), these long-lasting derivatives have been named ‘‘stealth’’ liposomes. However, there is significant accumulation of liposomes in the liver and spleen, when LEH is given, causing vacuolization seen on liver biopsy. Liver transaminases may also be elevated (41).
BIBLIOGRAPHY
Cited References
1.Epstein JS. The US blood supply. Am Fam Phys 2000;61: 549–550.
2.American Society of Anesthesiologists Task Force on Blood Component Therapy, Practice Guidelines for blood component therapy. Anesthesiology 1996;84:732–747.
3.Spahn DR, Casutt M. Eliminating blood transfusions: new aspects and perspectives. Anesthesiology 2000;93:242–255.
4.Cohn SM. Blood substitutes in surgery. Surgery 2000;127: 599–602.
5.Ketcham EM, Cairns CB. Hemoglobin-based oxygen carriers: development and clinical potential. Ann Emerg Med 1999; 33:326–337.
6.Myhre BA, Bove JR, Schmidt PJ. Wrong blood—a needless cause of surgical deaths. Anest Analg 1981;60:777–778.
7.Nichollis MD. Transfusions: morbidity and mortality. Anaesth Intensive Care 1993; 15–19.
8.Sazama K. Reports of 355 transfusion-associated deaths: 1976 through 1985. Transfusion (Paris) 1990;30:583–590.
9.Newman RJ, Podolsky D, Loeb P. Bad blood. US News World Rep 1994;116:68–70.
10.Lackritz EM. Prevention of hiv transmission by blood transfusion in the developing world: achievements and continuing challenges. AIDS 1998;12:81–86.
11.Chamberland M, Khabbaz RF. Emerging issues in blood safety. Inf Dis Clin North Am 1998;12:217–229.
12.Dodd RY. The risk of transfusion-transmitted infection. N Engl J Med 1992;327:419–421.
13.Yoshikawa A, Fukuda S, Itoh K, Kosaki N, Suzuki T, Hirakawa K, Nakao H, Inoue T, Fukuda M, Okamoto H. Infection with hepatitis g virus and its strain variant, the gb agent (gbv-c), among blood donors in japan. Transfusion (Paris) 1997;37:657–663.
14.Tacke M, Kiyosawa K, Stark K, Schlueter V, Ofenloch-Haehnle B, Hess G, Engel AM. Detection of antibodies to a putative hepatitis g virus envelope protein. Lancet 1997;349:318–320.
15.Fiebig EW, Busch MP. Emerging infections in transfusion medicine. Clin Lab Med 2004;24:797.
16.Alter MJ, Gallagher M, Morris TT, Moyer LA, Meeks EL, Krawczynski K, Kim JP, Margolis HS. Acute non-a-e hepa-
titis in the united states and the role of hepatitis g virus infection. Sentinel counties viral hepatitis study team. N Engl J Med 1997;336:741–746.
17.Alter HJ, Nakatsuji Y, Melpolder J, Wages J, Wesley R, Shih JW, Kim JP. The incidence of transfusion-associated hepatitis g virus infection and its relation to liver disease. N Engl J Med 1997;336:747–754.
18.Karayiannis P, Thomas HC. Current status of hepatitis g virus (gbv-c) in transfusion: is it relevant? Vox Sang 1997;73:63–69.
19.Ricketts MN, Cashman NR, Stratton EE, ElSaadany S. Is creutzfeldt-jakob disease transmitted in blood? Emerg Infect Dis 1997;3:155–163.
20.Houston F, Foster JD, Chong A, Hunter N, Bostock CJ. Transmission of bse by blood transfusion in sheep. Lancet 2000;356:999–1000.
21.Mitka M. Blood groups differ on donor deferral. JAMA 2001;285:1694–1695.
22.Iwamoto M, Jernigan DB, Guasch A, Trepka MJ, Blackmore CG, Hellinger WC, Pham SM, Zaki S, Lanciotti RS, LanceParker SE, DiazGranados CA, Winquist AG, Perlino CA, Wiersma S, Hillyer KL, Goodman JL, Marfin AA, Chamberland ME, Petersen LR. West Nile Virus in Transplant Recipients Investigation Team, Transmission of west nile virus from an organ donor to four transplant recipients. N Engl J Med 2003;348:2196–203.
23.Blumberg N, Triulzi DJ, Heal JM. Transfusion-induced immunomodulation and its clinical consequences. Transfus Med Rev 1990;4:24–35.
24.Bordin JO, Blajchman MA. Immunosuppressive effects of allogeneic blood transfusions: implications for the patient with a malignancy. Hematol Oncol Clin North Am 1995;9: 205–218.
25.Biedler AE, Schneider SO, Seyfert U, Rensing H, Grenner S, Girndt M, Bauer I, Bauer M. Impact of alloantigens and
storage-associated factors on stimulated cytokine response in an in vitro model of blood transfusion. Anesthesiology 2002;97:1102–1109.
26.Clark Jr LC, Gollan F. Survival of mammals breathing organic liquids equilibrated with oxygen at atmospheric pressure. Science 1966;152:1755–1766.
27.Gehes RP, Monroe RG, Taylor K. Survival of rats having red cells totally replaced with emulsified fluorocarbon. Fed Pro 1968;27:384.
28.Woodcock BJ, Tremper KK. Red Blood Cell Substitutes. In: Evers AS, Maze M, editors. Anesthetic Pharmacology: Physiological Principles and Clinical Practice: A Companion to Miller’s Anesthesia. Philidelphia: Churchill Livingstone; 2004.
29.Tremper KK, Friedman AE, Levine EM, Lapin R, Camarillo D. The preoperative treatment of severely anemic patients with a perfluorochemical oxygen-transport fluid, Fluosol-DA. N Engl J Med 1982;307:277–283.
30.Tremper KK, Levine EM, Waxman K. Clinical experience with Fluosol-DA (20%) in the United States. Int Anesthesiol Clinic 1985;23:185–197.
31.Gould SA, Rosen AL, Sehgal LR, Sehgal HL, Langdale LA, Krause LM, Rice CL, Chamberlin WH, Moss GS. Fluosol-DA as a red-cell substitute in acute anemia. N Engl J Med 1986;314:1653–1656.
32.Keipert PE, Faithfull NS, Bradley JD, Hazard DY, Hogan J, Levisetti MS, Peters RM. Oxygen delivery augmentation by low-dose perfluorochemical emulsion during profound normovolemic hemodilution. Adv Exp Med Biol 1994;345: 197–204.
33.Spahn DR, van Brempt R, Theilmeier G, Reibold JP, Welte M, Heinzerling H, Birck KM, Keipert PE, Messmer K, Heinzerling H, Birck KM, Keipert PE, Messmer K. Perflubron emulsion delays blood transfusions in orthopedic surgery. European perflubron emulsion study group. Anesthesiology 1999;91:1195–208.
34.Spahn DR, Waschke KF, Standl T, Motsch J, Van Huynegem L, Welte M, Gombotz H, Coriat P, Verkh L, Faithfull S, Keipert P. Use of perflubron emulsion to decrease allogeneic blood transfusion in high-blood-loss non-cardiac surgery: results of a European phase 3 study. Anesthesiology 2002;97:1338–1349.
35.Tremper KK. Perfluorochemical ‘‘red blood cell substitutes’’: the continued search for an indication. Anesthesiology 2002;97:1333–1334.
36.Wahr JA, Levy JH, Kindscher J. Hemodynamic effects of a bovine based oxygen carrying solution in surgical patients. Anesthesiology 1996;85:A347.
37.Creteur J, Vincent JL. Hemoglobin solutions: an ‘‘all-in-one’’ therapeutic strategy in sepsis? Crit Care Med 2000;28: 894–896.
38.Reah G, Bodenham AR, Mallick A, Daily EK, Przybelski RJ. Initial evaluation of diaspirin cross-linked hemoglobin (DCLHB) as a vasopressor in critically ill patients. Crit Care Med 1997;25:1480–1488.
39.Robalino BD, Marwick T, Lafont A, Vaska K, Whitlow PL. Protection against ischemia during prolonged balloon inflation by distal coronary perfusion with use of an autoperfusion catheter or Fluosol. J Am Coll Cardiol 1992;20:1378– 1384.
40.Kent KM, Cleman MW, Cowley MJ, Forman MB, Jaffe CC, Kaplan M, King SB 3rd, Krucoff MW, Lassar T, McAuley B. et al. Reduction of myocardial ischemia during percutaneous transluminal coronary angioplasty with oxygenated Fluosol. Am J Cardiol 1990;66:279–284.
41.Creteur J, Sibbald W, Vincent JL. Hemoglobin solutions– not just red blood cell substitutes. Crit Care Med 2000;28: 3025–3034.
BLOOD, ARTIFICIAL |
521 |
42.Teicher BA, Schwartz GN, Dupuis NP, Kusomoto T, Liu M, Liu F, Northey D. Oxygenation of human tumor xenografts in nude mice by a perfluorochemical emulsion and carbogen breathing. Artif Cells, Blood Sub Immobil Biotechnol 1994; 22:1369–1375.
43.Teicher BA. An overview on oxygen carriers in cancer therapy. Artif Cells, Blood Sub Immobil Biotechnol 1995; 23:395– 405.
44.Martin SM, Laks H, Drinkwater DC, Stein DG, Capouya ER, Pearl JM, Barthel SW, Chang P, Kaczer E, Bhuta S. Perfluorochemical reperfusion yields improved myocardial recovery after global ischemia. Ann Thorac Surg 1993;55: 954–960.
45.Kloner RA, Hale S. Cardiovascular applications of fluorocarbons in regional ischemia/reperfusion. Artif Cells Blood Substit Immobil Biotechnol 1992;22:1069–1081.
46.Segel LD, Follette DM, Iguidbashian JP, Contino JP, Castellanos LM, Berkoff HA, Kaufman RJ, Schweighardt FK. Posttransplantation function of hearts preserved with fluorochemical emulsion. J Heart Lung Trans 1994;13: 669–680.
47.Grunert A, Qiu H, Muller I, Schuh S, Steinbach G, Wennauer R, Wolf C, Von Schenck H. A new extracorporeal perfusion system: prolongation of liver organ vitality beyond 24 hours. Ann N Y Acad Sci 1994;723:488–490.
48.Premaratne S, Harada RN, Chun P, Suehiro A, McNamara
JJ.Effects of perfluorocarbon exchange transfusion on reducing myocardial infarct size in a primate model of ischemiareperfusion injury: a prospective, randomized study. Surgery 1995;117:670–676.
49.Cole DJ, Schell RM, Drummond JC, Pryzbelski RJ, Marcantonio S. Focal cerebral ischemia in rats: effect of hemodilution with alpha-alpha cross-linked hemoglobin on brain injury and edema. Can J Neurol Sci 1993;20:30–36.
50.Gauger PG, Overbeck MC, Chambers SD, Cailipan CI, Hirschl RB. Partial liquid ventilation improves gas exchange and increases EELV in acute lung injury. J Appl Physiol 1998;84:1566–1572.
51.Hirschl RB, Pranikoff T, Wise C, Overbeck MC, Gauger P, Schreiner RJ, Dechert R, Bartlett RH. Initial experience with partial liquid ventilation in adult patients with the acute respiratory distress syndrome. JAMA 1996;275:383–389.
52.Wong DH. Liquid ventilation: more than ‘‘PEEP in a bottle’’? Crit Care Med 1999;27:1052–1053.
53.Colton DM, Till GO, Johnson KJ, Dean SB, Bartlett RH, Hirschl RB. Neutrophil accumulation is reduced during partial liquid ventilation. Crit Care Med 1998;26:1716– 1724.
54.Mrozek JD, Smith KM, Bing DR, Meyers PA, Simonton SC, Connett JE, Mammel MC. Exogenous surfactant and partial liquid ventilation: physiologic and pathologic effects. Am J Resp Crit Care Med 1997;156:1058–1065.
55.Hirschl RB, Conrad S, Kaiser R, Zwischenberger JB, Bartlett RH, Booth F, Cardenas V. Partial liquid ventilation in adult patients with ARDS: a multicenter phase I-II trial. Adult PLV Study Group. Ann Surg 1998;228:692–700.
56.Rabiner SF, Friedman LH. The role of intravascular haemolysis and the reticulo-endothelial system in the production of a hypercoagulable state. Br J Haematol 1968;14: 105–118.
57.Kasper SM, Grune F, Walter M, Amr N, Erasmi H, Buzello
W.The effects of increased doses of bovine hemoglobin on hemodynamics and oxygen transport in patients undergoing preoperative hemodilution for elective abdominal aortic surgery. Anesth Analg 1998;87:284–291.
58.Standl T, Burmeister MA, Horn EP, Wilhelm S, Knoefel WT, Schulte am Esch J. Bovine haemoglobin-based oxygen
522 BLOOD, ARTIFICIAL
carrier for patients undergoing haemodilution before liver resection. Br J Anaesth 1998;80:189–194.
59.Johnson JL, Moore EE, Offner PJ, Haenel JB, Hides GA, Tamura DY. Resuscitation of the injured patient with polymerized stroma-free hemoglobin does not produce systemic or pulmonary hypertension. Am J Surg 1998;176:612–617.
60.Gould SA, Moore EE, Hoyt DB, Burch JM, Haenel JB, Garcia J, DeWoskin R, Moss GS. The first randomized trial of human polymerized hemoglobin as a blood substitute in acute trauma and emergent surgery. J Am Coll Surg 187:113–20; discussion 1998; 120–122.
61.Sehgal LR, Rosen AL, Gould SA, Sehgal HL, Moss GS. Preparation and in vitro characteristics of polymerized pyridoxylated hemoglobin. Transfusion (Paris) 1983;23: 158–162.
62.Privalle C, Talarico T, Keng T, DeAngelo J. Pyridoxalated hemoglobin polyoxyethylene: a nitric oxide scavenger with antioxidant activity for the treatment of nitric oxide-induced shock. Free Rad Biol Med 2000;28:1507–1517.
63.DeAngeles DA, Scott AM, McGrath AM, Korent VA, Rodenkirch LA, Conhaim RL, Harms BA. Resuscitation from hemorrhagic shock with diaspirin cross-linked hemoglobin, blood, or hetastarch. J Trauma-Injury Inf Crit Care 42:406– 412; discussion 1997; 412–414.
64.Saxena R, Wijnhoud AD, Carton H, Hacke W, Kaste M, Przybelski RJ, Stern KN, Koudstaal PJ. Controlled safety study of a hemoglobin-based oxygen carrier, DCLHB, in acute ischemic stroke. Stroke 1999;30:993–996.
65.Rudolph AS. Encapsulated hemoglobin: current issues and future goals. Artif Cells, Blood Sub Immobiliz Biotechnol 1994;22:347–360.
66.Hughes GS Jr., Antal EJ, Locker PK, Francom SF, Adams WJ, Jacobs EE Jr Physiology and pharmacokinetics of a novel hemoglobin-based oxygen carrier in humans. Crit Care Med 1996;24:756–764.
67.Winslow RM. Blood substitutes. Adv Drug Del Rev 2000;40: 131–142.
68.USDA Interim Rule on Import Restrictions of Ruminant Material from Europe. Fed Proc 1998;63:406–408.
69.Ulatowski JA, Nishikawa T, Matheson-Urbaitis B, Bucci E, Traystman RJ, Koehler RC. Regional blood flow alterations after bovine fumaryl beta beta-crosslinked hemoglobin transfusion and nitric oxide synthase inhibition. Crit Care Med 1996;24:558–565.
70.Ning J, Wong LT, Christoff B, Carmichael FJ, Biro GP.
Haemodynamic response following a 10% topload infusion of HemolinkTM in conscious, anaesthetized and treated spontaneously hypertensive rats. Transfus Med 2000;10:13–22.
71.Krieter H, Hagen G, Waschke KF, Kohler A, Wenneis B, Bruckner UB, van Ackern K. Isovolemic hemodilution with a bovine hemoglobin-based oxygen carrier: effects on hemodynamics and oxygen transport in comparison with a non- oxygen-carrying volume substitute.[See comment]. J Cardiothor Vas Anesthes 1997;11:3–9.
72.Maxwell RA, Gibson JB, Fabian TC, Proctor KG. Resuscitation of severe chest trauma with four different hemoglobinbased oxygen-carrying solutions. J Trauma-Injury Inf Crit Care 49:200–209; discussion 2000; 209–211.
73.Standl T, Wilhelm S, Horn EP, Burmeister M, Gundlach M, Schulte am Esch J. Preoperative hemodilution with bovine hemoglobin. Acute hemodynamic effects in liver surgery patients. Anaesthesist 1997;46:763–770.
74.Cheng DC, Ralph-Edwards A, Mazer CD, Carmichael FJL,
Biro GP. The hemodynamic effects of the red cell substitute HemolinkTM (o-rafinose cross-linked human hemoglobin) on vital signs in patients undergoing CABG surgery. Anesthesiology 2000;93:A-180.
75.LaMuraglia GM, O’Hara PJ, Baker WH, Naslund TC, Norris EJ, Li J, Vandermeersch E. The reduction of the allogenic transfusion requirement in aortic surgery with a hemoglo- bin-based solution. J Vasc Surg 2000;31:299–308.
76.Schubert A, Mascha E, O’Hara JF. Synthetic hemoglobin reduces perioperative blood transfusions in vascular, orthopedic and abdominal surgery. Anesthesiology 2000;93:180.
77.Lamy ML, Daily EK, Brichant JF, Larbuisson RP, Demeyere RH, Vandermeersch EA, Lehot JJ, Parsloe MR, Berridge JC, Sinclair CJ, Baron JF, Przybelski RJ. Randomized trial of diaspirin cross-linked hemoglobin solution as an alternative to blood transfusion after cardiac surgery. The DCLHB cardiac surgery trial collaborative group. Anesthesiology 2000;92:646–656.
78.Carmichael FJ, Ali AC, Campbell JA, Langlois SF, Biro GP, Willan AR, Pierce CH, Greenburg AG. A phase I study of oxidized raffinose cross-linked human hemoglobin. Crit Care Med 2000;28:2283–2292.
79.Patel RP. Biochemical aspects of the reaction of hemoglobin and no: implications for hb-based blood substitutes. Free Rad Biol Med 2000;28:1518–1525.
80.Kim HW, Greenberg AG. Ferrous sulphate scavenging of endothelium derived nitric oxide is a principal mechanism for hemoglobin mediated vasoactivities in isolated rat thoracic aorta. Artf Cells, Blood Sub Immobil Biotechnol 1997;25:121–133.
81.Su D, Roth RI, Levin J. Hemoglobin infusion augments the tumor necrosis factor response to bacterial endotoxin (lipopolysaccharide) in mice. Crit Care Med 1999;27:771–778.
82.Freilich E, Freilich D, Hacker M, Leach L, Patel S, Hebert J. The hemodynamic effects of diaspirin cross-linked hemoglobin in dopamine-resistant endotoxic shock in swine. Art Cells, Blood Sub Immobiliz Biotechnol 2002;30:83–98.
83.Zimmerman JJ. Deciphering the dark side of free hemoglobin in sepsis. Crit Care Med 1999;27:685–686.
84.Winslow RM. Current status of blood substitute research: towards a new paradigm. J Intern Med 2003;253:508–517.
85.Kramer GC. Counterintuitive red blood cell substitute– polyethylene glycol-modified human hemoglobin. Crit Care Med 2003;31:1882–1884.
86.Wettstein R, Tsai AG, Erni D, Winslow RM, Intaglietta M. Resuscitation with polyethylene glycol-modified human hemoglobin improves microcirculatory blood flow and tissue oxygenation after hemorrhagic shock in awake hamsters. Crit Care Med 2003;31:1824–1830.
87.Slanetz PJ, Lee R, Page R, Jacobs EE Jr, LaRaia PJ, Vlahakes GJ. Hemoglobin blood substitutes in extended preoperative autologous blood donation: an experimental study. Surgery 1994;115:246–254.
88.Lee R, Neya K, Svizzero TA, Vlahakes GJ. Limitations of the efficacy of hemoglobin-based oxygen-carrying solutions. J Appl Physiol 1995;79:236–242.
89.Vlahakes GJ. Hemoglobin solutions come of age. Anesthesiology 2000;92:637–638.
90.Levy JH. Hemoglobin-based oxygen-carrying solutions: close but still so far. Anesthesiology 2000;92:639–641.
91.Hughes GS Jr, Francome SF, Antal EJ, Adams WJ, Locker PK, Yancey EP, Jacobs EE Jr. Hematologic effects of a novel hemoglobin-based oxygen carrier in normal male and female subjects. J Lab Clin Med 1995;126:444–451.
92.Schumacher YO, Ashenden M. Doping with artificial oxygen carriers: an update. Sports Med 2004;34:141–150.
93.Levy JH, Goodnough LT, Greilich PE, Parr GV, Stewart RW, Gratz I, Wahr J, Williams J, Comunale ME, Doblar D, Silvay G, Cohen M, Jahr JS, Vlahakes GJ. Polymerized bovine hemoglobin solution as a replacement for allogeneic red blood cell transfusion after cardiac surgery: