
- •VOLUME 1
- •CONTRIBUTOR LIST
- •PREFACE
- •LIST OF ARTICLES
- •ABBREVIATIONS AND ACRONYMS
- •CONVERSION FACTORS AND UNIT SYMBOLS
- •ABLATION.
- •ABSORBABLE BIOMATERIALS.
- •ACRYLIC BONE CEMENT.
- •ACTINOTHERAPY.
- •ADOPTIVE IMMUNOTHERAPY.
- •AFFINITY CHROMATOGRAPHY.
- •ALLOYS, SHAPE MEMORY
- •AMBULATORY MONITORING
- •ANALYTICAL METHODS, AUTOMATED
- •ANALYZER, OXYGEN.
- •ANESTHESIA MACHINES
- •ANESTHESIA MONITORING.
- •ANESTHESIA, COMPUTERS IN
- •ANGER CAMERA
- •ANGIOPLASTY.
- •ANORECTAL MANOMETRY
- •ANTIBODIES, MONOCLONAL.
- •APNEA DETECTION.
- •ARRHYTHMIA, TREATMENT.
- •ARRHYTHMIA ANALYSIS, AUTOMATED
- •ARTERIAL TONOMETRY.
- •ARTIFICIAL BLOOD.
- •ARTIFICIAL HEART.
- •ARTIFICIAL HEART VALVE.
- •ARTIFICIAL HIP JOINTS.
- •ARTIFICIAL LARYNX.
- •ARTIFICIAL PANCREAS.
- •ARTERIES, ELASTIC PROPERTIES OF
- •ASSISTIVE DEVICES FOR THE DISABLED.
- •ATOMIC ABSORPTION SPECTROMETRY.
- •AUDIOMETRY
- •BACTERIAL DETECTION SYSTEMS.
- •BALLOON PUMP.
- •BANKED BLOOD.
- •BAROTRAUMA.
- •BARRIER CONTRACEPTIVE DEVICES.
- •BIOCERAMICS.
- •BIOCOMPATIBILITY OF MATERIALS
- •BIOELECTRODES
- •BIOFEEDBACK
- •BIOHEAT TRANSFER
- •BIOIMPEDANCE IN CARDIOVASCULAR MEDICINE
- •BIOINFORMATICS
- •BIOLOGIC THERAPY.
- •BIOMAGNETISM
- •BIOMATERIALS, ABSORBABLE
- •BIOMATERIALS: AN OVERVIEW
- •BIOMATERIALS: BIOCERAMICS
- •BIOMATERIALS: CARBON
- •BIOMATERIALS CORROSION AND WEAR OF
- •BIOMATERIALS FOR DENTISTRY
- •BIOMATERIALS, POLYMERS
- •BIOMATERIALS, SURFACE PROPERTIES OF
- •BIOMATERIALS, TESTING AND STRUCTURAL PROPERTIES OF
- •BIOMATERIALS: TISSUE-ENGINEERING AND SCAFFOLDS
- •BIOMECHANICS OF EXERCISE FITNESS
- •BIOMECHANICS OF JOINTS.
- •BIOMECHANICS OF SCOLIOSIS.
- •BIOMECHANICS OF SKIN.
- •BIOMECHANICS OF THE HUMAN SPINE.
- •BIOMECHANICS OF TOOTH AND JAW.
- •BIOMEDICAL ENGINEERING EDUCATION
- •BIOSURFACE ENGINEERING
- •BIOSENSORS.
- •BIOTELEMETRY
- •BIRTH CONTROL.
- •BLEEDING, GASTROINTESTINAL.
- •BLADDER DYSFUNCTION, NEUROSTIMULATION OF
- •BLIND AND VISUALLY IMPAIRED, ASSISTIVE TECHNOLOGY FOR
- •BLOOD BANKING.
- •BLOOD CELL COUNTERS.
- •BLOOD COLLECTION AND PROCESSING
- •BLOOD FLOW.
- •BLOOD GAS MEASUREMENTS
- •BLOOD PRESSURE MEASUREMENT
- •BLOOD PRESSURE, AUTOMATIC CONTROL OF
- •BLOOD RHEOLOGY
- •BLOOD, ARTIFICIAL
- •BONDING, ENAMEL.
- •BONE AND TEETH, PROPERTIES OF
- •BONE CEMENT, ACRYLIC
- •BONE DENSITY MEASUREMENT
- •BORON NEUTRON CAPTURE THERAPY
- •BRACHYTHERAPY, HIGH DOSAGE RATE
- •BRACHYTHERAPY, INTRAVASCULAR
- •BRAIN ELECTRICAL ACTIVITY.
- •BURN WOUND COVERINGS.
- •BYPASS, CORONARY.
- •BYPASS, CARDIOPULMONARY.
322BIOMATERIALS FOR DENTISTRY
80.Santavirta S, Lappalainen R, Heinonen H, Anttila A. Some relevant issues related to the use of amorphous diamond coatings for medical applications. Diamond Related Mater 1998;7:482–485.
81.Sheeja D, et al. Mechanical and tribological characterization of diamond-like carbon coatings on orthopedic materials. Diamond Related Mater 2001;10:1043–1048.
82.Sheeja D, Tay BK, Lau SP, Nung LN. Tribological characterization of diamond-like carbon coatings on Co-Cr-Mo alloy for orthopaedic applications. Surface Coatings Technol 2001;146: 410–416.
83.Ahlroos T, Saikko V. Wear of prosthetic joint materials in various lubricants. Wear 1997;211:113–119.
84.Saikko V, Ahlroos T. Phospholipids as boundary lubricants in wear tests of prosthetic joint materials. Wear 1997;207:86–91.
85.Affatato S, Frigo M, Toni A. An in vitro investigation of diamond-like carbon as a femoral head coating. J Biomed Mater Res 2000;53:221–226.
86.Dong H, Shi W, Bell T. Potential of improving tribological performance of UHMWPE by engineering the Ti6Al4V counterfaces. Wear 1999;229:146–153.
87.Morshed MM, McNamara BP, Cameron DC, Hashmi MSJ. Effect of surface treatment on the adhesion of DLC film on 316L stainless steel. Surface Coatings Technol 2003;163:541– 545.
88.Schwan J, et al. Stress-induced formation of high-density amorphous carbon thin films. J Appl Phys 1997;82:6024– 6030.
89.Lifshitz Y, et al. Growth mechanisms of DLC films from Cþ ionsexperimental studies. Diamond Related Mater 1995;4: 318–323.
90.Narayan RJ, Scholvin D. Nanostructured carbon-metal composite films. J Vac Sci Technol B 2005;23:1041–1046.
91.Narayan RJ. Pulsed laser deposition of functionally gradient diamondlike carbon-metal nanocomposites. Diamond Related Mater 2005;14(8):1319–1330.
92.Narayan RJ, et al. Antimicrobial properties of diamond-like carbon-silver-platinum nanocomposite thin films. J Mater Eng Perform 2005;14:435–440.
See also BIOMATERIALS, TESTING AND STRUCTURAL PROPERTIES OF;
HEART VALVE PROSTHESES; HIP JOINTS, ARTIFICIAL.
BIOMATERIALS FOR DENTISTRY
STEVE ARMSTRONG
University of Iowa
INTRODUCTION
Gold has been used for dental purposes for at least 2500 years; the fabrication of gold crowns and bridgework flourished in Etruria and Rome as early as 700–500 BC. Gold leaf came into use during the fifteenth century for the restoration of carious teeth. Restorative materials and techniques continued to develop through the nineteenth century including the use of waxes, fused porcelain, ‘‘silver paste’’ amalgam, cements, vulcanite, the angle handpiece, and a gold inlay casting machine. A rapid development in materials and instrumentation has occurred since the 1950s, to include the high speed handpiece, steel and diamond cutting instruments, adhesive techniques to metal, ceramics, enamel and dentin, resin-based compo-
sites, glass ionomers, base-metal alloys for partial dentures, metal-ceramic systems, high-strength all ceramic structures, and titanium alloys for dental implants. This increasing complexity and body of knowledge has lead to the establishment of uniform material standards and the recognition of the science of dental materials as a distinct and essential branch of dentistry.
Biomaterials are used in the oral cavity either to restore function, comfort, or aesthetics caused by developmental disorders, disease, or trauma. More elective procedures are being requested and performed purely for aesthetic purposes as the incidence of caries has dropped in certain population groups and as patients have become more aware of various restorative or cosmetic options. However, the replacement of diseased tooth structure or missing teeth accounts for the bulk of work in restorative dentistry. The instruments and materials used in the surgical aspects of oral, maxillofacial and periodontal surgery have much in common with medicine. This article will focus on those commonly used materials in the restoration of individual teeth or the replacement of missing teeth.
Restorative materials include noble and base metal alloys, resin-based composites (RBCs), glass ionomers, ceramics, acrylics, and amalgam alloys. Techniques to apply these materials include both direct and indirect approaches. Materials or ‘‘fillings’’ can be directly placed in a prepared cavity by the use of adhesives and/or reten- tive-type preparations. Full or partial coverage crowns, bridges, and dentures are fabricated indirectly by dental laboratories or computer aided milling machines and then attached or cemented into the mouth for the coverage of missing or weakened tooth structure or the replacement of missing teeth. Various forms of ceramic or metallic implants can be placed into the upper or lower jaw bones to serve as tooth root substitutes upon which a prosthesis is attached to replace missing teeth. Auxiliary materials, such as waxes, gypsum, and impression materials are also utilized during clinical and laboratory steps but will not be covered in this article.
DIRECTLY PLACED RESTORATIVE MATERIALS: ‘FILLINGS’
Amalgam
Dental amalgam has been very successfully used in dentistry for 150 years and is one of the most technique insensitive dental restorative materials available. Dental amalgams are inexpensive and have demonstrated a relatively long service life. The disadvantages of amalgam are the silver color and the presence of mercury. The presence of mercury requires regulatory control of wastewater effluent and has raised unsubstantiated health concerns regarding mercury toxicity to the individual patient.
Dental amalgam is a mixture of mercury and a solid metal alloy of silver, tin, copper, and sometimes zinc, palladium, indium, and selenium. Once the mercury and alloy is mixed, the plastic mass is condensed into the prepared cavity and carved to required form before hardening. The alloy particles are microspheres of various sizes, irregular lathe-cut particles or mixtures of the two. ‘‘High

copper’’ alloys (13–30%) have essentially replaced the low copper alloys of the past. These high copper alloys, along with the addition of zinc for manufacturing procedures, have improved early clinical strength, lowered creep, and improved corrosion resistance. The mixing of mercury and the alloy is referred to as trituration or amalgamation. A surface reaction occurs between the alloy and liquid mercury that binds the unreacted particles together by a surrounding matrix of reaction products. Increasing the copper content eliminates most of the weakest and most corrosive phase (Sn7–8Hg) from the setting reaction.
After the carious lesion is removed, the plastic dental amalgam is condensed into the prepared cavity before hardening and subsequently retained by the mechanical resistance and retention form of the surgically prepared cavity. An adhesive liner is not required. The material slowly corrodes and the corrosion products ‘‘self-seal’’ the margin between tooth and amalgam, thereby protecting the tooth from leakage of oral fluids and bacteria and their byproducts. Dental amalgam is brittle and undergoes creep at mouth temperature, which can lead to marginal or bulk fracture and clinical failure. However, if the cavity is well designed and the amalgam placed with technical competence, many years of service should be expected. A vast number of studies have shown the safety and efficacy of dental amalgam. When a dentist is faced with a patient’s request to remove amalgam fillings due to a claimed medical malady, the dentist is professionally obligated to explain that the possibility of their medical condition(s) being related to the presence of dental amalgam fillings is extremely remote. These patients typically face a complex problem with biological, psychological, and social components unrelated to mercury intake.
Resin Composites
Modern day resin-based composites placed with dental adhesives have replaced the silicates and acrylic resins of the past and are now widely used throughout dentistry. In addition to the treatment of decay and trauma, RBCs are used in aesthetic or cosmetic dentistry procedures due to their versatility and conservative nature. Discolored, misshapen, or misaligned dentition can be aesthetically treated with cosmetic ‘‘bonding’’. The RBCs are composed of four main components: (1) a continuous organic polymer matrix, (2) a dispersion of inorganic filler particles, (3) silane coupling agents to bind the filler particles with the polymer matrix, and (4) an initiator–accelerator system. They also contain various pigments for matching tooth shades and ultraviolet (UV) absorbers to minimize oxidative color changes. The two most common oligomers are the dimethacrylates 2,2- bis[4(2-hydroxy-3-metharcy- loyloxy-propyloxy)-phenyl] (bis-GMA) and urethane dimethacrylate (UDMA). Diluents such as triethylene glycol dimethacrylate (TEGDMA) are added to reduce the viscosity for the addition of filler and to obtain clinically acceptable handling properties. The inorganic filler particles can be of borosilicate, lithium aluminum silicate, barium aluminum silicate, strontium or zinc glasses, quartz, or colloidal silica. The combination of relatively larger glass or quartz particles and a significant addition of
BIOMATERIALS FOR DENTISTRY |
323 |
Table 1. Classification of Resin-Based Composites by Filler Particle Size
Class |
Particle Size, mm |
Filler Loading, vol% |
|
|
|
Macrofill |
>10 |
50–70 |
Midifill |
1–10 |
50–70 |
Minifill |
0.1–1 |
50–70 |
Microfill |
0.01–0.1 |
20–50 |
|
|
|
colloidal silica are referred to as a ‘‘hybrid’’. A useful classification method is by filler particle size (Table 1) with minifill hybrids and microfills being the most popular types. Recently, using a proprietary process, manufacturers have been able to produce a smaller average silica particle size (0.02 mm) as compared with traditional microfills (0.04 mm). Marketed as ‘‘nanofills’’, these smaller silica particles are produced in a nondrying method thereby avoiding agglomeration due to physical forces and thusly enabling a higher degree of filler loading. The microfill RBCs polish to the most enamel-like surface, but lack the strength of the hybrids due to the lower filler volume. Newer formulations of minifill hybrids can be used as ‘‘universal’’ RBCs possessing both the strength for posterior chewing forces and acceptable surface finish for use in aesthetic anterior regions. Clinical studies showed that the long-term wear resistance of RBC restorations placed on posterior teeth is still inferior to the dental amalgam restorations.
Polymerization occurs through either a self-cured free radical initiation when a peroxide–amine system is mixed or through light-activated free radical initiation when a diketone–amine system is exposed to blue light. The photoactivator, most commonly camphoroquinone, is added in small amounts, which forms a free radical when exposed to 467-nm blue light. Dual-cure varieties of RBCs are available as well. Halogen light-curing units are most commonly used, but several other light curing units are currently being marketed to include: plasma-arc, laser and lightemitting diodes. To insure proper polymerization care must be taken to match the unit’s spectral emission and the RBCs spectral requirements. One aspect that all RBCs currently share is 2–4% volumetric shrinkage of the continuous polymeric network upon polymerization. Shrinkage induces residual stress that can disrupt the adhesive bond between the RBC and the tooth structure, damage enamel or the RBC. Incremental placement of light-cured RBCs can help to reduce the net effect of this shrinkage stress. Manufacturer’s are currently working to develop noor low shrinkage RBCs; several approaches are noted: (1) addition of ring-opening monomers that expand upon polymerization (spiroorthocarbonates, oxiranes, vinylcyclopropanes), (2) low shrinkage cyclopolymerizable diand multifunctional oligomers synthesized through the reaction of acrylates and formaldehyde, and (3) the addition of strain-absorbing polybutadiene rubber polymer adsorbed onto the fumed silica.
Variations in filler loading, viscosity, and polymerization initiating systems allow RBCs to be used in a wide variety of clinical situations, to include: sealants, cements, crown core buildups, so-called flowables and packables,
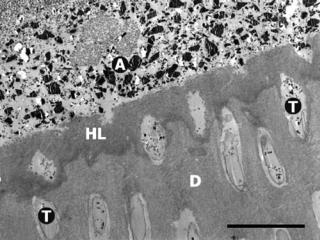
324 BIOMATERIALS FOR DENTISTRY
provisional restorations, and in a variety of laboratory processed RBCs for adhesive cementation as inlays, onlays, crowns and veneers. Several manufacturer’s have promoted fiber reinforced RBCs for use as bridges, as well, but these lack convincing clinical data for their recommended usage.
Glass Ionomers
Smith (1968) developed glass ionomer cements (GIC) by combining the polyacrylic acid from polycarboxylate cement, which is strongly adhesive to tooth structure, and the aluminosilicate glass from the fluoride-containing silicate cements. The GICs form a true chemical bond to the tooth mineral (hydroxyapatite) by ionic bonding between calcium and carboxylic ions and act as chemotherapeutic agents in the treatment and prevention of dental caries through the release of fluoride.
The GIC are composed of a basic ion-leachable aluminosilicate glass powders that, upon exposure to watersoluble homopolymer or copolymers of alkenoic acids, form a matrix of continuous polysalts (polyalkenoates) surrounded by partially solubilized glass filler particles. The clinical placement technique must account for the relatively slow-setting reaction and moisture sensitivity. Fluoride easily passes in and out of the matrix without any degradative effects due to the substitution of carboxyl ions for fluoride within the salt matrix. Fluoride ‘‘recharging’’ has been clearly demonstrated in vitro to prevent the demineralization of tooth structure at the margins adjacent to the GIC under an artificial caries challenge. In vivo evidence is not as clearly demonstrated, but when evaluating clinical data from high caries risk cohort populations the anticariogenic effect of GIC is elucidated.
Since their development GIC has been modified in a number of different ways to improve their clinical handling properties and durability. A significant development was the addition of water soluble monomers, for example, HEMA, and the grafting of methacrylate side groups on the polyacid polymer. By the addition of visible light initiator–accelerator systems these resin-modified glass ionomers (RMGI) can be command set with a light curing unit while also self-curing through the acid–base reaction. These improvements to the conventional GIC and RMGI have made these materials widely used as restorative materials. The self-adhesive and self-cure properties of GIC, along with improvements in strength have allowed these materials to be used in nontraditional field situations without the luxuries of electricity or modern equipment. Auxiliary personnel have been trained to remove caries with sharp hand instruments with the cavity then filled with a heavier filled GIC. This treatment has aided thousands through a technique termed Atraumatic Restorative Treatment or ‘‘ART’’. These materials are also used as cements and liners.
Adhesives, Cements, and Liners
Adhesive dentistry has become increasingly important as the use of dental amalgam and direct compacted gold foil and cemented gold restorations has declined. Unlike dental amalgam, RBCs require an adhesive liner for placement
and retention. A durable bond of RBCs to enamel can be accomplished by first cleaning and demineralizing the surface with a 30–40% phosphoric acid, followed by a polymerizable methacrylate monomer [bis(GMA), UDMA, TEGDMA], which diffuses into the porosities created by the acid etching. However, bonding to dentin is a much greater challenge due to the compositional differences in dentin relative to enamel and its extremely variable clinical presentation. Dentin contains less inorganic components and more organic components and water than enamel. Dentin is made permeable by tubules that travel from the dental pulp through the dentin to the coronal enamel.
Similar to enamel, the dentin is treated with an acid that removes any smear layer and exposes the collagen fibers by demineralizing the surface. An adhesive primer containing a hydrophilic solvent (water, acetone, ethanol, or HEMA) and an amphipathic monomer (hydrophilic– hydrophobic functionalities) then penetrates the exposed collagen network. After the solvent is evaporated from the primed surface, an adhesive monomer is applied that attaches to the hydrophobic functionality of the primer to create a wetted surface for subsequent copolymerization with the RBC. This bonding process is also approached with so-called ‘‘self-etch’’ adhesives. The initial acid application is eliminated and a water-soluble acidic polymer is included in the primer to simultaneously demineralize the tooth surface while penetrating with the adhesive monomers and oligomers. Regardless of the approach, the adhesive liner penetrates into the exposed collagen network and also partially into the dentinal tubules. The interdiffusion of the synthetic adhesive polymer within the collagen network forms a micromechanical bond and is commonly referred to as a hybrid layer (Fig. 1). In the last 20 years,
Figure 1. Scanning electron micrograph (SEM) image of a totaletch three-step dental adhesive system applied to dentin. A ¼ filled adhesive resin (ceramic filler particles visible within resin matrix), HL ¼ hybrid layer (interdiffusion zone of adhesive polymers and collagen network), T ¼ dentinal tubules, D ¼ laboratory demineralized dentin. Original magnification ¼ 2000X, black bar¼10 mm. (Photomicrograph courtesy of Marcos Vargas, University of Iowa College of Dentistry.)
dentin bonding has improved the clinical success of adhesively placed restorations, but difficulties remain. Completely penetrating the exposed collagen matrix with the adhesive monomers can be hindered by the presence of excess solvent, dentinal fluids, or by physical blockage of the interpenetrating microchannels between collagen fibrils. Even if the adhesive fully wets the dentin surface, suboptimal polymerization may reduce bonding effectiveness. This union of restorative material to enamel and dentin is critical not only for retaining restorations in place, but also for sealing the margin from the passage of bacterial fluids, molecules, or ions. Leakage between the interface of the dental restoration and the wall of the cavity preparation has been associated with marginal discoloration, secondary caries, and pulpal pathology.
Adhesive liners or bonding agents are also used in conjunction with resin-based composite cements when adhesively cementing crowns or other fixed appliances into or onto prepared teeth. The ceramic elements or oxides of the internal surface of dental porcelain–ceramic, metals, and resin-based composite restorative materials are mechanically or chemically roughened before applying a silane coupling agent. The silane bonds to the ceramic surface with both covalent and hydrogen-bonding protecting from hydrolytic degradation while making the surface hydrophobic and organophilic for resin cement wettability and copolymerization. Self-adhesive cements include the glass ionomer and polycarboxylate cements. Zinc phosphate and zinc oxide eugenol are nonadhesive cements that act as mortar or a luting agent.
Additional uses of the above mentioned cements include (1) cavity liners to achieve a physical barrier to bacteria and their products and/or to provide a therapeutic effect, such as fluoride release from glass ionomer or pulpal obtundent with zinc oxide eugenol, (2) cavity bases to block out undercuts in cavity preparations for indirect restorations or for insulating the pulp from thermal changes, and (3) temporary or provisional restorations. The beneficial effects of adhesion to tooth structure and fluoride release obtained from adhesive liners and glass ionomer materials are rapidly replacing the traditional liner, base, and cement materials, that is, zinc phosphate, polycarboxylate, and zinc oxide eugenol.
PROSTHETIC RESTORATIVE MATERIALS:
CROWNS, BRIDGES, DENTURES
Metals and Alloys
Noble and base metal alloys are used for (1) crowns and bridges with fused porcelain in esthetic areas, (2) inlays, onlays, crowns, and bridges without porcelain veneering in the posterior or nonaesthetic regions of the mouth, and (3) partial and complete removable denture bases. Base metals commonly used in dental alloys include, nickel, chromium, copper, zinc, gallium, silver, indium, and tin. Silver, a ‘‘precious’’ metal, is not considered a noble metal in dentistry due to its corrosion in the oral cavity. The noble metals utilized in dentistry are gold, platinum, palladium, iridium, rhodium, and ruthenium. Cold-worked or wrought noble and base alloys can be cast with or ‘‘soldered’’
BIOMATERIALS FOR DENTISTRY |
325 |
(brazed) to cast structures as attachments or clasps to removable partial dentures. High purity gold, being soft and malleable, can be cohesively adapted in the form of gold foil into small cavity preparations by careful condensation techniques. This process develops adequate hardness and physical properties through work hardening, resulting in a clinically successful, long-lasting restoration. However, the compacted gold foil restoration is becoming increasingly uncommon due to the success of adhesively bonded tooth colored restorations and the skill and time required to properly place gold foil. Almost all fixed-dental prostheses contained a minimum of 75% gold before the dramatic increase in the price of gold after the United States separated gold from monetary standards in 1969. The increase in gold prices, and rise in palladium prices three decades later, has lead to an increased use of alternative alloys containing base metals.
The lost wax technique became common in dentistry after W.H. Taggart introduced his casting machine in 1907. A wax pattern of the desired restoration is fabricated and then invested in a ceramic material (casting investment), which is subsequently heated to burn out the wax pattern, that is ‘‘lost wax’’. A molten metal alloy is then cast into the resultant space previously occupied by the wax. The restoration is recovered, finished, and polished before cementing or delivering to the patient. The investment must be able to expand enough upon setting and heating to compensate for the wax and metal shrinkage if a precise fit is to be obtained.
Alloys should (1) produce no toxic, carcinogenic, or allergic reactions; (2) resist corrosion and physical changes in the oral environment; (3) possess physical properties, that is, strength, fusing temperature, thermal conductivity, and coefficient of thermal expansion, appropriate for the desired application; (4) be able to be fabricated in a technically feasible manner; and (5) be available and relatively inexpensive. The alloys used for metal-ceramic or commonly termed porcelain-fused-to-metal (PFM) restorations must possess a fusion temperature range that is substantially higher (>100 8C) than the ceramic firing temperature, have sufficient creep resistance at that temperature, and have the ability to form a good bond between its oxide surface and the ceramic veneer.
Wrought stainless steel alloys are used in orthodontic brackets and wires, endodontic instruments, prefabricated temporary crowns and space maintainers. In addition, wrought cobalt–chromium–nickel, nickel–titanium, cop- per–nickel–titanium, and beta-titanium alloys are also used as orthodontic wires. Nickel–titanium and copper– nickel–titanium orthodontic wires have a unique superelastic (pseuedoelastic) property that delivers a constant low-level force over an extended range of deformation. Nickel–titanium and copper–nickel–titanium alloys are also the shape memory alloy (SMA), that is, they can be deformed plastically below its transition temperature range (TTR), then after heating through and above the TTR, they will return to their original desired shape due to a crystallographic transformation from martensitic phase into austenitic phase. Titanium and titanium alloys, especially due to their thin stable oxide layers, are very important endosseous dental implant materials and, with the
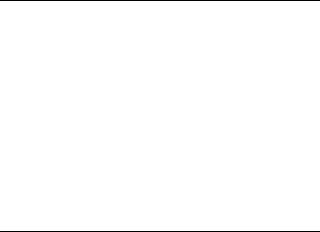
326 BIOMATERIALS FOR DENTISTRY
recent refinement in casting techniques, can be used for crowns, partial dentures and complete denture bases.
Ceramics
The first porcelain was developed in the T’ang Dynasty from 618 to 906 AD and the first suggested use of porcelain for dentistry was by Pierre Fauchard in France after the porcelain formula was brought from China by a Jesuit priest. Several developments followed but the current approaches to ceramic-metal crowns and bridges occurred from the 1950s–1960s. High-fusing alloys combined with the development of low-fusing thermally compatible leucitic porcelains permitted the fabrication of ceramic-metal restorations. High expansion leucite was mixed with feldspar glass during manufacturing to refine the coefficient of thermal expansion (a) creating a successful junction between dental porcelain and metal. The combination of which must be thermally compatible for fabrication and so that the veneering materials surface is left in a residual state of compression. The a of the veneering material is generally 0.5–1.0 10 6 8C 1 lower than the core material so that upon cooling the inner core will contract more resulting in a residual compressive state resisting crack formation and propagation of the relatively brittle veneering material.
Minor refinements have continued with metal–ceramic systems, but more recently significant advances have occurred in the area of ‘‘all-ceramic’’ systems, in which the metal is replaced with a ceramic core upon which veneering porcelain is fused. This eliminates the masking of the metal with opaquing agents and greatly simplifies the aesthetic technical procedures by the dental technician. However, more tooth structure may need to be removed in preparation for the construction of these allceramic restorations and their brittle nature does not yet permit their function in long-span bridges.
Ceramic systems can be classified by their fusion temperature, clinical usage, processing methods, or crystalline phase. Table 2 lists some crystalline types used for the three major applications of ceramics in dentistry: (1) metal–ceramic crowns and fixed-partial dentures
Table 2. Classification of Dental Ceramic Materials
|
|
Crystalline (dispersed) |
|
Fabrication |
Phase |
|
|
|
Ceramic–Metal |
Sintered |
Leucite (KAlSi2O6) |
(PFM) |
|
|
All-Ceramic |
Machined |
Alumina (Al2O3) |
|
|
Feldspar (KAlSi3O8) |
|
|
Mica (KMg2.5,Si4O10F2) |
|
Slip-cast |
Alumina (Al2O3) |
|
|
Spinel (MgAl2O4) |
|
|
Zirconia (ZrO2) |
|
Heat-pressed |
Leucite (KAlSi2O6) |
|
|
Lithium disilicate |
|
|
(Li2Si2O5) |
|
Sintered |
Alumina (Al2O3) |
|
|
Leucite (KAlSi2O6) |
Denture Teeth |
|
Feldspar (KAlSi3O8) |
aAdapted from Ref. (1) p. 553.
(bridges); (2) all-ceramic crowns, inlays, onlays, veneers, and shortspan bridges; and (3) denture teeth.
‘‘Porcelains’’ are composed of kaolin (clay), feldspar, and quartz (flint), while the dental porcelains being quite similar, are fabricated from silica (SiO2), soda (NaO2), potash (K2O), alumina (Al2O3), with the addition of pigments, opacifiers and fluxes. Naturally occurring minerals such as feldspar (K2O Al2O3 6SiO2), quartz, and nepheline syenite have been utilized to provide these constituents. The use of feldspar has lead to the term feldspathic porcelain, however, feldspar is not necessarily present in the final processed porcelain, nor is it essential to form leucite, the major crystalline phase of the porcelain. Like all dental ceramics, dental porcelain is composed of a glassy (vitreous) matrix phase surrounding a dispersed crystalline phase. The glassy matrix, composing 75–85% of the porcelain, is formed by heating the raw materials into a glassy state then quenching. This pyrochemical reaction produces a supercooled liquid of metastable equilibrium that is quenched then ground into a fine powder. These fine powders or frit can be reheated and will fuse at a lower temperature with little pyroplastic flow giving increased homogeneity, translucency, smoother texture, a lower fusion temperature, and less shrinkage. The temperature at which the surface glassy phase softens allowing the fritted particles to coalesce without further pyrochemical change is called sintering. These sintered dental porcelains, in general, will have little change in the physical, chemical, or optical properties of the glassy matrix upon repeated firings during the necessary steps of the restoration fabrication. However, if improperly fired or over fired, the dispersed leucite crystals can be altered leading to reduced strength or porcelain–metal (core) thermal incompatibilities. During the fritting process the silica matrix is disordered due to the rapid cooling from the molten state and also by the addition of fluxes that break up the silica tetrahedral network by occupying oxygen. These alkali ions reduce the number of cross-linkages between the silicon–oxygen tetrahedra by randomly occupying space in the open network. The net effect of flux (LiO2, Na2O, K2O, BaO, CaO, MgO, ZnO) addition is lower softening or fusion temperature, decreased viscosity, production of glassy phase, increased a, decreased strength, lowered chemical resistance, increased risk of devitrification during repeated firing cycles. The lower fusing temperatures and increased a made possible the modern day metal– ceramic systems. Three components of the porcelain to metal bond are classically described: (1) mechanical interlocking through good wetting of the porcelain on the roughened metal surface, (2) chemical physical bonding between the oxides of the porcelain and the oxides on the metal surface, and (3) a controlled mismatch in a leading to residual compressive forces in the porcelain (described earlier). Any of these may predominate depending on the ceramic system.
Achieving superior esthetics, in general, is simplified by having a ceramic core. However, strength, wear, fit, and longevity must be proven in controlled clinical trials. Increasing the strength of the ceramic core to perform comparably to metal substructures is approached by manipulating the crystalline phase for reinforcement.
Techniques for fabricating all-ceramic systems include (1) sintering with alumina-based, magnesiabased, and leu- cite-reinforced ceramics; (2) heat-pressed techniques with leucite-reinforced and lithium-disilicate-based ceramics; (3) slip-casting with alumina-, spinel-, and zirconia-based ceramics; and (4) the machining of manufactured ceramic blocks available in several types of ceramic. One method uses computer-aided designing/computer aided machining (CAD/CAM) technology to fabricate inlays, onlays, veneers, and crowns. An ‘‘optical’’ impression is obtained from the prepared tooth with an optical scanner and the computerized image of the restoration is designed by the computer software. Subsequently, a ceramic block is machined in the computer-controlled milling machine according to the design and later cemented into or on the tooth by the dentist.
Slip-cast all-ceramics are fabricated by a process very similar to that used for the production of common objects such as plumbing fixtures and beer steins. Successive layers of ceramic slurry are applied to porous refractory gypsum that draws in the water depositing a solid layer of alumina on its surface. The ceramic buildup is dried then sintered for 4 h at 1100 8C, the porous alumina coping is then carved into the desired shape before infiltrating with a slurry of lanthanum aluminosilicate glass by firing at 1120–1150 8C for 3–5 h. The resultant ceramic is a threedimensional (3D) interpenetrating network of alumina and glass of high strength due to the presence of densely packed alumina and low porosity. The excess glass is removed and the core is subsequently veneered with a thermally compatible veneering ceramic. Improved translucency (esthetics) can be obtained by glass infiltrating a core composed of magnesium spinel and alumina. The strongest slip-cast material currently available contains tetragonal zirconia along with alumina and glass. When a load is induced on the tetragonal zirconia it absorbs energy by transforming into a monoclinic crystal form accompanied by a volume increase of 3% in a crack arresting manner.
Flexural strengths (380–700 MPa) and fracture toughness (2–7 MPa m1/2) for these core materials are in the following
rank order: spinel <alumina <zirconia.
Prosthetic Resin Materials
Poly(methylmethacrylate) was introduced as a denture base material in 1937 and in roughly a decade had virtually replaced the use of vulcanite. Acrylic polymers also enjoy a wide variety of uses in additional prosthetic applications, such as, artificial denture teeth, provisional restorations and temporary crowns, denture base repair, relining and rebasing materials, and obturators for maxillofacial defects.
Denture base materials are typically fabricated from heat-cured poly(methylmethacrylate) and rubber-rein- forced poly(methylmethacrylate) and perform surprisingly well. These plastics are supplied in a powder liquid or gel form. The 10 poly(methylmethacrylate) powder is modified with ethyl, butyl, or other alkyl methacrylates for impact resistance and contains benzoyl peroxide or diisobutylazonitrile to initiate polymerization when mixed with the liquid monomer. Pigments are added to obtain natural
BIOMATERIALS FOR DENTISTRY |
327 |
tissue appearance, for example, mercuric sulfide, cadmium sulfide, cadmium selenide, ferric oxide, or carbon black. Various glasses, ceramics and polymer fibers have been added as dispersed phases to various products in an attempt to reinforce the acrylic polymers. The liquid component is methyl methacrylate, modified with various other monomers while including an inhibitor such as hydroquinone to prevent premature polymerization for adequate shelf life. The liquid of cold-, self-, or autocuring resins contain tertiary amine or sulfinic acid chemical accelerators to allow the polymerization of the monomer at room temperature. Plasticizers for resilience and crosslinkers for hardness and decreased solubility may also be included. Denture base resins can also be fabricated through pressure, heat and light-activated techniques with compositional modifications for the various initiation reactions and physical handling properties during fabrication. A number of general requirements for denture base resins are outlined in ANSI/ADA Specification No. 12 (ISO 1567) providing guidance to dentists and dental manufacturers.
Denture teeth are also fabricated from acrylic and modified acrylic materials and are generally preferred over porcelain denture teeth due to wear characteristics, phonetics and technical considerations during fabrication and repair. Temporary or provisional restorations are also fabricated from acrylic-based resins, placed during an interim period in or over the coronal aspects of the tooth while a crown or bridge is fabricated in the dental laboratory. Due to ease of fabrication and tooth-like appearance these are much more popular than aluminum shells or polycarbonate crowns that typically must be relined before temporary cementation to the tooth.
Defects of the head and neck resulting from cancer surgery, accidents and congenital deformities have been corrected with a wide variety of maxillofacial resin materials, including poly(methylmethacrylate), plasticized polyvinylchloride, polyurethane, heatvulcanized and room temperature-vulcanized (RTV) silicone and a whole host of various other elastomers. It is important to use prosthetic resin materials with color stability, ease of fabrication, dimensional stability, edge strength, flexibility, low thermal conductivity, biocompatibility, and surface texture to achieve clinical success and patient acceptance. Silicones are the most widely used materials for facial restorations in the United States, with RTV Silicone MDX-4-4210, possessing surface texture and hardness within the range of human skin. The prosthesis can be held in place by tissue undercuts, the patient’s glasses or dentures, medical grade adhesives, magnetic attachment to endosseous implantretained metallic attachments or bars or through a combination of methods. A mold is made from an impression of the defect upon which a prosthesis is fabricated and color matched by mixing small amounts of pigments into the elastomer. Surface coloration and texturing is completed and the patient returns periodically for esthetic touchups to achieve a lifelike match to the skin.
Implants
The surgical placement of endosseous dental implants to support dental restorations has become a routine aspect of
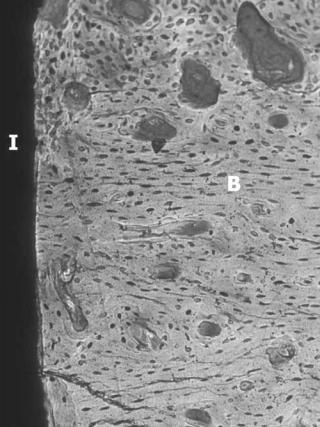
328 BIOMATERIALS FOR DENTISTRY
Figure 2. Dental implant demonstrating osseointegration. I ¼ implant, B ¼ bone. (Courtesy of John Keller, University of Iowa College of Dentistry.)
dental care enjoying a high success rate. Commercially pure titanium (CpTi) and Ti-6Al-4V are the materials most commonly used for endosseous dental implants. The stable oxides surfaces formed on CpTi and Ti- 6Al-4V have proven to successfully biointegrate with bone. The terms osseointegration and functional ankylosis are used to describe the direct bone apposition on the implant surface giving evidence to support a direct biochemical bonding (Fig. 2). The clinical success of these implants depends not only on osseointegration, but also the quantity, quality and distribution of bone at the implant site, the technical skill during surgical placement, and the timing and degree of mechanical loading under function. Many other factors play a role but six biological and technical factors are recognized as key to implant success: (1) implant surface texture, (2) biocompatibility, (3) implant design, (4) host tissue condition, (5) surgical technique, and (6) loading conditions.
Lower success is observed in areas of the mouth that may have less cancellous bone or thin cortical plates such as the posterior regions of the maxilla. Therefore, attempts are made to manipulate the osseous response to the implant so that the bone quantity and quality at the implant interface is optimized for the clinical requirements. This will not only help the routine implant but is especially important for those patients with: (1) poor bone
quality, (2) heavy masticatory loading, and (3) the need for multiple tooth replacement. Surgical procedures are also utilized that enhance the osseous tissues at the intended implant site by autoor allo-grafting.
Implant surface modifications have been heavily investigated with every major manufacturer offering various implant designs and surface textures. Two of the most thoroughly investigated and successful surfaces are machined titanium and titanium plasma-sprayed (TPS) surface, with the latter significantly increasing the surface area for bone contact. These rougher surfaces have been shown to require higher forces to be removed from the bone than do smoother surface implants and may allow: shorter healing periods, the use of less invasive shorter implants and may not require bicortical implant engagement. Improving the bone adaptation through microretentive mechanisms can be divided into those that attempt to enhance the immigration of new bone through surface topography, that is, osteoconduction, and those that attempt to manipulate the type of cell response and growth for new bone formation, that is, osteoinduction. Osteinductive methods also include the use of the implant as a delivery device for biomolecules for the induction of the desired response. A complex cascade of molecular and cellular processes occur after the placement of the implant into a surgical site, many of which are just now beginning to be understood, leading to the possibility of implantmediated tissue engineering. Calcium phosphates or ‘‘hydroxyapatite’’ can also be coated on titanium implants and have been documented to create a very intimate bone- to-implant contact with a reduced healing period; however, the long-term results are less favorable than that achieved with TPS due to surface degradation and coating separation problems.
Macroretentive features are also part of the implant design including: screw threads, solid body press-fit designs, and sintered bead technology. These macroretentive features are intended to improve initial implant stability and enhanced bone ingrowth. Without the aid of a periodontal ligament (present between the natural tooth root and bone) the bone responds most favorable to compressive loading, which must accounted for in the implant design.
The original guidelines for implant success have changed over time with an increased use of nonsubmerged (not covered by the gum tissue) and single-staged surgical techniques (immediate abutment placement). These timeand cost-saving changes have come about after studies revealed similar end-results, in terms of ‘‘biological width’’ (composed of junctional epithelial and connective tissue attachment) and clinical survival rates. Considerations such as these are blurring the distinction between the clinical healing period (Phase I) and the functional period (Phase II). Additionally, studies have shown similar clinical predictability using both solely implant supported and mixed tooth-implant-supported fixed partial dentures (bridges), while the use of cemented rather than screwretained prostheses have reduced technique complications.
Abutments of either titanium or alumina, as compared with those abutments of more ‘‘esthetic’’ quality, such as gold alloys or dental porcelains, are most likely to have favorable soft-tissue healing with formation of a