
- •Preface
- •Acknowledgements
- •Contents
- •1.1 Introduction
- •1.2 Classical Physics Between the End of the XIX and the Dawn of the XX Century
- •1.2.1 Maxwell Equations
- •1.2.2 Luminiferous Aether and the Michelson Morley Experiment
- •1.2.3 Maxwell Equations and Lorentz Transformations
- •1.3 The Principle of Special Relativity
- •1.3.1 Minkowski Space
- •1.4 Mathematical Definition of the Lorentz Group
- •1.4.1 The Lorentz Lie Algebra and Its Generators
- •1.4.2 Retrieving Special Lorentz Transformations
- •1.5 Representations of the Lorentz Group
- •1.5.1 The Fundamental Spinor Representation
- •1.6 Lorentz Covariant Field Theories and the Little Group
- •1.8 Criticism of Special Relativity: Opening the Road to General Relativity
- •References
- •2.1 Introduction
- •2.2 Differentiable Manifolds
- •2.2.1 Homeomorphisms and the Definition of Manifolds
- •2.2.2 Functions on Manifolds
- •2.2.3 Germs of Smooth Functions
- •2.3 Tangent and Cotangent Spaces
- •2.4 Fibre Bundles
- •2.5 Tangent and Cotangent Bundles
- •2.5.1 Sections of a Bundle
- •2.5.2 The Lie Algebra of Vector Fields
- •2.5.3 The Cotangent Bundle and Differential Forms
- •2.5.4 Differential k-Forms
- •2.5.4.1 Exterior Forms
- •2.5.4.2 Exterior Differential Forms
- •2.6 Homotopy, Homology and Cohomology
- •2.6.1 Homotopy
- •2.6.2 Homology
- •2.6.3 Homology and Cohomology Groups: General Construction
- •2.6.4 Relation Between Homotopy and Homology
- •References
- •3.1 Introduction
- •3.2 A Historical Outline
- •3.2.1 Gauss Introduces Intrinsic Geometry and Curvilinear Coordinates
- •3.2.3 Parallel Transport and Connections
- •3.2.4 The Metric Connection and Tensor Calculus from Christoffel to Einstein, via Ricci and Levi Civita
- •3.2.5 Mobiles Frames from Frenet and Serret to Cartan
- •3.3 Connections on Principal Bundles: The Mathematical Definition
- •3.3.1 Mathematical Preliminaries on Lie Groups
- •3.3.1.1 Left-/Right-Invariant Vector Fields
- •3.3.1.2 Maurer-Cartan Forms on Lie Group Manifolds
- •3.3.1.3 Maurer Cartan Equations
- •3.3.2 Ehresmann Connections on a Principle Fibre Bundle
- •3.3.2.1 The Connection One-Form
- •Gauge Transformations
- •Horizontal Vector Fields and Covariant Derivatives
- •3.4 Connections on a Vector Bundle
- •3.5 An Illustrative Example of Fibre-Bundle and Connection
- •3.5.1 The Magnetic Monopole and the Hopf Fibration of S3
- •The U(1)-Connection of the Dirac Magnetic Monopole
- •3.6.1 Signatures
- •3.7 The Levi Civita Connection
- •3.7.1 Affine Connections
- •3.7.2 Curvature and Torsion of an Affine Connection
- •Torsion and Torsionless Connections
- •The Levi Civita Metric Connection
- •3.8 Geodesics
- •3.9 Geodesics in Lorentzian and Riemannian Manifolds: Two Simple Examples
- •3.9.1 The Lorentzian Example of dS2
- •3.9.1.1 Null Geodesics
- •3.9.1.2 Time-Like Geodesics
- •3.9.1.3 Space-Like Geodesics
- •References
- •4.1 Introduction
- •4.2 Keplerian Motions in Newtonian Mechanics
- •4.3 The Orbit Equations of a Massive Particle in Schwarzschild Geometry
- •4.3.1 Extrema of the Effective Potential and Circular Orbits
- •Minimum and Maximum
- •Energy of a Particle in a Circular Orbit
- •4.4 The Periastron Advance of Planets or Stars
- •4.4.1 Perturbative Treatment of the Periastron Advance
- •References
- •5.1 Introduction
- •5.2 Locally Inertial Frames and the Vielbein Formalism
- •5.2.1 The Vielbein
- •5.2.2 The Spin-Connection
- •5.2.3 The Poincaré Bundle
- •5.3 The Structure of Classical Electrodynamics and Yang-Mills Theories
- •5.3.1 Hodge Duality
- •5.3.2 Geometrical Rewriting of the Gauge Action
- •5.3.3 Yang-Mills Theory in Vielbein Formalism
- •5.4 Soldering of the Lorentz Bundle to the Tangent Bundle
- •5.4.1 Gravitational Coupling of Spinorial Fields
- •5.5 Einstein Field Equations
- •5.6 The Action of Gravity
- •5.6.1 Torsion Equation
- •5.6.1.1 Torsionful Connections
- •The Torsion of Dirac Fields
- •Dilaton Torsion
- •5.6.2 The Einstein Equation
- •5.6.4 Examples of Stress-Energy-Tensors
- •The Stress-Energy Tensor of the Yang-Mills Field
- •The Stress-Energy Tensor of a Scalar Field
- •5.7 Weak Field Limit of Einstein Equations
- •5.7.1 Gauge Fixing
- •5.7.2 The Spin of the Graviton
- •5.8 The Bottom-Up Approach, or Gravity à la Feynmann
- •5.9 Retrieving the Schwarzschild Metric from Einstein Equations
- •References
- •6.1 Introduction and Historical Outline
- •6.2 The Stress Energy Tensor of a Perfect Fluid
- •6.3 Interior Solutions and the Stellar Equilibrium Equation
- •6.3.1 Integration of the Pressure Equation in the Case of Uniform Density
- •6.3.1.1 Solution in the Newtonian Case
- •6.3.1.2 Integration of the Relativistic Pressure Equation
- •6.3.2 The Central Pressure of a Relativistic Star
- •6.4 The Chandrasekhar Mass-Limit
- •6.4.1.1 Idealized Models of White Dwarfs and Neutron Stars
- •White Dwarfs
- •Neutron Stars
- •6.4.2 The Equilibrium Equation
- •6.4.3 Polytropes and the Chandrasekhar Mass
- •6.5 Conclusive Remarks on Stellar Equilibrium
- •References
- •7.1 Introduction
- •7.1.1 The Idea of GW Detectors
- •7.1.2 The Arecibo Radio Telescope
- •7.1.2.1 Discovery of the Crab Pulsar
- •7.1.2.2 The 1974 Discovery of the Binary System PSR1913+16
- •7.1.3 The Coalescence of Binaries and the Interferometer Detectors
- •7.2 Green Functions
- •7.2.1 The Laplace Operator and Potential Theory
- •7.2.2 The Relativistic Propagators
- •7.2.2.1 The Retarded Potential
- •7.3 Emission of Gravitational Waves
- •7.3.1 The Stress Energy 3-Form of the Gravitational Field
- •7.3.2 Energy and Momentum of a Plane Gravitational Wave
- •7.3.2.1 Calculation of the Spin Connection
- •7.3.3 Multipolar Expansion of the Perturbation
- •7.3.3.1 Multipolar Expansion
- •7.3.4 Energy Loss by Quadrupole Radiation
- •7.3.4.1 Integration on Solid Angles
- •7.4 Quadruple Radiation from the Binary Pulsar System
- •7.4.1 Keplerian Parameters of a Binary Star System
- •7.4.2 Shrinking of the Orbit and Gravitational Waves
- •7.4.2.1 Calculation of the Moment of Inertia Tensor
- •7.4.3 The Fate of the Binary System
- •7.4.4 The Double Pulsar
- •7.5 Conclusive Remarks on Gravitational Waves
- •References
- •Appendix A: Spinors and Gamma Matrix Algebra
- •A.2 The Clifford Algebra
- •A.2.1 Even Dimensions
- •A.2.2 Odd Dimensions
- •A.3 The Charge Conjugation Matrix
- •A.4 Majorana, Weyl and Majorana-Weyl Spinors
- •Appendix B: Mathematica Packages
- •B.1 Periastropack
- •Programme
- •Main Programme Periastro
- •Subroutine Perihelkep
- •Subroutine Perihelgr
- •Examples
- •B.2 Metrigravpack
- •Metric Gravity
- •Routines: Metrigrav
- •Mainmetric
- •Metricresume
- •Routine Metrigrav
- •Calculation of the Ricci Tensor of the Reissner Nordstrom Metric Using Metrigrav
- •Index
2 |
1 Special Relativity: Setting the Stage |
paper of Gauss on curved surfaces and, through the work of Riemann, Christoffel, Ricci-Curbastro, Bianchi, Levi Civita and the young Cartan, had reached a high degree of development. It was time to adapt Physics to this higher level mathematical language and to incorporate the basic concepts of the new geometry among the building blocks utilized to formulate the fundamental laws of Nature. Einstein did so with General Relativity, discovering that gravitation is nothing else but a manifestation of the curvature of space-time, interpreted as a Riemannian differentiable manifold. Curiously, Maxwell Theory which, via its Lorentz covariance, motivated Special Relativity, was also a theory of curvature in disguise: the curvature of a principle connection on a fibre-bundle. Indeed Electromagnetism is the simplest example of what we name nowadays gauge-theories and the electromagnetic potential Aμ is the simplest example of a principal connection. Today we know that more complicated connections on non-Abelian principal bundles describe the other nongravitational fundamental interactions.
1.2Classical Physics Between the End of the XIX and the Dawn of the XX Century
James Clerk Maxwell (see Fig. 1.1) put Classical Physics into perfection performing, after that encoded in Newton’s Law, the second great unification of Physics. Before Maxwell there were, on one side, electrostatics, electrodynamics and magnetism and there was optics on the other. After Maxwell there stood only electromagnetism and its corollary, namely propagating electromagnetic waves, which provide the explanation of what light is. Yet, while completing the classical building, Maxwell opened into it a small window, through which a completely different vision of Physics slept in, first silently and almost reluctantly, to develop then, over the short period of just a few years, into a revolutionary reframing of the whole fabrics of physical thinking.
1.2.1 Maxwell Equations
In his scientific masterpiece [2], Maxwell summarized into four differential equations for the electric field E(t, x) and the magnetic field B(t, x) all the laws of electricity and magnetism that had been explored in the course of the XIX century. When written in the standard notation of three-dimensional vector calculus, they read as follows:
· B = 0 |
(1.2.1) |
||||
1 |
∂B |
|
|||
× E + |
|
|
|
= 0 |
(1.2.2) |
c |
∂t |
||||
· E = 4πρ |
(1.2.3) |
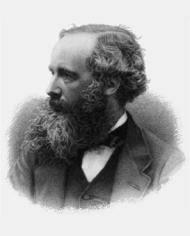
1.2 Classical Physics Between the End of the XIX and the Dawn of the XX Century |
3 |
Fig. 1.1 James Clerk Maxwell (1831–1879) had a short life of only 47 years since he died from abdominal cancer in 1879, while he was the first occupant of the Cavendish Professor Chair at Cambridge University. He was born in Edinburgh from a family belonging to the peerage, namely to the upper nobility. He studied first at the Edinburgh Academy, then at the University of Edinburgh and finally at Cambridge University, which he attended as a member of the Trinity College (from 1850 to 1856). After finishing studies in mathematics at Cambridge, Maxwell was in Scotland, where he was professor in Aberdeen. Then in 1860 he made return to England with an appointment by the King’s College in London. After his resignation from King’s in 1865 he was once again in Scotland with his wife and lived on his estates. Finally in 1871 he was appointed by Cambridge as first Cavendish professor. The Treatise on Electricity and Magnetism, which contains a complete exposition of Maxwell Equations, was published in 1873 on the basis of previous articles that had appeared starting from 1861. Actually Maxwell began to study electromagnetism already in the years 1855–1856, while he was graduating from Cambridge. The first proposal that light might be identified with an electromagnetic wave dates to an article written by Maxwell in 1864 [1]. Besides his fundamental work on Electromagnetism, Maxwell gave other fundamental contributions to mathematical physics. One was the explanation of the nature of Saturn’s rings that Maxwell demonstrated to be necessarily composed of dust of small rocky grains. The other monumental achievement of Maxwell studies is of course in the field of Thermodynamics where, independently from Boltzmann, he formulated in 1866 the kinetic theory of gases and introduced the celebrated Maxwell distribution, which gives the fraction of gas molecules moving at a specified velocity at any given temperature
1 |
∂E |
|
4π |
J |
|
||
× B − |
|
|
|
= |
|
(1.2.4) |
|
c |
|
∂t |
c |
If we introduce indices i, j, k = 1, 2, 3 for the vector components, the above equations take the following appearance:
|
|
∂i Bi = 0 |
(1.2.5) |
||
1 ∂Bi |
|
|
|||
εij k ∂j Ek + |
|
|
|
= 0 |
(1.2.6) |
c |
∂t |
||||
|
|
∂i Ei = 4πρ |
(1.2.7) |
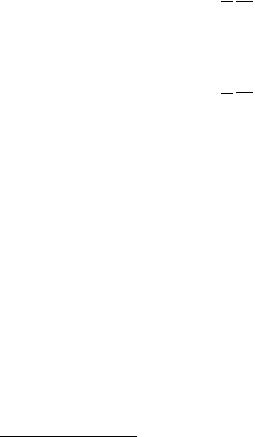
4 |
|
|
|
|
1 Special Relativity: Setting the Stage |
|||
εij k ∂j Bk − |
1 ∂Ei |
= |
4π |
J i |
(1.2.8) |
|||
c |
|
∂t |
|
c |
where, in both notations, ρ denotes the electric charge density and J i the electric charge current.
The number c appearing in the above equations has the dimension of a velocity and the genius of Maxwell led him to think that it was just the speed of light. His guess was motivated by the observation that in the vacuum, ρ = J i = 0, namely in regions where there are neither charges nor currents, by taking a further derivative× of (1.2.2) one obtains that all three components of the electric field E satisfy the d’Alembert propagation equation with velocity c:
1 ∂2 |
|
2E = c2 ∂t2 E |
(1.2.9) |
With a similar procedure one obtains that in the vacuum, also the magnetic field satisfies the same propagation equation:
1 ∂2 |
|
2B = c2 ∂t2 B |
(1.2.10) |
Hence Maxwell concluded that there are electromagnetic waves and he rightly guessed that visible light consists of nothing else but electromagnetic waves belonging to a particular region of the possible frequency spectrum. The first experimental detection of electromagnetic waves was done by Heinrich Rudolf Hertz1 in the mid eighties of the XIX century, already after the death of Maxwell.
1.2.2 Luminiferous Aether and the Michelson Morley Experiment
To the mind of XIX century physicists, the pillar of whose thinking was Newtonian mechanics, the detection of electromagnetic waves posed a severe problem. All the waves they knew corresponded to the propagation of oscillations of some mechanical medium. Hence a so far unknown medium, pervading the whole Universe, had to exist, whose propagating oscillations the humans perceived as electromagnetic waves. Such a substance was named Luminiferous aether, following an old idea dating back to Newton himself. The seed of disruption of Newtonian physics was already contained in Maxwell equations, since, as Lorentz demonstrated few years
1Heinrich Rudolf Hertz, had a short life. He died in Bonn in 1894 at the age of thirty six. He was born in Hamburg in 1857. In his laboratory at the University of Karlsruhe, where he had been appointed full professor, Hertz constructed the first dipole antennas, both transmitter and receiver and in this way produced the first radio waves demonstrating the existence of the electromagnetic waves implied by Maxwell theory.

1.2 Classical Physics Between the End of the XIX and the Dawn of the XX Century |
5 |
Fig. 1.2 The Michelson Morley interferometer experiment. Albert Abraham Michelson (1852–1931) obtained the American citizenship and lived most of his life in the States, but he was born in Prussia in a Jewish family. He was the first American to get a Nobel Prize for science, which he obtained in 1907. The initial scientific career of Michelson developed in the American Navy which he left in 1881 to become professor of Physics in Cleveland, Ohio, after having visited several European Univerisities. Edward Williams Morley (1838–1923) was also American, being born in Newark, New Jersey. He held a chair as professor of Chemistry at the Case Western Reserve University in Cleveland. There, together with Michelson, he constructed the famous interferometer experiment. The Michelson Morley apparatus is conceptually identical to the modern interferometers, devised as detectors of gravitational waves. It aimed instead at measuring the motion of the Earth with respect to the luminiferous aether. The absolutely negative result of this experiment was a puzzle which could be resolved only by the theory of Special Relativity
later, they are not invariant against the transformations of the Galilei group, that is the foundation stone on which the whole Newtonian building stands. Yet in the mid 18-eighties this fact was still unnoticed. So it was concluded that the existence of the Luminiferous aether was a logical necessity and it was also concluded that the aether provided the means of defining an absolute reference frame, that one where aether is at rest. In 1887 the two American scientists, Michelson and Morley constructed their interferometric apparatus aimed at measuring the velocity of the Earth with respect to the aether (see Fig. 1.2). Indeed since Earth moves, the speed of light cannot be the same in all directions and at all times throughout the year. In some directions and at some times, light goes against the Earth movement, in some other almost along it. Hence one should necessarily measure interference fringes due to this fact. Yet subtle is the Lord, according to a famous phrasing of Einstein, and no such fringes were detected. The speed of light seemed to be the same in all directions and at all times. This negative result was received as a puzzle by the scientific community and caused a lot of thinking. In particular it motivated Hendrik Antoon