
John Wiley & Sons - 2004 - Analysis of Genes and Genomes
.pdf
158 |
POLYMERASE CHAIN REACTION 4 |
|
|
and each will act as a template for the binding of new primers. In cycle 2, primer binding to both the original template strands and the strands synthesized during cycle 1 will occur. Primer binding to the original template strands will result in the formation of the same products that were made during cycle 1.
Table 4.1. The theoretical yield of correctly formed double-stranded target DNA molecules during a PCR experiment beginning with a single target DNA molecule
Cycle |
Double-stranded |
Larger double- |
Total DNA |
number |
target molecules |
stranded molecules |
strands replicated |
|
|
|
|
1 |
0 |
2 |
2 |
2 |
0 |
4 |
4 |
3 |
2 |
6 |
8 |
4 |
8 |
8 |
16 |
5 |
22 |
10 |
32 |
6 |
52 |
12 |
64 |
7 |
114 |
14 |
128 |
8 |
240 |
16 |
256 |
9 |
494 |
18 |
512 |
10 |
1 004 |
20 |
1 024 |
11 |
2 026 |
22 |
2 048 |
12 |
4 072 |
24 |
4 096 |
13 |
8 166 |
26 |
8 192 |
14 |
16 356 |
28 |
16 384 |
15 |
32 738 |
30 |
32 768 |
16 |
65 504 |
32 |
65 536 |
17 |
131 038 |
34 |
131 072 |
18 |
262 108 |
36 |
262 144 |
19 |
524 250 |
38 |
524 288 |
20 |
1 048 536 |
40 |
1 048 576 |
21 |
2 097 110 |
42 |
2 097 152 |
22 |
4 194 260 |
44 |
4 194 304 |
23 |
8 388 562 |
46 |
8 388 608 |
24 |
16 777 168 |
48 |
16 777 216 |
25 |
33 554 382 |
50 |
33 554 432 |
26 |
67 108 812 |
52 |
67 108 864 |
27 |
134 217 674 |
54 |
134 217 728 |
28 |
268 435 400 |
56 |
268 435 456 |
29 |
536 870 854 |
58 |
536 870 912 |
30 |
1073 741 764 |
60 |
1073 741 824 |
|
|
|
|
4.1 PCR REACTION CONDITIONS |
159 |
|
|
However, primer binding to the DNA strands produced in cycle 1, followed by replication, will result in the formation of a DNA strand with both a defined 5 -end and a defined 3 -end. This occurs because DNA replication will terminate when there is no more DNA sequence to copy. Thus, at the end of cycle 2, two DNA strands are formed (shown in purple) that have a defined 5 - and a defined 3 -end. These are, however, base-paired to DNA fragments that have ill defined 3 -ends. Again, the products from cycle 2 of the PCR process will go forward into cycle 3 and, again, each DNA strand can be used as a template for primer binding. At the end of cycle 3, two double-stranded DNA molecules are formed that have 5 - and 3 -ends beginning and ending at the positions of primer binding within the original target DNA sequence. These are the boxed sequences shown in Figure 4.3.
Beyond cycle 3 of the PCR, the repeating cycles of heat denaturation, primer annealing and extension will result in the exponential accumulation of specific target fragments of DNA (Table 4.1). After 25 cycles, typical for many PCR experiments, an amplification of about 30 million-fold is expected, and amplifications of this order are actually attained in practice. All PCR reactions are ‘contaminated’ with small quantities of DNA fragments that have incorrectly formed ends, but the massive amplification of specific DNA fragments means that these are almost insignificant.
There are several factors that need to be considered when attempting to amplify a specific DNA sequence using PCR. These include the choice of DNA polymerase used, the template DNA, the overall reaction conditions and the sequence of the oligonucleotide primers. We will briefly discuss each of these issues below with particular reference as to how genetic engineering experiments may be influenced, but interested readers are directed to a variety of texts that deal with the practical aspects of PCR in greater depth (McPherson and Møller, 2000; Innis, Gelfand and Sninsky, 1999).
4.1PCR Reaction Conditions
A typical PCR experiment will contain the following components:
•DNA (0.01 –0.1 µg)
•primer 1 (20 pmol)
•primer 2 (20 pmol)
•Tris-HCl (20 mM, pH 8.0)
•MgCl2 (2 mM)
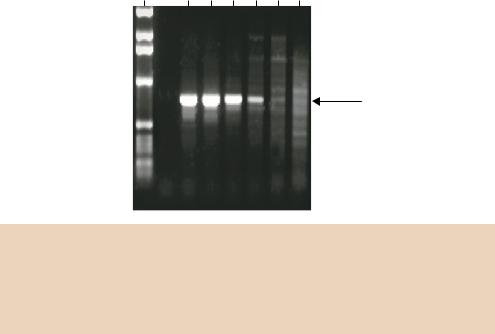
160 |
POLYMERASE CHAIN REACTION 4 |
|
|
•KCl (25 mM) or KCl (10 mM) and (NH4)2SO4 (10 mM)
•deoxynucleotide triphosphates (50 µM each of dATP, dCTP, dGTP, dTTP)
•thermostable DNA polymerase (2 units)
•a total reaction volume of 50 – 100 µL.
We will discuss the polymerase, the input DNA and the primers in greater detail below. Of the reagent components of the reaction (Tris, KCl and MgCl2), the concentration of magnesium ions in the reaction plays a significant role in the success of a PCR reaction (Figure 4.4). Magnesium is required for the DNA polymerase to function, but the specificity of any particular PCR reaction is dependent upon the concentration of magnesium used. At low concentrations of magnesium, the reaction fails because the polymerase is insufficiently active. At high concentrations of magnesium, the reaction loses specificity and multiple products are produced. The optimum magnesium concentration needs to be determined empirically for each separate PCR primer set, but will usually be in the range of 1 –5 mM. The buffer and salt components of the reaction (Tris and KCl) are usually held constant, although some protocols reduce the level of KCl to encourage DNA polymerase to remain on the template for longer and achieve a greater length of amplified product (Foord and Rose, 1994).
mM Mg2+
M 1.5 2.0 2.5 3.0 3.5 4.0
Specific
PCR product
Figure 4.4. The effect of magnesium concentration on the efficiency and specificity of a PCR experiment. A PCR experiment was set up containing different concentrations of magnesium chloride. After PCR, the products were separated on an agarose gel and stained with ethidium bromide. The size of the PCR product obtained was compared with a set of DNA size standards (M). Reproduced from Critical Factors for Successful PCR by permission, of Qiagen GmbH

4.1 PCR REACTION CONDITIONS |
161 |
|
|
Once the PCR reaction has been set up, it is often covered with a layer of mineral oil to prevent evaporation of the sample during heating – alternatively, a PCR machine with a heated lid will prevent evaporation – before being subjected to the varying temperatures that will promote the denaturation, annealing, and extension components of a PCR cycle. Typical cycling conditions for a PCR experiment might be
•94 ◦C, 30 s – denaturation
•60 ◦C, 30 s – annealing
•72 ◦C, 1 min – extension.
The denaturing and annealing steps are relatively short, but are sufficient to allow breaking and reforming of the hydrogen bonds between DNA strands. Early protocols included an initial denaturing step (94 ◦C, 2 min) to ensure that the initial template DNA was fully single stranded. This is usually not included now since long exposures to high temperature will induce nicks in the template DNA. The length of the amplified PCR product dictates the time allowed for the extension step of the reaction. Most of the polymerases used in PCR will replicate DNA in vitro at a rate of approximately 500 –1000 bp min−1 (Table 4.2). The time that the PCR experiment is incubated at the extension temperature is adjusted depending upon the length of the expected product.
Table 4.2. The properties of different types of thermostable DNA polymerase
|
|
Taq DNA |
Tfl DNA |
Pfu DNA |
Tli DNA |
Tgo DNA |
||||||
|
|
polymerase polymerase polymerase |
polymerase |
polymerase |
||||||||
|
|
|
|
|
||||||||
|
Organism |
Thermus |
Thermus Pyrococcus Thermococcus Thermococcus |
|||||||||
|
|
aquaticus |
flavus |
furiosis |
litoralis |
gorgonarius |
||||||
|
|
|
|
|
|
|
|
|
|
|
|
|
|
PCR optima: |
|
|
|
|
|
|
|
|
|
|
|
|
Extension time/kb (min) |
|
1 |
|
1 |
|
2 |
|
2 |
|
2 |
|
|
Extension temperature (◦ C) |
70 |
– 75 |
70 |
– 74 |
70 |
– 75 |
70 |
– 75 |
70 |
– 75 |
|
|
[Magnesium] (mM) |
1 |
– 4 |
1 |
– 4 |
2 |
– 4 |
2 |
– 4 |
2 |
– 4 |
|
|
pH @ 25 ◦ C |
7.0 |
– 7.5 |
7.0 |
– 7.5 |
8.0 |
– 9.0 |
7.0 |
– 7.5 |
7.0 |
– 7.5 |
|
|
[dNTP] (mM) |
40 – 200 |
40 – 200 |
40 – 200 |
40 – 200 |
200 |
|
|||||
|
[Primers] (mM) |
0.1 |
– 1.0 |
0.1 |
– 1.0 |
0.1 |
– 1.0 |
0.1 |
– 1.0 |
0.1 |
– 1.0 |
|
|
5 –3 exonuclease activity |
Yes |
Yes |
No |
No |
No |
||||||
|
3 –5 exonuclease activity |
No |
No |
Yes |
Yes |
Yes |
||||||
|
Approximate error rate |
5.0 × 10−4 |
5 × 10−5 |
1.3 × 10−6 |
2.8 × 10−6 |
2.0 × 10−6 |
||||||
|
(errors per base replicated) |
|
|
|
|
|
|
|
|
|
|
|
|
PCR product ends |
3 -A |
3 -A |
Blunt |
Blunt |
Blunt |
162 |
POLYMERASE CHAIN REACTION 4 |
|
|
The number of PCR cycles that are performed during an individual experiment depends upon both the amount of initial DNA template in the reaction and the amount of DNA required after the amplification process. In general, to avoid replication errors (see below), as few cycles as possible will be performed. This will usually be in the range of 17 –25 cycles. After the cycles are complete, many PCR protocols include a final extension step (72 ◦C for 5 min) to ensure that all of the DNA in the reaction has been replicated into a double-stranded form. This final extension may also increase cloning efficiency of the PCR products (Li and Guy, 1996).
4.2Thermostable DNA Polymerases
The bacterium Thermus aquaticus was first discovered in several hot springs in the Yellowstone National Park (Brock and Freeze, 1969). It has since been found in thermal habitats throughout the world. The organism has a temperature tolerance range between about 50 and 80 ◦C, and its optimum growth temperature is around 70 ◦C. Taq DNA polymerase is a monomeric enzyme with a molecular weight of 94 kDa that is isolated from the organism (Chien, Edgar and Trela, 1976; Lawyer et al., 1989). The enzyme itself is thermostable; it replicates DNA at 74 ◦C and remains functional even after incubation at 95 ◦C. The enzyme includes a 5 to 3 polymerase activity and a 5 to 3 exonuclease activity, but it lacks a 3 to 5 exonuclease (proofreading) activity. The lack of proofreading activity means that if an incorrect base is inserted into the extending polynucleotide chain, it cannot be removed and consequently Taq DNA polymerase is error prone and will introduce mutations into amplified PCR products. In in vitro assays, Taq DNA polymerase misincorporates a base every 104 –105 bases replicated (Barnes, 1992; Cline, Braman and Hogrefe, 1996). The wide variation in the estimated error rate is due, in part, to the methods used to assess the introduction of mutations. At its worst estimated level, with an error rate of 1 × 10−4 errors per base pair replicated, if a 1 kb sequence is amplified for 25 cycles with Taq then approximately 10 per cent of the amplified products will contain mutations. However, since mutations occurring in one cycle will be amplified in later cycles, the actual mutational frequency may vary from experiment to experiment. This level of error introduction does not, however, affect the affect the outcome of a PCR experiment. If the PCR is being performed merely to identify the presence or absence of a gene within a particular target DNA molecule, then the success of the reaction will not be affected by the introduction of errors into the amplified sequence. However if the amplified gene is to be studied functionally, then PCR errors
4.2 THERMOSTABLE DNA POLYMERASES |
163 |
|
|
may significantly affect the experiment. The problem of error introduction does mean, however, that PCR products should be subjected to DNA sequence analysis before they are used in cloning experiments. Additionally, several independent PCR clones should be chosen to ensure that the sequence obtained is representative.
Another functional aspect of Taq DNA polymerase that impinges upon the sequence of the final PCR product is the tendency of the enzyme to incorporate
adeoxynucleotide (often an adenosine) in a template-independent manner on the 3 -end of the newly synthesized DNA strand. A consequence of this activity is that PCR products produced by Taq do not have blunts ends, but have
asingle 3 A residue overhang. This property has been exploited to aid the cloning of PCR products (see below).
Since the discovery of Taq DNA polymerase, a number of other thermostable DNA polymerases have been described and have been used in PCR experiments. While Taq remains the most commonly used of the thermostable enzymes for PCR, polymerases from other sources have different properties that make them useful for certain applications (Table 4.2).
Some of the other thermostable DNA polymerases, e.g. Pfu polymerase isolated from the organism Pyrococcus furiosis, do possess a 3 to 5 exonuclease proofreading activity, and so their mutation rate is reduced. Using the example above, if a 1 kb segment of DNA is amplified over 25 cycles with Pfu polymerase, then only 0.1 per cent of the amplified products will contain mutations. Additionally, some of the other thermostable DNA polymerases
produce blunt-ended PCR products (Table 4.2).
The 5 to 3 exonuclease activity of Taq DNA polymerase means that the enzyme is able to degrade the oligonucleotide primers within the PCR reaction. This is particularly relevant during the first denaturing step of cycle 1, when the oligonucleotides are not bound to the DNA template, and the polymerase is free
in solution. During the first heating cycle, the temperature of the PCR mix rises from room temperature (or 4 ◦C if the reaction was set up on ice) to 94 ◦C. This means that, at some point, the temperature within the tube will be 72 ◦C – the optimum for the polymerase – but the enzyme will be unable to replicate DNA since none of the oligonucleotides are bound to the template DNA. Passing through the temperature of the enzyme without replication occurring will tend to result in primer degradation, and subsequent inefficient PCR. To overcome this problem, and to prevent non-specific PCR products being synthesized
prior to cycling, Taq DNA polymerase can be added to the reaction mix already at 94 ◦C. This ‘hot start’ increases both the yield and specificity of the PCR reaction. Alternatively, Taq DNA polymerase can be mixed with a specific antibody that binds to the enzyme and inhibits its activity (Kellogg et al., 1994).
164 |
POLYMERASE CHAIN REACTION 4 |
|
|
The antibody – enzyme complex inhibits replication at low temperatures, but the complex irreversibly dissociates at high temperature, after which the enzyme is unhindered in its function.
The existence of a number of thermostable DNA polymerases with varying properties has led to the ‘blending’ of polymerases for specific functions. For instance, Taq DNA polymerase produces high yields of PCR product, but is error prone and has a maximum PCR product size of about 5 –7 kbp. Pfu DNA polymerase, on the other hand, is much less error prone, but still has difficulty efficiently producing PCR products over 7 kbp. A mixture of the two polymerases (15 parts Taq and 1 part Pfu) has been found to efficiently amplify DNA fragments up to 35 kbp in length with high fidelity (Barnes, 1994).
4.3Template DNA
Almost any DNA sample can be used as a template for a PCR reaction, including linear, closed-circular and supercoiled plasmid DNA, genomic DNA, cDNA etc. The source of the DNA is immaterial, since PCR is merely a sequence directed event. The only requirement is that the primer binding sites, and the sequence between them, are intact. DNA samples over 7000 years old have successfully been used in PCR experiments (Lawlor et al., 1991).
In the cases we have looked at so far, and for the sake of clarity, we have considered the amplification of a single target DNA molecule. While this is certainly achievable (Li et al., 1988), in practice larger amounts of DNA are commonly used. When very small amounts of DNA are used, contamination of the PCR reaction can become a major problem. The massive amplification properties of PCR mean that even the slightest DNA contamination can ruin an experiment. Contamination may come from a variety of sources, including the researcher who is performing the experiment, the tubes and tips that are used to set up the reaction and even the enzymes and buffers used in the reaction itself. In a typical PCR experiment between 0.1 and 1 µg of genomic DNA would be added to the reaction so that a relatively low number of PCR cycles can be performed and still sufficient material produced for further experiments. This reduces the likelihood of contaminating sequences interfering with the desired amplification. How many copies of the target sequence does this amount of DNA correspond to? If you add 1 µg of human genomic DNA to a PCR reaction, this is equivalent to 1 × 10−6/(6.4 × 109 × 650) = 2.4 × 10−19 mol, since human DNA contains approximately 6.4 × 109 bp of DNA and the average molecular weight
4.4 OLIGONUCLEOTIDE PRIMERS |
165 |
|
|
of a base pair is 650 Da. Therefore 1 µg of human DNA corresponds to 2.4 × 10−19 mol × 6 × 1023 (Avogadro’s number) = approximately 144 000 molecules. That is, a single gene will be represented 288 000 times in 1 µg of genomic diploid DNA. An eight million-fold amplification of a 1000 bp segment of this genomic DNA, that should be achieved after 25 PCR cycles, will generate almost 10 µg of that 1000 bp DNA fragment. This amount is sufficient that a small fraction of the PCR reaction (typically 10 µL of a 50 µL total reaction) can be readily visualized by ethidium bromide staining after agarose gel electrophoresis. This amount is also more than sufficient for most cloning procedures and DNA sequencing protocols.
4.4Oligonucleotide Primers
The success, or otherwise, of a PCR experiment is almost wholly dependent upon the oligonucleotide primers. The primers need to be designed such that one recognizes the sense strand of the DNA to be replicated (i.e. is the same sequence as the antisense strand) while the other recognizes the antisense strand of the target DNA (i.e. is the same sequence as the sense strand). This is shown in Figure 4.5. Typically, primers will have the following characteristics.
•They will be between 17 and 30 nucleotides in length – sufficient to allow unique annealing to a single sequence within a genome.
•They will have a GC content of approximately 50 per cent.
•The annealing temperatures of the pair of primers – calculated from the equation 2(AT) + 4(GC) – used in a single experiment should be approximately equal.
•Sequences with long runs of a single nucleotide should be avoided to prevent binding of the primer to repetitive sequences in the target DNA.
•Individual primers should not contain sequences that are complementary. For example, a primer of the sequence 5 -GAGATCGATGCATCGATCTC-
3 may appear a good choice for a PCR primer (20 nucleotides long, 50 per cent GC content and not containing repetitive sequences), but it is palindromic and will form a hair-pin structure if the 5 -end binds to the 3 -end. This secondary structure is undesirable, and will effectively remove the primer from the PCR reaction so amplification of the target will not occur.
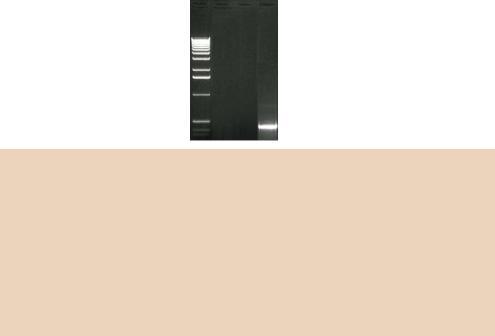

4.4 OLIGONUCLEOTIDE PRIMERS |
167 |
|
|
be replicated during the first cycles of the PCR reaction:
5′-GATCGATCGATACGTGATCC-3′
3′-GCACTAGGATCGATCGATGC-5′
PCR
5′-GATCGATCGATACGTGATCCTAGCTAGCTACG-3′
3′-CTAGCTAGCTATGCACTAGGATCGATCGATGC-5′
Most primers that conform to the above criteria can be made to work in PCR experiments, but a number of freely available software packages, including several of which that are web based, have been written to aid the primer design process (Rozen and Skaletsky, 1998).
4.4.1 Synthesis of Oligonucleotide Primers
Most oligonucleotides are made on commercial nucleic acid synthesizers using phosphoramidite chemistry. Oligonucleotide phosphoramidite synthetic chemistry was introduced nearly 20 years ago (McBride and Caruthers, 1983). The building blocks used for synthesis are DNA phosphoramidite nucleosides (sometimes called monomers). These are modified to prevent branching or other undesirable side reactions from occurring during synthesis. They are modified at the 5 -end (with a dimethoxytrityl group) and at the 3 -end (with a β-cyanoethyl protected 3 -phosphite group), and may also include additional modifiers to protect reactive primary amines in the nucleoside ring structure.
The phosphoramidite approach to oligonucleotide synthesis proceeds in four steps that are schematically outlined in Figure 4.6. Automated synthesis is performed on solid supports, usually polystyrene. The polystyrene is loaded into a small column that serves as the reaction chamber. A loaded column is attached to reagent delivery lines on a DNA synthesizer and the chemical reactions proceed under computer control. Bases are added to the growing chain in a 3 to 5 direction (opposite to enzymatic synthesis by DNA polymerases). Synthesis is begun using polystyrene that is already derivatized with the first base, which is attached via an ester linkage at the 3 -hydroxyl (Figure 4.6(a)).
Primer synthesis initiates with cleavage of the 5 -trityl group (Figure 4.6(b)) by brief treatment with acid. Monomer activated by tetrazole is coupled to the available 5 -hydroxyl (Figure 4.6(c)) and the resulting phosphite linkage is oxidized to phosphate by treatment with iodine (Figure 4.6(d)). This completes one ‘cycle’ of oligonucleotide synthesis.