
John Wiley & Sons - 2004 - Analysis of Genes and Genomes
.pdf
218 GENE IDENTIFICATION 6
6.5Phage Display
Phage display is a powerful technique for identifying peptides or proteins that bind to other molecules. DNA encoding a specific gene or a library of cDNA fragments is cloned into the M13 bacteriophage genome in frame with gene 3 (see Figure 3.15), encoding the minor coat protein pIII. This results in the expressed peptide or protein being displayed on the surface of the phage particle as a fusion to endogenous pIII (Smith, 1985). Phage display thus creates a physical linkage between a library of random peptide sequences to the DNA encoding each sequence, and allows for the rapid identification of peptide ligands for a variety of target molecules (antibodies, enzymes, cellsurface receptors etc.) by an in vitro selection process called panning. In its simplest form, panning is carried out by incubating a library of phage displayed peptides with a plate (or bead) coated with the target, washing away the unbound phage, and eluting the specifically bound phage. The eluted phage is then amplified and taken through additional binding/amplification cycles to enrich the pool in favour of binding sequences. After three or four rounds, individual clones are characterized by DNA sequencing. Phage display has been used to identify peptides that bind to receptors, substrates or inhibitors of enzymes, epitopes, improved antibodies, altered enzymes and cDNA clones (O’Neil and Hoess, 1995). Random peptide libraries displayed on phage have been used in a number of applications, including epitope mapping, mapping protein – protein contacts and identification of peptide mimics of non-peptide ligands. For example, Beck et al. used a displayed random peptide library to select for substrates for the HIV-1 protease (Beck et al., 2000). They were able to identify peptides that could be cleaved over 200 times more efficiently than the wild-type sequence, and may provide a basis for designing highly specific inhibitors of the protease itself.
6.6Two-hybrid Screening
The basis for two-hybrid interaction screening is the modular nature of eukaryotic RNA polymerase II transcriptional activator proteins. They consist of at least two protein domains – a sequence-specific DNA binding domain (DBD) that allows them to bind to the promoters of specific genes, and an activation domain (AD), which serves as a site of recruitment for a number of protein complexes that result in the transcription of the gene. As early as 1985, it had been shown that fusion proteins composed of the DNA binding domain from one protein fused to the activation domain of another could function
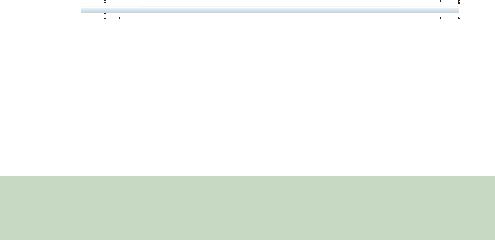
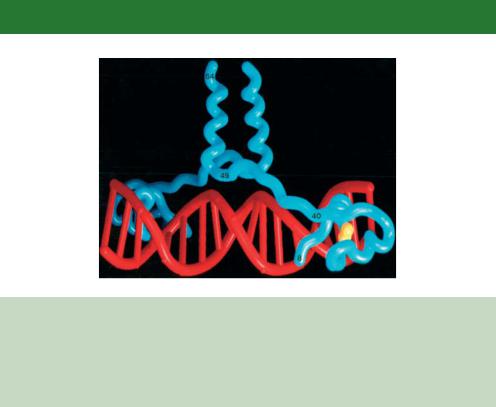
220 GENE IDENTIFICATION 6
Figure 6.7. The structure of the amino-terminal 65 amino acids of Gal4p bound to DNA as determined by X-ray crystallography (Marmorstein et al., 1992). The DNA (red) is contacted by one-half of the protein dimer on each side of the double helix. The DNA recognition sequences on the protein fold into a Zn(II)2Cys6 binuclear cluster, which is held by two zinc atoms (yellow). The start of the coiled-coil dimerization surface (residues 49–64) is observable within the crystal structure and extends away from the helix
the UASG – upstream activation sequence for galactose. This sequence (5 - CGGN11CCG-3 , where N can be any nucleotide) is found, often in multiple copies, 50 –200 bp upstream of the transcriptional start site of galactose regulated genes (Giniger, Varnum and Ptashne, 1985).
•Amino acids 65 –94 form a coiled-coil motif that is responsible for the dimerization of the protein.
•Amino acids 768 –881 form the major AD. Like all yeast activation domains, this region is acidic. That is, it contains a preponderance of negatively charged amino acids (Ma and Ptashne, 1987c). Acidity is, however, not the sole determinant defining an activation domain or its relative strength. Activating domains appear to be largely unstructured, with acidic and hydrophobic amino acids contributing to the overall activating potential (Triezenberg, 1995). Gal4p also possesses an additional AD located between amino acids 148 and 196. This region is a weak activator and its relevance to contributing to the overall function of the protein is not understood.
•Amino acids 851 –881 also form the binding site for a transcriptional repressor, Gal80p, which functions to modulate the activity of Gal4p (Ma and Ptashne, 1987a; Johnston, Salmeron and Dincher, 1987). The binding
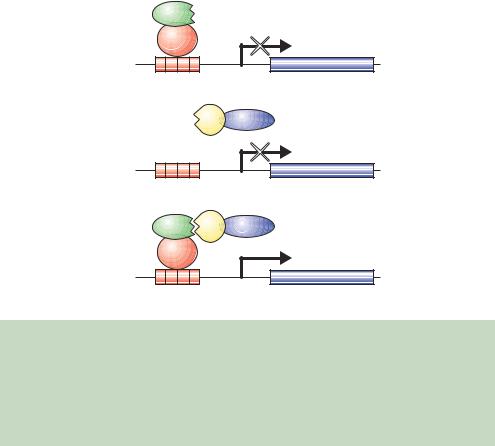
6.6 TWO-HYBRID SCREENING |
221 |
|
|
of Gal80p to this site appears to maintain the carboxy-terminal end of Gal4p in an orientation that cannot activate transcription.
The basis for interaction screening using transcription factors arises from the observations that the expression of just the DBD or just the AD within a cell is insufficient to elicit transcriptional activation (Ma and Ptashne, 1987b). A general scheme for screening in this way is shown in Figure 6.8. The Gal4p DBD is sufficient for binding to the UASG, but the lack of an AD means that it will not recruit the RNA polymerase or activate gene expression. Similarly, the Gal4p AD alone will not activate gene expression because the protein cannot be targeted to particular genes. However, if the DBD and AD can be linked in some way, e.g. through the protein –protein interactions of polypeptides fused to them, then a functional activator protein in which the DBD is linked to the AD will be formed and gene activation will occur.
(a)
X
Gal4p
DBD
Reporter gene
UASG
(b)
YGal4p AD
Reporter gene
UASG
(c)
X |
Y Gal4p AD |
Gal4p |
|
DBD |
|
|
Reporter gene |
UASG |
|
Figure 6.8. The two-hybrid screen. (a) The expression of a hybrid protein composed of the DNA binding domain (DBD) of Gal4p and a bait protein (X) is able to bind to the promoter of the reporter gene but cannot activate it. (b) The expression of another hybrid protein comprising the Gal4p activation domain (AD) and a prey protein (Y) cannot activate transcription because it is unable to bind DNA. (c) The expression of the two hybrid proteins in which the bait and prey interact with each other results in the formation of a functional activator protein and expression of the reporter gene occurs
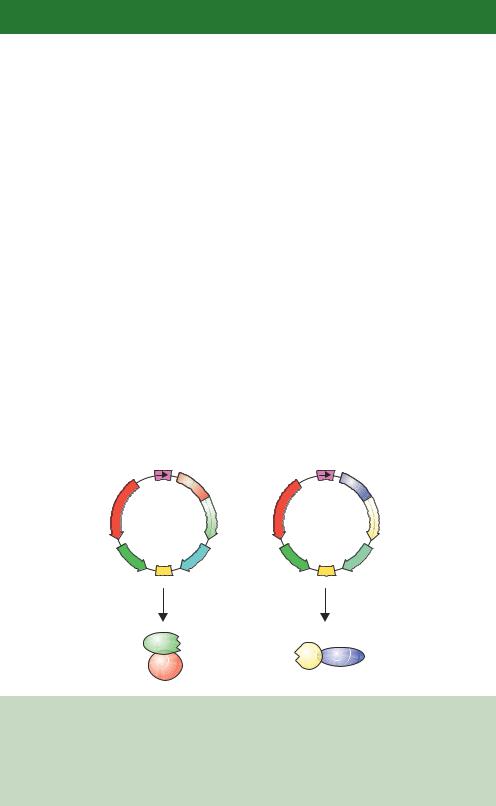
222 GENE IDENTIFICATION 6
In 1989 Stanley Fields and Ok-kyu Song first used this split transcriptional activator system to detect protein –protein interactions in yeast cells (Fields and Song, 1989). They constructed yeast expression plasmids in which the gene sequences encoding either the Gal4p DBD or the Gal4p AD were fused to the gene sequences encoding other polypeptides (Figure 6.9). These result in the production of hybrid proteins bearing either the Gal4p DBD or AD. When expressed in cells on their own, neither is able to activate gene expression. Additionally, if both fusion proteins are expressed in the same cell but the polypeptides fused to the DBD and AD do not interact with each other, gene activation will not occur. If, however, the polypeptides fused to the DBD and AD do interact, then the DBD and AD functions will be brought together through this protein –protein interaction and gene activation will occur (Figure 6.8). That is, only if the two hybrid proteins are able to interact with each other will gene expression occur.
Although the activation of the galactose metabolizing genes naturally regulated by the UASG could be used to detect fruitful protein –protein interactions (Fields and Song, 1989), it is more convenient to analyse the expression of a more easily detectable gene product. One of the advantages of performing interaction screening in yeast is that a large number of reporters are available for use in yeast – these include those shown in Table 6.2 and a number of
ADH1 |
|
ADH1 |
|
promoter |
GAL4 |
promoter |
GAL4 |
|
|
||
|
−147) |
|
(768−881) |
AMPR |
X |
AMPR |
Y |
Gal4- |
Gal4-AD |
||
plasmid |
|
plasmid |
|
2m |
TRP1 |
2m |
URA3 |
ori |
ori |
||
Produces |
Produces |
||
X |
|
|
|
Gal4p |
Y |
Gal4p AD |
|
|
|
DBD |
|
|
Figure 6.9. Plasmids to generate the DNA binding and activation domain fusions required for the two-hybrid screen. One plasmid produced the DBD fusion hybrid and another produced the AD fusion hybrid. The genes for both hybrid proteins are expressed from a strong constitutive promoter (ADH1) and are contained on high-copy-number plasmids bearing the 2µ sequence. Different nutritional genes (TRP1 and URA3) allow for plasmid selection in yeast cells
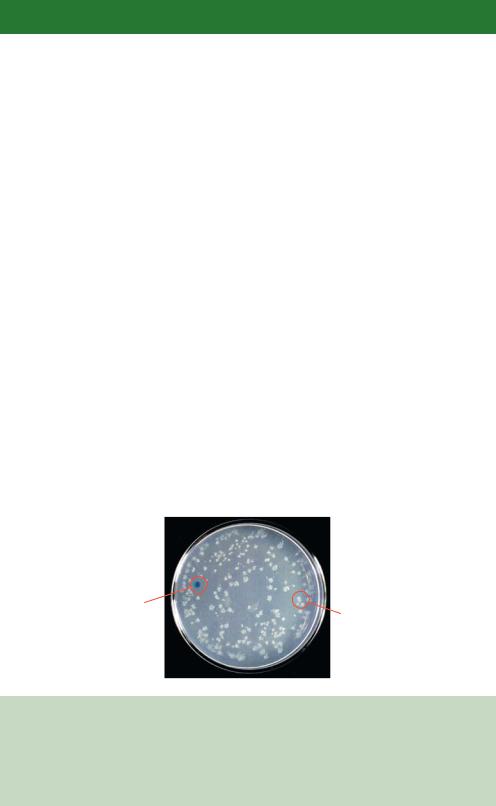
224 GENE IDENTIFICATION 6
nutritional genes that can be selected for in deficient hosts. To make use of the reporter gene, the UASG DNA sequence is cloned upstream of a reporter gene so that its expression is dependent on the association of the split DBD and AD. For example, the expression of the E. coli lacZ gene in eukaryotic cells, including yeast, produces a functional β-galactosidase enzyme. The production of this enzyme can be detected using methods similar to those we have already discussed for insertional inactivation in cloning vectors (Chapter 3) by, for example, producing a blue colour when grown on plates containing XGal. Yeast cells in which the UASG has been placed upstream of the lacZ coding sequence will produce β-galactosidase in a galactose-dependent fashion through the action of Gal4p (Yocum et al., 1984).
As originally described, the two-hybrid system was used to detect specific interactions between two known proteins, sometimes called the bait and prey, in yeast (Fields and Song, 1989). The screen was performed in a yeast strain that was deleted for the wild-type copies of the GAL4 and GAL80 genes, and the fruitful interaction of the bait and prey was measured using a lacZ reporter containing Gal4p binding sites within its promoter. The system was, however, soon adapted to screen cDNA libraries (Chien et al., 1991) and modified to operate in higher-eukaryotic cells (Luo et al., 1997) and in bacteria (Hu, Kornacker and Hochschild, 2000). To screen cDNA libraries, in-frame cDNA fusions are made to the AD. The library is then transformed into yeast cells together with the Gal4p DBD –bait fusion. Interacting partners can be isolated from the library as those that activate the expression of the reporter gene (Figure 6.10). Screening must be performed with the library fused to the AD,
Interacting |
Non- |
|
partner |
||
interacting |
||
|
||
|
partner |
Figure 6.10. The results of a typical two-hybrid screen. A yeast strain harbouring a UASG-lacZ reporter was transformed with two plasmids – one expressing a Gal4p DBD bait fusion and the other expressing a Gal4p cDNA library fusion. Transformants were selected and the transferred to plates containing XGal. Only one of the Gal4p cDNA library encoded proteins (circled) was able to interact with the bait to produce a blue colony. Non-interacting partners did not elicit lacZ transcription and remained white
6.6 TWO-HYBRID SCREENING |
225 |
|
|
since cDNA fusion of the Gal4p DBD gives rise to a high number of sequences that are able to activate transcription when tethered to DNA. Experiments have shown that approximately 1 per cent of random E. coli genomic DNA fragments will function as transcriptional activators when fused to the Gal4p DBD (Ma and Ptashne, 1987c). This gives an unacceptably high background level of false positives in the screen – that is, many yeast colonies will be able to activate the reporter gene even though they do not contain proteins that interact with each other.
6.6.1Problems, and Some Solutions, with Two-hybrid Screening
The two-hybrid screen is a very commonly used technique to detect protein –protein interactions (Colas and Brent, 1998). The technique does, however, suffer from a number of deficiencies. Some of these, together with some potential solutions, are listed below.
•False positives. Sequences contained within a cDNA library may encode DNA binding proteins that can circumvent the interaction with the DBD – bait protein. If the prey fusion is able to bind fortuitously to the promoter of the reporter gene, then it will promote transcription of the reporter even though it does not interact with the bait. This problem can be overcome by screening for the expression of two, or more, different reporter genes. This approach is essential if screening libraries for interacting partners, and two ways in which this can be achieved are shown in Figure 6.11. Yeast strains harbouring multiple reporter genes, which are different except for the Gal4p binding sites, have been constructed (James, Halladay and Craig, 1996). Colonies are only analysed further if all, and not just a sub-set, of the reporters are activated. An alternative approach is to perform the screen in a yeast strain expressing two different baits and a single prey (Xu, Mendelsohn and Brent, 1997). In this case, activation of only one reporter should occur, and a false positive would be assigned if both reporters become activated (Serebriiskii, Khazak and Golemis, 1999).
•Weak interactions. Some protein –protein interactions, although physiologically relevant, are very weak. Weak protein –protein interactions may not be sufficiently strong to recruit the transcriptional machinery to the promoter, and consequently the reporter gene will not be activated. It has been estimated that if the binding affinity between the bait and the prey is weaker than about 10 – 50 µM, then a transcriptional response will not be elicited (Brent and Finley, 1997).
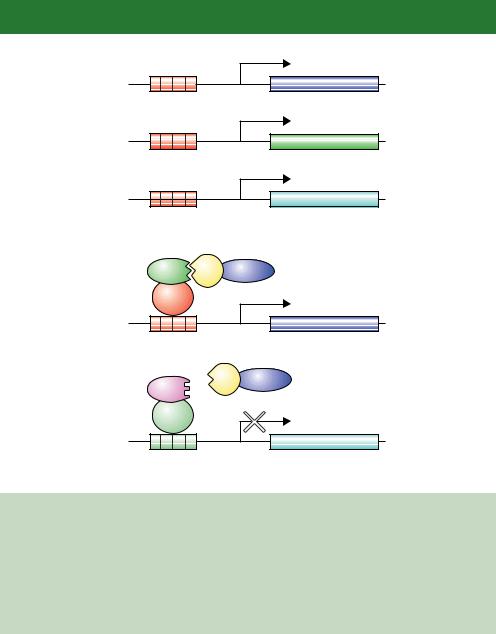
226 GENE IDENTIFICATION 6
(a)
HIS3
UASG
ADE2
UASG
lacZ
UASG
Identify His+, Ade+, blue colonies
(b)
Bait 1 |
Y |
AD |
TetR
DBD
URA3
TetOp
Y AD
Bait 2
LexA
DBD
lacZ
LexOp
Identify Ura+, white colonies
Figure 6.11. Using multiple reporters in the same cell to reduce the number of false positives in a two-hybrid screen. (a) Three different reporters each bearing Gal4p binding sites (UASG) are screened simultaneously for activity. Interactions between the bait and prey should activate all of the reporters and not a sub-set of them. (b) Screening using two different baits. A specific interaction between one of the bait proteins and the prey will result in the activation of only one of the reporter genes. This type of screening is particularly useful if the two bait proteins are closely related to each other to identify a prey that interacts specifically with only one bait (Serebriiskii, Khazak and Golemis, 1999)
•Bait protein cannot itself be a transcriptional activator. If the bait is itself an activator then it cannot be used to find interacting partners using the screens described above. Many proteins, even though they are not normally transcriptional activators, will activate transcription when they are tethered to DNA. Alternative screening systems, such as those based on RNA polymerase III transcription (Marsolier, Prioleau and Sentenac, 1997), or the split-ubiquitin system (Johnsson and Varshavsky, 1994), have been developed to assay baits that fall into this category.
6.6 TWO-HYBRID SCREENING |
227 |
|
|
•Membrane proteins. The traditional two-hybrid system relies on both the bait and prey proteins entering the eukaryotic nucleus and being able to interact there. Some proteins will not be able to do this. For example, a membrane associated protein will be tethered to the membrane and consequently unable to enter the nucleus. An alternative screening procedure, termed the SOS recruitment system, has been developed, in which the protein –protein interaction screen takes place in the plasma membrane of the yeast cell (Aronheim et al., 1997).
•Fortuitous interactions. Some proteins are able to interact within a twohybrid system even though the interaction is not physiologically relevant. For example, proteins that are normally located in different cellular compartments or at different developmental stages may be capable of interaction with each other when expressed in the same cell, but they would never normally be expressed together in their natural state. It is also possible that the bait and prey do not directly interact with each other, but that their apparent association is mediated by another cellular protein. For example, the HIV encoded protein Rev interacts in a two-hybrid screen with Rip1p, a yeast nucleoporin protein (Stutz, Neville and Rosbash, 1995), but this interaction is in fact mediated via another yeast protein, Crm1p, which is involved in nuclear export (Neville et al., 1997).
•Baits must be tested individually. A single bait can be screened using a library of interacting prey partners, but the DBD-bait fusions must be produced individually and transformed along with the library into yeast cells. This can be very time consuming if multiple baits need to be screened. Interaction mating is now widely used to screen large numbers of bait proteins (Finley and Brent, 1994). A panel of different baits are constructed and transformed into haploid yeast of one of the two mating types (a or α). A prey library is transformed into a yeast strain of the opposite mating type and the different strains are mated. The resulting diploid yeasts are then screened for reporter gene activity. This method allows for rapid screening of multiple baits in a single experiment (Colas and Brent, 1998).
The two-hybrid system offers excellent opportunities to identify proteins that interact with each other. The caveats described above mean that the technique will not work for every protein but, as we will see in Chapter 10, genome-wide protein interaction maps have been constructed using data collected from twohybrid screens (Uetz et al., 2000). Many different two-hybrid systems have been described using baits with different DBDs (e.g. those of the bacterial repressor proteins LexA or TetR) or preys with different AD sequences (e.g. the activation