
John Wiley & Sons - 2004 - Analysis of Genes and Genomes
.pdf268 |
PROTEIN PRODUCTION AND PURIFICATION 8 |
|
|
8.2.1.2The CUP1 System
Copper ions (Cu2+ and Cu+) are essential at appropriate levels, yet toxic at high levels for all living organisms. Cells must therefore maintain a proper cellular level of copper ions that is not too low to cause deficiency and not too high to cause toxicity. In S. cerevisiae, copper homeostasis consists of uptake, distribution and detoxification mechanisms (Eide, 1998). At high concentrations, copper ion detoxification is mediated by a copper ion sensing metalloregulatory transcription factor called Ace1p. Upon interaction with copper, Ace1p binds DNA upstream of the CUP1 gene (Winge, 1998), which encodes a metallothionein protein, and induces its transcription. The transcription of CUP1 is induced rapidly by addition of exogenous copper to the medium (Winge, Jensen and Srinivasan, 1998). Expression vectors harbouring the CUP1 promoter can therefore be used to induce target gene expression in a copper-dependent fashion (Mascorrogallardo, Covarrubias and Gaxiola, 1996). Unlike the GAL system, yeast cultures containing the CUP1 expression plasmid can be grown on rich carbon sources, such as glucose, to high cell density, and protein production is initiated by the addition of copper sulphate (0.5 mM final concentration) to the cultures. One potential drawback with this system is the presence of copper ions in yeast growth media, and indeed in water supplies. Therefore, the ‘off’ state in the absence of added copper may still yield significant levels of protein production.
8.2.2Pichia Pastoris
Pichia pastoris is a methylotrophic yeast, capable of metabolizing methanol as its sole carbon source. The first step in the metabolism of methanol is the oxidation of methanol to formaldehyde using molecular oxygen (O2) by the enzyme alcohol oxidase. Alcohol oxidase has a poor affinity for O2, and P. pastoris compensates for this deficiency by generating large amounts of the enzyme (Koutz et al., 1989). The promoter regulating the production of alcohol oxidase (AOX1) can be used to drive heterologous protein expression in P. pastoris (Tschopp et al., 1987) since it is tightly regulated and induced by methanol to very high levels. P. pastoris cells containing the expression vector, which is usually integrated into the genome as single or multiple copies, are grown in glycerol (growth on glucose represses AOX1 transcription, even in the presence of the methanol) to extremely high cell density prior to the addition of methanol. Once induced, target proteins may accumulate at very high levels, often in the range of 0.5 to tens of grams of protein per litre of yeast culture. For example, the expression of the gene encoding recombinant hepatitis B surface antigen results in the production of more than 1 g of the antigen from 1 L of P.
8.3 EXPRESSION IN INSECT CELLS |
269 |
|
|
pastoris cells (Hardy et al., 2000). This is much greater than could be achieved in S. cerevisiae. Additionally, in comparison to S. cerevisiae, P. pastoris may have an advantage in the glycosylation of secreted proteins. Glycoproteins generated in P. pastoris more closely resemble the glycoprotein structure of those found in higher eukaryotes (Cregg, Vedvick and Raschke, 1993).
8.2.3Schizosaccharomyces pombe
S. pombe is a single-cell eukaryotic organism with many properties similar to those found in higher-eukaryotic organisms. These properties, such as chromosome structure and function, cell-cycle control, RNA splicing and codon usage, suggest that S. pombe would make an ideal candidate for the production of eukaryotic proteins (Giga-Hama and Kumagai, 1999). Additionally, eukaryotic proteins expressed in S. pombe are more likely to be folded properly, which may reduce protein insolubility associated with the production of many proteins in E. coli. Protein production in S. pombe is usually controlled by the expression from the nmt1 (no message in thiamine) promoter (Maundrell, 1993). This promoter is active when the cells are grown in the absence of thiamine, allowing downstream transcription of genes under its control, while in the presence of greater than 0.5 µM thiamine, the promoter is turned off (Maundrell, 1990). Overall protein production levels are similar to those found in S. cerevisiae.
8.3Expression in Insect Cells
Baculoviruses are rod-shaped viruses that infect insects and insect cell lines. They have double-stranded circular DNA genomes in the range of 90 –180 kbp (Ayres et al., 1994). Viral infection results in cell lysis, usually 3 –5 d after the initial infection, and the subsequent death of the infected insect. The nuclear polyhedrosis viruses are a class of baculoviruses that produce occlusion bodies in the nucleus of infect cells. These occlusion bodies consist primarily of a single protein, polyhedrin, which surrounds the viral particles and protects them from harsh environments. Most viruses of this type need to be eaten by the insect before infection will occur, and the occlusion body protects the viral particles from degradation in the insect gut. The polyhedrin gene is transcribed at very high levels late in the infection process (2 –4 d post-infection). In cultured insect cells, the production of inclusion bodies is not essential for viral infection or replication. Consequently, the polyhedrin promoter can be used to drive target gene expression.
The baculovirus Autographa californica nuclear polyhedrosis virus (AcNPV) has become a popular tool of the production recombinant proteins in insect
270 |
PROTEIN PRODUCTION AND PURIFICATION 8 |
|
|
cells (Fraser, 1992). It is used in conjunction with insect cell lines derived from the moth Spodoptera frugiperda. These cell lines (e.g. Sf9 and Sf21) are readily cultured in the laboratory, and a scheme for constructing baculovirus recombinants is shown in Figure 8.6. The size of the baculoviral genome generally precludes the cloning of target genes directly onto it. Instead, the target gene is cloned downstream of the polyhedrin promoter in a transfer plasmid (Lopez-Ferber, Sisk and Possee, 1995). The transfer plasmid also contains the sequences of baculovirus genomic DNA that flank the polyhedrin gene, both upstream and downstream. To produce recombinant viruses, the recombinant transfer plasmid is co-transfected with linearized baculovirus vector DNA into insect cells. The flanking regions of the transfer plasmid participate in homologous recombination with the viral DNA sequences and introduce the target gene into the baculovirus genome. The recombination process also results in the repair of the circular viral DNA and allows viral replication to proceed through the re-formation of ORF1629 (a viral capsid associated protein that is essential for the production of viral particles). Recombinant viral infection can be observed microscopically by viewing viral plaques on a lawn of insect cells. Plaques containing recombinant virus will be unable to form occlusion bodies due to the lack of a functional polyhedrin protein (Smith, Summers and Fraser, 1983). Screening plaques this way is, however, technically difficult. Therefore, the transfer plasmids also usually contain the lacZ gene, or another readily observable reporter gene, which allows for the visual identification of recombinant plaques by their blue appearance after staining with X-Gal (Figure 8.6). Following transfection and plaque purification to remove any contaminating parental virus, a high-titre virus stock is prepared, and used to infect large-scale insect cell culture for protein production. The infected cells undergo a burst of target protein production, after which the cells die and may lyse.
Protein production in baculovirus infected insect cells has the advantage that very high levels of protein can be produced relative to other eukaryotic expression systems, and that the glycosylation pattern obtained is similar, but not identical, to that found in higher eukaryotes (Possee, 1997; Joshi et al., 2000). Baculoviruses also have the advantage that multiple genes can be expressed from a single virus. This allows the production of protein complexes whose individual components may not be stable when expressed on their own (Roy et al., 1997). The main disadvantages of producing proteins in this way is that the construction and purification of recombinant baculovirus vectors for the expression of target genes in insect cells can take as long as 4 –6 weeks, and that the cells grow slowly (increasing the risk of contamination) in expensive media. An alternative approach to recombinant viral genome production uses

|
|
|
|
|
|
|
|
|
|
8.3 EXPRESSION IN INSECT CELLS |
271 |
||||||
|
|
|
|
|
|
|
|
|
|
|
|
|
|
|
|
|
|
|
Baculoviral genome |
|
|
|
|||||||||||||
|
|
|
|
PPH |
|
RE |
RE |
|
|||||||||
|
|
|
RE |
|
|
|
|
|
|
|
|
|
|
|
|||
ORF603 |
|
|
|
|
|
polyH |
|
|
ORF1629 |
|
|||||||
|
|
|
|
|
|
|
|
||||||||||
|
|
|
|
|
|
|
|
|
|
Cut DNA with |
|
||||||
|
|
|
|
|
|
|
|
|
|
|
|||||||
|
|
|
|
|
|
|
|
|
|
restriction enzymes |
|
||||||
|
|
|
Linear viral genome |
|
|
|
|||||||||||
|
|
RE |
|
|
|
|
|
|
RE |
|
|||||||
|
|
|
|
|
|
|
|
|
|
|
|||||||
ORF603 |
|
|
|
|
polyH |
|
ORF1629 |
|
|||||||||
|
|
|
|
|
|
||||||||||||
|
|
|
|
|
|
PPH |
|
|
|
||||||||
|
|
|
|
|
|
|
|
|
|
|
|
||||||
ORF603 |
|
|
lacZ |
|
|
Target |
ORF1629 |
|
|||||||||
|
|
|
|
||||||||||||||
|
|
Plasmid transfer vector |
|
|
|
||||||||||||
|
|
|
|
|
|
|
|
|
|
|
|
|
|
|
|||
|
ori |
|
|
|
|
|
|
|
AMPR |
|
|
|
|||||
|
|
|
|
|
|
|
|
|
|
Recombination |
|
||||||
|
|
|
|
|
|
|
|
|
|
|
|||||||
|
|
|
|
|
|
|
|
|
|
|
|||||||
|
Recombinant viral genome |
|
|
|
|||||||||||||
|
|
|
|
|
|
|
|
PPH |
|
|
|
||||||
ORF603 |
|
|
lacZ |
|
Target |
ORF1629 |
|
||||||||||
|
|
|
|
||||||||||||||
|
|
|
|
|
|
|
|
|
|
Transfect into |
|
||||||
|
|
|
|
|
|
|
|
|
|
|
|||||||
|
|
|
|
|
|
|
|
|
|
insect cells |
|
|
|
Viral plaque expressing lacZ and target gene
Figure 8.6. The production of a recombinant baculoviral genome for the production of proteins in insect cells. The target gene is cloned under the control of the polyhedrin promoter into a transfer vector that also contains regions of the viral genome that flank the polyhedrin locus. The vector is then co-transfected into insect cells with a viral genome that has been linearized using restriction enzymes (RE) that cut in several places. Homologous recombination between the linear genome and the vector will result in formation of a functional viral genome that is capable of producing viral particles. The inclusion of lacZ in the transfer vector allows for visual screening of viral plaques to identify recombinants
272 |
PROTEIN PRODUCTION AND PURIFICATION 8 |
|
|
site-specific transposition in E. coli rather than homologous recombination in insect cells (Luckow et al., 1993). It is based on site-specific transposition of an expression cassette into a baculovirus shuttle vector (bacmid) propagated in E. coli. The bacmid contains the entire baculovirus genome, a low-copy- number E. coli F-plasmid origin of replication and the attachment site for the bacterial transposon Tn7. The bacmid propagates in E. coli as a large plasmid. Recombinant bacmids are constructed by transposing a Tn7 element from a donor plasmid, which contains the target gene to be expressed, to the attachment site on the bacmid – a helper plasmid encoding the transposase is required for this function. The recombinant bacmid can be isolated from E. coli and transfected directly into insect cells.
8.4Expression in Higher-Eukaryotic Cells
For the production of mammalian proteins, mammalian cells have an obvious advantage. The major problem with expressing genes in mammalian cells is that expression levels like those we have discussed above are simply not currently available. For many years protein production in mammalian cells has utilized strong constitutive promoters to elicit transcription of target genes. Promoters, such as those derived from the SV40 early promoter, the Rous sarcoma virus (RSV) long terminal repeat promoter and the cytomegalovirus (CMV) immediate early promoter, will all constitutively drive the expression of genes placed under their control (Mulligan and Berg, 1981; Gorman et al., 1982; Boshart et al., 1985). Inducible systems can also be used. For example, heat-shock promoters or glucocorticoid hormone inducible systems have been used to express target genes (Wurm, Gwinn and Kingston, 1986; Hirt et al., 1992). These systems, however suffer from leaky gene expression in the absence of induction and potentially damaging induction conditions. To overcome some of the problems of using endogenous promoters to drive target gene expression, systems have been imported from bacteria to control gene expression in mammalian cells.
8.4.1Tet-on/Tet-off System
As we have discussed in Chapter 1, the control of transcriptional initiation is fundamentally different between eukaryotes and prokaryotes. An activator from prokaryotes is unable to bring about a transcriptional response in eukaryotes and vice versa. DNA binding is, however, species independent. The tightly regulated DNA binding properties of prokaryotic activators can be used to direct eukaryotic activation domains to drive the expression of target genes. One such system exploits the DNA properties of the E. coli tetracycline repressor.
8.4 EXPRESSION IN HIGHER-EUKARYOTIC CELLS |
273 |
|
|
The E. coli tet operon was originally identified as a transposon (Tn10) that confers resistance to the antibiotic tetracycline (Foster et al., 1981). The TetR protein, in a similar fashion to the lac repressor protein (LacI) we have already discussed (Chapter 1), binds to the operator of the tetracycline-resistance operon and prevents RNA polymerase from initiating transcription. Activation of the tetracycline-resistance operon occurs when tetracycline itself binds to the repressor and induces a conformational change that inhibits its DNA binding activity. The TetR protein has a very high affinity for the antibiotic (association constant 3 × 10−9 M−1) and will dissociate from its DNA binding site when tetracycline is present at low concentrations (Takahashi, Degenkolb and Hillen, 1991). The regulated DNA binding activity of TetR cannot itself elicit a transcriptional response in eukaryotes, but can if the protein is fused to a eukaryotic transcriptional activator domain. The use of the tet system to drive target gene expression in eukaryotes relies on the insertion of two recombinant DNA molecules into the host cell (Figure 8.7).
•Regulator plasmid – produces a version of the E. coli tetracycline repressor (TetR) that is fused to the transcriptional activation domain of the herpes simplex virus VP16 protein. The fusion protein is constitutively produced in the host cell from the CMV promoter.
•Response plasmid – contains the target gene cloned downstream of multimerised copies of the tetracycline operator (tetO) DNA sequence that form a tetracycline response element (TRE) cloned into a minimal CMV promoter that is not, on its own, able to support gene activation.
In the absence of tetracycline, the TetR-VP16 fusion protein will bind to the TRE and activate transcription of the target gene. Upon the addition of tetracycline to the cells, however, TetR will dissociate and target gene transcription will be turned off (Gossen and Bujard, 1992). That is, the addition of tetracycline turns target gene expression off. The use of the tet system has become more prevalent due to the existence of a mutant version of TetR. The mutant tetracycline repressor contains four amino acid changes (E71K, D95N, L101S and G102D) from the wild-type protein that radically alter its DNA binding properties. Rather than tetracycline inhibiting its DNA binding properties, the mutant protein, called rTetR for reverse tetracycline repressor, will only bind DNA in the presence of tetracycline (Gossen et al., 1995). This means that, with the appropriate TetR fusion to the activation domain of VP16, target gene expression can either be inhibited or activated in the presence of tetracycline.
•Tet-off uses the wild-type TetR protein fused to VP16. Target gene expression is active in the absence of tetracycline but not in its presence.

274 |
PROTEIN PRODUCTION AND PURIFICATION |
8 |
|||||
|
|
|
|
|
|
|
|
|
Tet-Off |
|
|
|
|
||
|
|
PCMV |
|
|
|
|
|
|
|
|
tetR |
|
VP16 |
||
|
|
Regulator |
|
|
|
|
|
|
|
|
|
|
|
||
|
|
|
|
|
|
|
|
VP16
TetR
− Tc
+ Tc c
VP16
TetR |
|
Transcription |
|
|
|
TRE |
PCMV |
Target gene |
Responder
Tet-On
PCMV |
rtetR |
VP16 |
Regulator
VP16
rTetR
+ Tc c
− Tc
VP16
rTetR |
|
Transcription |
|
|
|
TRE |
PCMV |
Target gene |
Responder
Figure 8.7. Tetracycline regulated gene expression for protein production in mammalian cells. The Tet-off and Tet-on systems differ in their transcriptional response to added tetracycline. The Tet-off system turns transcription of the target gene off in response to tetracycline, whereas the Tet-on system, which contains a mutant version of TetR with altered DNA binding properties, activates gene expression in response to tetracycline addition
•Tet-on uses the mutant rTetR proteins fused to VP16. Target gene expression is active in the presence of tetracycline but not in its absence.
The advantage of this on and off switching system is that host cells do not need to be exposed for long times to the antibiotic prior to the induction of either gene expression or gene silencing. Additionally, the control over target gene activation achieved using the Tet system is very tight. For example, transgenic mice have been produced that carry the diptheria toxin A gene under the control for a TRE promoter. Small quantities of the toxin, perhaps as little as a single molecule, will lead to cell death. When fed with water containing
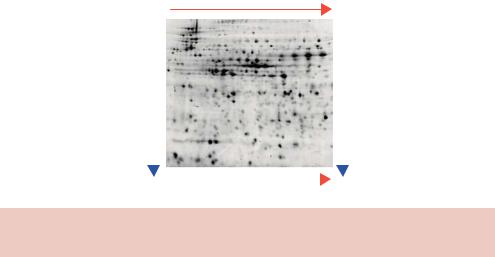
276 |
PROTEIN PRODUCTION AND PURIFICATION 8 |
|
|
reactions will occur in crude cell lysates without the need for protein purification, while other methods, particularly those of structural biology, demand a high degree of protein homogeneity. What is required is the ability to impart the target protein with a unique property that can be used to separate it from all other host proteins. Protein purification tags are protein sequences that possess high-affinity binding properties for particular molecules, and the tag allows the target protein to bind to a solid support, usually in the form of a column matrix, to which very few (if any) other proteins are able to bind. The purification of tagged proteins from host cells consists of four steps: lysis of the host cell, binding of the tagged protein to an affinity column, washing the column to remove untagged proteins, and finally elution of the tagged protein itself. Ideally, the tag should allow binding of the recombinant protein to the column with high affinity and specificity, yet the interaction between the tag and the column needs to be capable of disruption under mild conditions so that the protein is not denatured during the elution process. Additionally, the tag should not interfere with the normal function of the recombinant protein. Some of the commonly used protein purification tags are described below, while others are listed in Table 8.1.
8.5.1The His-tag
The simplest of all protein purification tags, the his-tag is normally composed of six histidine residues. The DNA encoding these residues is cloned into the target gene such that the produced protein contains, at some point in its polypeptide sequence, six consecutive histidine residues (Hoffman and Roeder, 1991). Cloning is often performed such that the tag is located at either the extreme aminoor extreme carboxy-terminal end of the protein, where it is less likely to impair protein function. The tag may, however, also be placed in the middle of a protein if a central region is already known to be nonessential for function (see, e.g. Zenke et al., 1996). The histidines will bind non-covalently and with high affinity for certain metal ions (Figure 8.9). In a technique called immobilized metal ion affinity chromatography (IMAC), metal ions, e.g. nickel, are bound to a resin matrix and used to capture his-tagged proteins (Yip and Hutchens, 1996). The most commonly used resins for this purpose have nitriloacetic acid (NTA) covalently attached to them. NTA has
four coordination sites that bind a single nickel ion very tightly. The charging of NTA with Ni2+ leaves two of the six possible coordination sites of the ion free. In solution these will weakly interact with water, but can interact more strongly with the side chain imidazole rings of consecutive histidine residues on a polypeptide chain. At least six histidine residues are required to provide the necessary binding affinity to firmly adhere the tagged protein to the column. The vast majority of host proteins will not be able to bind to a column of this type.