
John Wiley & Sons - 2004 - Analysis of Genes and Genomes
.pdf68 |
BASIC TECHNIQUES IN GENE ANALYSIS 2 |
|
|
as the recognition site for HindII. Wherever this particular sequence of six base pairs occurs unmodified in a DNA molecule, HindII will cleave the DNA.
In fact, Smith was very fortunate in his experiments. Haemophilus influenzae contain two different restriction enzymes, HindII and HindIII, which were probably both present in his partially purified restriction enzyme activity. HindIII recognizes and cleaves the sequence 5 -AAGCTT-3 , but this sequence does not occur within the bacteriophage T7 genome, and thus would not interfere with the digestion patterns he obtained.
Shortly after Smith’s discovery, another restriction enzyme, EcoRI, was isolated and characterized from Escherichia coli strain RY13 (Hedgpeth, Goodman and Boyer, 1972). This enzyme was found to have the recognition site 5 -GAATTC-3 . More than 900 restriction enzymes have now been isolated from over 230 species of bacteria. Restriction enzymes have names that reflect their origin – the first letter of the name comes from the genus and the second and third letters from the species of bacteria from which they were isolated. The letters were, by convention, written in italics, but recently this has changed so that they are now written in plain font (Roberts et al., 2003). The numbers following the nuclease name indicate the order in which the enzyme was isolated from the bacterial strain. The number is written as a Roman numeral. For example, the first restriction enzyme to be isolated from the bacterium Providencia stuartii was named PstI, and the second to be isolated from Bacillus stearothermophilus strain ET was named BstEII.
A pertinent question to ask here is why the DNA of an organism producing a restriction enzyme is not itself attacked by the restriction enzyme. In Smith’s experiments described above, he was able to observe cleavage of T7 genomic DNA by HindII, but genomic DNA from Haemophilus influenzae, from which the enzyme is derived, was not cleaved, even though it contains many recognition sites for HindII. The answer lies in the modification part of the restriction –modification system. Haemophilus influenzae, like all organisms that produce restriction enzymes, also produces enzymes that modify its own DNA so that it will not be cleaved by the restriction enzyme. For example, E. coli RY13 strains, which produce EcoRI, also make EcoRI methylase. Like many restriction enzymes, the genes encoding both the nuclease and the modifying enzymes are adjacent to each other in the genome. EcoRI methylase attaches methyl groups to the N6 position (Figure 1.5) of the second adenine residues in the EcoRI recognition site, and this covalent modification renders the site uncleavable by EcoRI (Figure 2.1). Indeed, a methylation of just one of the DNA strands in the EcoRI recognition sequence is sufficient to prevent cleavage by the restriction enzyme. The resistance of the hemimethylated DNA site to cleavage protects the bacteria DNA from degradation immediately after
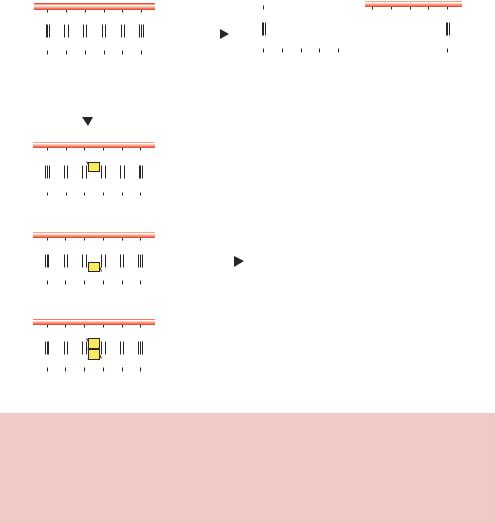
|
|
|
|
|
|
|
|
|
|
|
|
|
|
|
|
2.1 |
RESTRICTION ENZYMES |
69 |
||||||||
|
|
|
|
|
|
|
|
|
|
|
|
|
|
|
|
|
|
|
|
|
|
|
|
|
|
|
5′ |
|
|
|
|
|
|
3′ |
|
|
|
|
5′ |
|
|
|
3′ |
|
|
5′ |
|
|
|
3′ |
|||
|
|
|
|
|
|
|
|
|
|
|
|
|
|
|
|
|||||||||||
|
|
|
|
|
|
|
|
|
|
|
|
|
A A T |
T |
|
|||||||||||
|
|
|
G |
A |
A |
T |
T |
C |
EcoRI |
|
G |
+ |
C |
|||||||||||||
|
|
|
|
|
|
|
|
|
|
|
||||||||||||||||
|
|
|
|
|
|
|
|
|
|
|
|
|
|
|
|
|
|
|
||||||||
|
|
|
G T T A A G |
|
|
|
|
3′ |
|
C T T A A |
|
G |
||||||||||||||
3′ |
|
|
|
|
|
|
5′ |
|
|
|
|
|
|
|
|
|
|
5′ |
3′ |
|
5′ |
|||||
|
|
|
|
|
|
|
|
|
|
|
|
|
|
|
|
|
||||||||||
|
|
|
|
|
|
|
|
|
|
|
|
|
|
|
|
|
||||||||||
|
|
|
|
|
|
EcoRI methylase |
|
|
|
|
|
|
|
|
|
|
|
|
|
|
|
|
||||
|
|
|
|
|
|
|
|
|
|
|
|
|
|
|
|
|
|
|
|
|
|
|||||
5′ |
|
|
|
|
|
|
|
3′ |
|
|
|
|
|
|
|
|
|
|
|
|
|
|
|
|
|
|
|
|
|
|
|
|
|
|
|
|
|
|
|
|
|
|
|
|
|
|
|
|
|
|
|||
|
|
|
G |
A |
A |
T |
T |
C |
|
|
|
|
|
|
|
|
|
|
|
|
|
|
|
|
||
|
|
|
|
|
|
m |
|
|
|
|
|
|
|
|
|
|
|
|
|
|
|
|
|
|
|
|
|
|
|
C |
T |
T |
A |
A |
G |
|
|
|
|
|
|
|
|
|
|
|
|
|
|
|
|
||
3′ |
|
|
|
|
|
|
5′ |
|
|
|
|
|
|
|
|
|
|
|
|
|
|
|
|
|||
|
|
|
|
|
|
|
|
|
|
|
|
|
|
|
|
|
|
|
|
|
|
|
||||
|
|
Hemimethylated |
|
|
|
|
|
|
|
|
|
|
|
|
|
|
|
|
|
|||||||
|
|
|
|
|
|
|
|
|
|
|
|
|
|
|
|
|
|
|
|
|
|
|
||||
5′ |
|
|
|
|
|
|
|
3′ |
|
|
|
|
|
|
|
|
|
|
|
|
|
|
|
|
||
|
|
|
|
|
|
|
|
|
|
|
|
|
|
|
|
|
|
|
|
|
|
|
||||
|
|
|
|
|
|
|
|
|
|
|
|
|
|
|
|
|
|
|
|
|
|
|
|
|||
|
|
|
G |
A |
A |
T |
T |
C |
|
|
EcoRI |
|
|
|
|
|
|
|
|
|
|
|
||||
|
|
|
|
|
|
|
|
|
|
|
|
|
No cleavage |
|
|
|
|
|
|
|
||||||
|
|
|
C |
T |
T |
m |
A |
G |
|
|
|
|
|
|
|
|
|
|
|
|
||||||
|
|
|
A |
|
|
|
|
|
|
|
|
|
|
|
|
|
|
|
|
|||||||
3′ |
|
|
|
|
|
|
|
5′ |
|
|
|
|
|
|
|
|
|
|
|
|
|
|
|
|
||
|
|
|
|
|
|
|
|
|
|
|
|
|
|
|
|
|
|
|
|
|
|
|
|
|||
|
|
Hemimethylated |
|
|
|
|
|
|
|
|
|
|
|
|
|
|
|
|
|
|||||||
|
|
|
|
|
|
|
|
|
|
|
|
|
|
|
|
|
|
|
|
|
|
|||||
5′ |
|
|
|
|
|
|
3′ |
|
|
|
|
|
|
|
|
|
|
|
|
|
|
|
|
|||
|
|
|
|
|
|
|
|
|
|
|
|
|
|
|
|
|
|
|
|
|
|
|
||||
|
|
|
A |
A |
|
|
|
|
|
|
|
|
|
|
|
|
|
|
|
|
|
|
|
|||
|
|
|
G |
T |
T |
C |
|
|
|
|
|
|
|
|
|
|
|
|
|
|
|
|
||||
|
|
|
|
|
|
m |
|
|
|
|
|
|
|
|
|
|
|
|
|
|
|
|
|
|
|
|
|
|
|
C |
T |
T |
m |
A |
G |
|
|
|
|
|
|
|
|
|
|
|
|
|
|
|
|
||
|
|
|
A |
|
|
|
|
|
|
|
|
|
|
|
|
|
|
|
|
|||||||
3′ |
|
|
|
|
|
|
|
5′ |
|
|
|
|
|
|
|
|
|
|
|
|
|
|
|
|
|
|
|
|
|
|
|
|
|
|
|
|
|
|
|
|
|
|
|
|
|
|
|
|
|
|
Fully methylated
Figure 2.1. Protection of DNA cleavage by modification. The restriction enzyme EcoRI recognizes and cleaves the sequence 5 -GAATTC-3 . E. coli R strains, from which this enzyme is derived, protect their own DNA from fragmentation by also producing a specific methylase. EcoRI methylase takes a methyl group from S-adenosylmethionine and places it on the N6 of the second adenine in the recognition sequence. EcoRI restriction enzyme is unable to cleave the methylated DNA
semiconservative DNA replication of the fully methylated sequence, until EcoRI methylase can once again restore the fully methylated state. Methylation of the adenine bases in the EcoRI recognition site does not affect the overall DNA structure, but inhibits cleavage by the enzyme (Jen-Jacobson et al., 1996). DNA methylase enzymes (sometimes called DNA methyltransferases) operate using an ingenious mechanism to add methyl groups to specific bases within doublestranded DNA. A number of these enzymes have been studied structurally (Figure 2.2). Binding of the methylase to DNA results in the flipping out of the base that is to be methylated, and stabilization of the orphaned base left in the helix by other parts of the protein. Base flipping involves rotation of backbone bonds in double-stranded DNA to expose an out-of-stack nucleotide, which
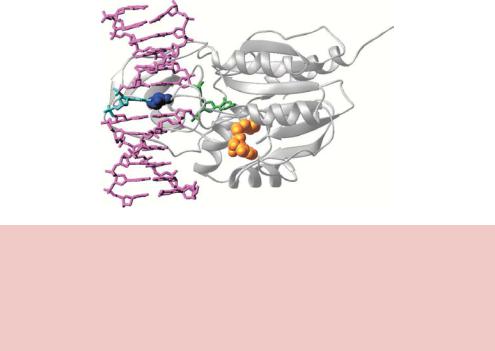
70 |
BASIC TECHNIQUES IN GENE ANALYSIS 2 |
|
|
Figure 2.2. The structure of the HhaI methylase bound to DNA. The enzyme (shown in white) inserts an amino acid (dark blue) into the double helix of its recognition site (5 - GCGC-3 ) and flips the first C residue (green) completely out of the helical axis (Blumenthal and Cheng, 2001). This residue is methylated using S-adenosylmethionine as a donor (shown in gold). The methylated base presumably flips back into the helix when the enzyme dissociates. Reproduced from Blumenthal and Cheng (2001) by permission of Xiaodong Cheng (Emory University)
can then be a substrate for the enzyme-catalysed chemical reaction (Cheng and Roberts, 2001).
So, the organism is able to protect its own DNA while degrading foreign DNA that it not correctly methylated. As we will see later, methylase enzymes are important tools for the genetic engineer who wants to protect certain DNA sequences from cleavage by restriction enzymes in vitro.
2.1.1Types of Restriction–Modification System
Restriction –modification systems are divided into three types on the basis of enzyme complexity, cofactor requirements and the position of DNA cleavage, although new systems are being discovered that do not fit readily into this classification. The properties of each type are summarized in Table 2.1.
The restriction enzyme EcoK, the first to be isolated in 1968, is a type I endonuclease that recognizes the DNA sequence 5 -AACN6GTGC-3 , where N can be any nucleotide, but cleaves DNA in an apparently random fashion
well away from the recognition site. Type I restriction enzymes require ATP, S-adenosylmethionine (SAM) and magnesium ions (Mg2+) for activity. The
2.1 RESTRICTION ENZYMES |
71 |
|
|
enzymes are composed of three subunits, a specificity subunit that determines the DNA recognition site, a modification subunit and a restriction subunit. In the presence of SAM, the enzyme binds to its recognition site irrespective of its methylation state. If the recognition site is methylated, then ATP hydrolysis stimulates the dissociation of the enzyme from DNA. If the recognition site is methylated on one strand only (hemimethylated), then ATP stimulates the methylation of the other strand, with SAM being a methyl donor. If the site is unmethylated, then DNA cleavage occurs. Cleavage occurs several kilobases away from the recognition site, with the intervening DNA sequences looping out from the enzyme. The relatively random nature of the DNA cleavage event makes enzymes of this type not particularly useful for genetic engineering experiments.
The HindII, HindIII and EcoRI restriction enzymes are all examples of type II enzymes. They recognize DNA sequences that are rotationally symmetrical – the sequence in the 5 to 3 direction on one DNA strand is the same as the sequence in the 5 to 3 direction on the other strand. Such DNA sequences are often referred to as being palindromic. The palindromic binding site may either be continuous (e.g. KpnI recognizes the sequence 5 -GGTACC-3 ) or interrupted (e.g. BstEII recognizes the sequence 5 -GGTNACC-3 , where N can be any nucleotide). Type II restriction enzymes require only magnesium ions for activity and usually cleave DNA either within the recognition site or very close to it. Different type II restriction enzymes will recognize and cleave different sites, but one restriction enzyme will always cut a particular base sequence the same way, no matter what the origin of the DNA. Different restriction enzymes isolated from different sources often recognize the same DNA sequence, although they may cleave the DNA differently. Such enzymes are called isoschizomers of each other. For example, the restriction enzymes SmaI and XmaI both recognize the same DNA sequence (5 -CCCGGG-3 ) and are therefore isoschizomers, but SmaI cleaves in the middle of the site between the central C and G nucleotides, while XmaI cleaves after the first C nucleotide on each DNA strand.
The type III restriction systems act as complexes of two different subunits: one subunit (M) is responsible for DNA sequence recognition and modification, and the other subunit (R) is responsible for nuclease action. DNA cleavage requires magnesium ions, ATP, and is stimulated by SAM. The recognition sites are approximately symmetric, and cleavage occurs by nicking one DNA strand at a measured distance to one side of the recognition sequence. The two recognition sites in opposite orientation are necessary to break the DNA duplex.
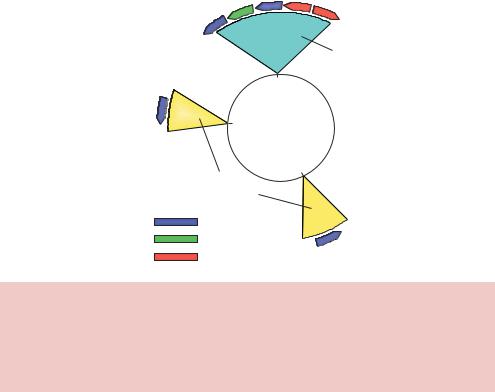
72 |
BASIC TECHNIQUES IN GENE ANALYSIS 2 |
|
|
2.1.2Other Modification Systems
Most E. coli strains used in the laboratory for DNA isolation contain three site-specific DNA methylation systems (Figure 2.3). DNA modification by these systems can lead to the protection of potential restriction enzyme recognition sites from cleavage. Consequently, knowledge of the sensitivity of restriction endonucleases to DNA methylation conferred by these systems in E. coli is of great importance to the genetic engineer.
(a) Dam methylation. The methylase specified by the Dam gene methylates the N6-position (see Figure 1.5) of the adenine residue in the sequence 5 -GATC-3 . The methylation of adenine at this point does not affect its ability to base pair with thymine. Methylation by Dam has been implicated in a variety of cellular functions, including DNA replication. Dam methylase is a relatively slow enzyme, and as a consequence newly synthesized DNA will be hemimethylated – the old DNA strand will be methylated, while the newly synthesized strand will not (Campbell and
M.EcoKI |
EcoKMrr |
Mcr B,C |
|
|
|
Type I |
|
|
|
4574 |
|
|
|
Dam |
E. coli K |
|
|
|
|
3512 |
|
|
|
|
4.64 Mbases |
|
|
|
|
4289 ORFs |
|
|
|
|
2029 |
|
|
|
|
Type II |
|
|
|
|
Methylase |
|
|
|
|
Specificity |
|
|
|
|
Restriction |
Dcm |
|
|
|
|
|
|
|
Figure 2.3. Restriction – modification systems found in laboratory |
E. coli |
K strains. |
||
The genomic location of |
restriction – modification systems in E. coli |
K12. |
The circle |
represents the genome of E. coli, with the numbers on the inside indicating genomic location. E. coli contains two type II modification systems (Dam and Dcm) and two type I restriction – modification systems (Mcr and EcoKI). The various methylase, restriction and specificity subunits are colour coded as indicated
2.1 RESTRICTION ENZYMES |
73 |
|
|
Kleckner, 1988). Later, however, both strands will become methylated. This is important since the methylation state of the E. coli origin of replication (oriC) controls whether replication will proceed or not. If the DNA on both strands of oriC is methylated, then this site can serve as an origin of replication and DNA synthesis will proceed. If, however, the DNA at oriC is hemimethylated, oriC is not active and DNA replication will not start. The slow remethylation of oriC after one round of replication delays another round until the first is complete (von Freiesleben et al., 2000).
(b)Dcm methylation. The methylase specified by the Dcm gene methylates the C5-position of the internal cytosine residue in the sequence 5 -CCWGG- 3 – where W is either an A or T residue. The precise role of Dcm methylation in the cell remains obscure since E. coli deleted for the dcm gene have no obvious phenotype (Palmer and Marinus, 1994).
(c)Mcr system. In the 1980s it became apparent that DNA from various bacterial and eukaryotic sources could only be cloned at very low efficiency in certain E. coli strains, the problem being that the incoming DNA was being restricted by the host. The phenomenon is caused by methylcytosine in DNA, and is called modified cytosine restriction (Mcr). One Mcr system is encoded by two genes, McrB and McrC, from within the E. coli genome, close to the site of the EcoKI restriction –modification system (Figure 2.3). The proteins encoded by these genes recognize the
DNA sequence 5 -R –mC – N40 – 80 – R – mC-3 , where R is either A or G, N is any nucleotide and mC is methylated cytosine. Cleavage occurs at multiple sites in both strands between the methylated cytosine residues, and requires GTP to translocate DNA between the two 5 -RmC-3 binding sites (Panne, Raleigh and Bickle, 1999; Stewart et al., 2000; Panne et al., 2001).
The Dam- and Dcm-dependent methylation may interfere with cleavage by restriction endonucleases with recognition sites partially or completely overlapping such methylation sites. For example, MboI (recognition sequence 5 -GATC-3 ) does not cut DNA methylated by the Dam methylase, while its isoschizomer Bsp143I is insensitive to Dam methylation. Similarly, EcoRII (5 CCWGG-3 - where w = A or T) does not cleave Dcm methylated DNA, meanwhile its isoschizomer MvaI does.
The precise nature of cutting by the type II restriction enzymes makes them most applicable for use in genetic engineering. The other systems are important, however, since they must be removed from host cells into which foreign DNA is to be inserted if the DNA is to be cut at certain sites.
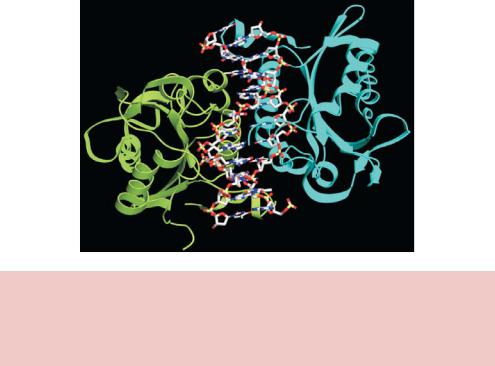
74 |
BASIC TECHNIQUES IN GENE ANALYSIS 2 |
|
|
2.1.3How Do Type II Restriction Enzymes Work?
Despite little, if any, sequence homology at the amino acid level, all of the type II restriction enzymes that have been studied at the structural level have a highly conversed catalytic core composed of a five-stranded β-sheet flanked by two α-helices (Figure 2.4) (Kovall and Matthews, 1999). Restriction endonucleases appear to function by ‘scanning’ the length of a DNA molecule by binding to it in a non-specific fashion (Pingoud and Jeltsch, 2001). Once it encounters its particular recognition sequence, the restriction enzyme undergoes a large conformational change, which activates the catalytic sites. The enzyme will
then make one cut in each of the two sugar –phosphate backbones of the DNA double helix to generate a 3 hydroxyl and a 5 phosphate (Figure 2.4). The positions of these two cuts, both in relation to each other, and to the recognition site itself, are determined by the individual restriction enzyme. Once the cuts have been made, the DNA molecule will break into fragments. Not all restriction enzymes cut symmetrically and leave blunt ends like HindII described above. Many cleave the DNA backbones in positions that are not
directly opposite each other and consequently leave overhanging ends at either the 5 - or the 3 -end of a DNA molecule (see Figure 2.5).
Figure 2.4. The structure of the restriction enzyme BamHI bound to DNA. The enzyme recognizes double-stranded DNA of the sequence 5 -GGATCC-3 , and cleaves the phosphodiester backbone between the two G residues. This results in formation of two DNA fragments that have 5 overhanging ends – called cohesive or sticky ends. The protein is a dimer of identical subunits (coloured in green and cyan)
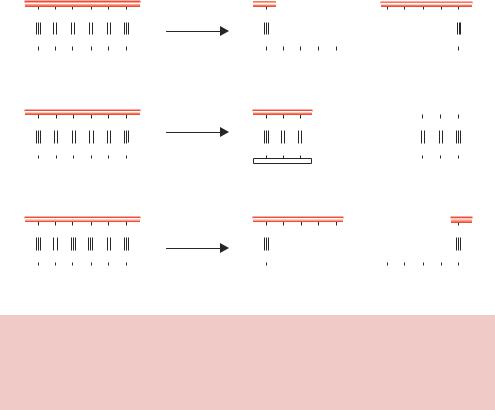
|
|
|
|
|
|
|
|
|
|
|
2.1 |
RESTRICTION ENZYMES |
75 |
|||||||
|
|
|
|
|
|
|
|
|
|
|
|
|
|
|
|
|
|
|
|
|
5′ |
|
|
|
|
3′ |
5′ |
|
|
|
3′ |
|
5′ |
|
|
|
|
|
3′ |
||
|
|
|
|
|
|
|
|
|
|
|
|
|
||||||||
|
G |
A A T T C |
EcoRI |
|
|
|
|
G |
|
|
+ |
A A T T C |
||||||||
|
|
|
|
|
|
|
|
|
|
|
|
|
|
|
|
|
|
|||
|
C T T A A |
G |
|
|
|
|
|
|
|
|
|
|
|
G |
||||||
3′ |
5′ |
|
|
|
|
C T T A A |
|
|
|
|||||||||||
|
|
|
|
3′ |
|
|
|
|
|
|
5′ |
|
3′ |
|
|
5′ |
||||
|
|
|
|
|
|
|
|
|
|
|
|
|||||||||
|
|
|
|
|
|
|
|
|
|
|
|
|||||||||
|
|
|
|
|
|
|
|
|
|
|
|
|
5′ overhang ends |
|
|
|
||||
|
|
|
|
|
|
|
|
|
|
|
|
|
|
|
|
|||||
5′ |
|
|
|
|
3′ |
5′ |
|
|
|
|
|
|
3′ |
5′ |
|
|
|
|
3′ |
|
|
|
|
|
|
|
|
|
|
|
|
|
|
||||||||
|
|
|
|
|
|
|
|
|
|
|
|
|
|
|||||||
|
G A T |
A T C |
EcoRV |
|
|
|
|
G |
A T |
|
|
|
A T |
C |
||||||
|
C T A |
T A G |
|
|
|
|
|
|
|
|
+ |
|
T A G |
|||||||
|
|
3′ |
C T A |
5′ |
|
|||||||||||||||
3′ |
|
|
|
|
5′ |
|
|
|
3′ |
|
|
|
|
5′ |
||||||
|
|
|
|
|
|
|
|
|
|
|
||||||||||
|
|
|
|
|
|
|
|
|
|
|
||||||||||
|
|
|
|
|
|
|
|
|
|
|
|
|
Blunt ends |
|
|
|
||||
5′ |
|
|
|
|
3′ |
5′ |
|
|
|
|
|
3′ |
|
5′ |
|
|
|
3′ |
||
|
|
|
|
|
|
|
|
|
|
|
|
|
||||||||
|
|
|
|
|
|
|
|
|
|
|
||||||||||
C T G C A |
G |
|
|
|
|
|
|
|
|
|
||||||||||
|
PstI |
|
|
|
|
C T G C A |
|
|
|
G |
||||||||||
|
|
|
|
|
|
|
|
|
G |
|
|
+ |
A C G T C |
|||||||
|
G |
A C G T C |
|
|
|
|
|
|
|
|||||||||||
3′ |
5′ |
3′ |
|
5′ |
|
3′ |
||||||||||||||
|
|
|
|
|
|
|
|
|
|
|
|
5′ |
||||||||
|
|
|
|
|
|
|
|
|
|
|
|
|||||||||
|
|
|
|
|
|
|
|
|
|
|
|
|||||||||
|
|
|
|
|
|
|
|
|
|
|
|
|
3′ overhang ends |
|
|
|
||||
|
|
|
|
|
|
|
|
|
|
|
|
|
|
|
|
Figure 2.5. DNA cleavage by type II restriction enzymes. Different restriction enzymes recognize different DNA sequences and can cleave to give either overhanging ends or blunt ends. The recognition site of each enzyme is shown, together with the cleavage site, indicated by the blue line. After cleavage, the weak hydrogen bonds holding the overhanging ends will fall apart, and two new DNA ends will be formed
Take, for example, the restriction enzyme EcoRI. The recognition sequence for this enzyme is 5 -GAATTC-3 . When the enzyme encounters this sequence, it cleaves each backbone between the G and the closest A base residues (Mertz and Davis, 1972). Once the cuts have been made, the resulting fragments are held together only by the relatively weak hydrogen bonds that hold the four complementary bases to each other. The weakness of these bonds allows the DNA fragments to separate from one each other. Each resulting fragment has a protruding 5 -end composed of unpaired bases. Other enzymes, for example PstI, create cuts in the DNA backbone that result in protruding 3 -ends. The protruding ends – both 3 and 5 – are sometimes called ‘sticky’ or ‘cohesive’ ends because they will bond with complementary sequences of bases. In other words, if an unpaired length of bases (5 -AATT-3 ) encounters another unpaired length with the sequence (3 -TTAA-5 ), they will bond to each other – they are ‘sticky’ for each other. Although compatible sticky-ended DNA fragments can associate with each other through complementary base pairing, they will not form a continuous sugar –phosphate DNA backbone. The nicks in the DNA
76 |
BASIC TECHNIQUES IN GENE ANALYSIS 2 |
|
|
backbone of paired sticky ends can, however, be repaired using DNA ligase enzymes (see below). The cellular origin, or even the species origin, of the sticky ends does not affect their stickiness. Any pair of complementary sequences will tend to bond, even if one of the sequences comes from a length of human DNA, and the other comes from a length of bacterial DNA.
How often do restriction enzyme recognition sites occur within a particular DNA sequence? Most of the restriction enzymes that we have talked about so far recognize DNA sequences that are six base pairs in length. Some other restriction enzymes, however, recognize either longer (seven or eight base pairs) or shorter (four or five base pairs) DNA sequences. The length of the recognition site plays a role in determining the frequency of DNA cleavage of a particular DNA molecule. We would expect that any particular restriction enzyme that recognizes a 4 bp target sequence would cut DNA once every 44 (i.e. 256) bp in a random DNA sequence, assuming all bases occur equally frequently. Any particular 6 bp target would be expected to occur once every 46 (4096) bp, and an 8 bp target every 48 (65 536) bp. So, the treatment of genomic DNA with, say EcoRI, should result in the formation of a series of DNA fragments of approximate size 4 kb. The actual frequency and DNA cleavage by any particular restriction enzyme is obviously dependent upon the frequency at which the bases that make up its recognition site actually occur within that DNA. As we saw in Chapter 1, Chargaff noted that the amount of C + G residues in different organisms was different to the amount of A + T. Such differences – often expressed as the GC content of a genome – represent the percentage of nucleotides within the genome that are either G or C. Restriction enzyme cleavage sites that contain a high proportion of G and C residues will cut DNA relatively infrequently if the overall GC content of the genome is low. Additionally, higher-eukaryotic genomes tend to contain fewer 5 -CG-3 dinucleotide pairs (also called CpG) than would be predicted by chance (Bird, 1980). Consequently, restriction enzymes that cleave recognition sequences bearing this motif will cut DNA from higher eukaryotes less frequently than might be predicted.
2.2Joining DNA Molecules
The discovery of restriction enzymes meant that the cleavage of DNA molecules at specific places along their length now became possible. The next problem that faces a genetic engineer is to join two DNA molecules together. The solution to the problem of DNA joining came from work carried out in the late 1960s. Several laboratories simultaneously discovered DNA ligase, an enzyme that catalyses the formation of a phosphodiester bond between two DNA
2.3 THE BASICS OF CLONING |
77 |
|
|
chains (Weiss and Richardson, 1967; Zimmerman et al., 1967). DNA ligase enzymes require a free hydroxyl group at the 3 -end of one DNA chain and a phosphate group at the 5 -end of the other. The formation of a phosphodiester bond between these groups requires energy. In E. coli and other bacteria NAD+ serves this role, whereas in animal cells and bacteriophage ATP drives the reaction. DNA ligases are only able to join DNA molecules that are part of a double helix – they are unable to join two molecules of single-stranded DNA. As we saw in Chapter 1, the role of DNA ligases is to seal nicks in the backbone of double-stranded DNA after replication. This joining process is essential for the normal synthesis of DNA and for repairing damaged DNA, and has been exploited by genetic engineers to join DNA chains to form recombinant DNA molecules.
The mechanism of action of DNA ligases has been studied in detail (Timson, Singleton and Wigley, 2000). ATP, or NAD+, reacts with the ligase enzyme to form a covalent enzyme –AMP complex in which the AMP is linked to an ε-amino group of a lysine residue in the active site of the enzyme in the form of a phosphoamide bond (Figure 2.6). The AMP moiety activates the phosphate group at the 5 -end of the DNA that is to be joined. The final step is a nucleophilic attack by the 3 -hydroxyl group on this activated phosphorus atom. A phosphodiester bond is formed and AMP is released. This sequence of reactions is driven by the hydrolysis of the pyrophosphate that was released in the formation of the enzyme –adenylate complex. Thus, two high-energy phosphate bonds are spent in forming a phosphodiester bond in the DNA backbone if ATP is the energy source.
The temperature optimum for the ligation of nicked DNA is 37 ◦C, but at this temperature the hydrogen bonding between the sticky ends of restriction enzyme cleaved DNA is unstable. For example, EcoRI generated DNA ends associate through only four AT base pairs, and these are not sufficient to resist thermal disruption at such a high temperature. The optimum temperature for ligating the sticky ends of restriction enzyme generated DNA fragments is therefore a compromise between the rate of enzyme action and the association of the DNA fragments. The temperature optimum for such reactions has been found to be in the range of 4 –20 ◦C (Sgaramella and Ehrlich, 1978). The DNA ligase from E. coli will only ligate blunt-ended DNA fragments if they are at a very high concentration. The high concentration is thought to promote association of the DNA fragments so that the ligation reaction may occur. The ligase enzyme from bacteriophage T4 will ligate blunt-ended DNA fragments, although at low efficiency with respect to the efficiency of ligation of stickyended DNA fragments (Ferretti and Sgaramella, 1981). A general scheme for the cutting and rejoining of DNA molecules is shown in Figure 2.7.