
- •Contents
- •Contributors
- •Part I General Principles of Cell Death
- •1 Human Caspases – Apoptosis and Inflammation Signaling Proteases
- •1.1. Apoptosis and limited proteolysis
- •1.2. Caspase evolution
- •2. ACTIVATION MECHANISMS
- •2.2. The activation platforms
- •2.4. Proteolytic maturation
- •3. CASPASE SUBSTRATES
- •4. REGULATION BY NATURAL INHIBITORS
- •REFERENCES
- •2 Inhibitor of Apoptosis Proteins
- •2. CELLULAR FUNCTIONS AND PHENOTYPES OF IAP
- •3. IN VIVO FUNCTIONS OF IAP FAMILY PROTEINS
- •4. SUBCELLULAR LOCATIONS OF IAP
- •8. IAP–IAP INTERACTIONS
- •10. ENDOGENOUS ANTAGONISTS OF IAP
- •11. IAPs AND DISEASE
- •SUGGESTED READINGS
- •1. INTRODUCTION
- •2.1. The CD95 (Fas/APO-1) system
- •2.1.1. CD95 and CD95L: discovery of the first direct apoptosis-inducing receptor-ligand system
- •2.1.2. Biochemistry of CD95 apoptosis signaling
- •2.2. The TRAIL (Apo2L) system
- •3.1. The TNF system
- •3.1.1. Biochemistry of TNF signal transduction
- •3.1.2. TNF and TNF blockers in the clinic
- •3.2. The DR3 system
- •4. THE DR6 SYSTEM
- •6. CONCLUDING REMARKS AND OUTLOOK
- •SUGGESTED READINGS
- •4 Mitochondria and Cell Death
- •1. INTRODUCTION
- •2. MITOCHONDRIAL PHYSIOLOGY
- •3. THE MITOCHONDRIAL PATHWAY OF APOPTOSIS
- •9. CONCLUSIONS
- •SUGGESTED READINGS
- •1. INTRODUCTION
- •3. INHIBITING APOPTOSIS
- •4. INHIBITING THE INHIBITORS
- •6. THE BCL-2 FAMILY AND CANCER
- •SUGGESTED READINGS
- •6 Endoplasmic Reticulum Stress Response in Cell Death and Cell Survival
- •1. INTRODUCTION
- •2. THE ESR IN YEAST
- •3. THE ESR IN MAMMALS
- •4. THE ESR AND CELL DEATH
- •5. THE ESR IN DEVELOPMENT AND TISSUE HOMEOSTASIS
- •6. THE ESR IN HUMAN DISEASE
- •7. CONCLUSION
- •7 Autophagy – The Liaison between the Lysosomal System and Cell Death
- •1. INTRODUCTION
- •2. AUTOPHAGY
- •2.2. Physiologic functions of autophagy
- •2.3. Autophagy and human pathology
- •3. AUTOPHAGY AND CELL DEATH
- •3.1. Autophagy as anti–cell death mechanism
- •3.2. Autophagy as a cell death mechanism
- •3.3. Molecular players of the autophagy–cell death cross-talk
- •4. AUTOPHAGY, CELLULAR DEATH, AND CANCER
- •5. CONCLUDING REMARKS AND PENDING QUESTIONS
- •SUGGESTED READINGS
- •8 Cell Death in Response to Genotoxic Stress and DNA Damage
- •1. TYPES OF DNA DAMAGE AND REPAIR SYSTEMS
- •2. DNA DAMAGE RESPONSE
- •2.2. Transducers
- •2.3. Effectors
- •4. CHROMATIN MODIFICATIONS
- •5. CELL CYCLE CHECKPOINT REGULATION
- •6. WHEN REPAIR FAILS: SENESCENCE VERSUS APOPTOSIS
- •6.1. DNA damage response and the induction of apoptosis
- •6.2. p53-independent mechanisms of apoptosis
- •6.3. DNA damage response and senescence induction
- •7. DNA DAMAGE FROM OXIDATIVE STRESS
- •SUGGESTED READINGS
- •9 Ceramide and Lipid Mediators in Apoptosis
- •1. INTRODUCTION
- •3.1. Basic cell signaling often involves small molecules
- •3.2. Sphingolipids are cell-signaling molecules
- •3.2.1. Ceramide induces apoptosis
- •3.2.2. Ceramide accumulates during programmed cell death
- •3.2.3. Inhibition of ceramide production alters cell death signaling
- •4.1. Ceramide is generated through SM hydrolysis
- •4.3. aSMase can be activated independently of extracellular receptors to regulate apoptosis
- •4.4. Controversial aspects of the role of aSMase in apoptosis
- •4.5. De novo ceramide synthesis regulates programmed cell death
- •4.6. p53 and Bcl-2–like proteins are connected to de novo ceramide synthesis
- •4.7. The role and regulation of de novo synthesis in ceramide-mediated cell death is poorly understood
- •5. CONCLUDING REMARKS AND FUTURE DIRECTIONS
- •5.1. Who? (Which enzyme?)
- •5.2. What? (Which ceramide?)
- •5.3. Where? (Which compartment?)
- •5.4. When? (At what steps?)
- •5.5. How? (Through what mechanisms?)
- •5.6. What purpose?
- •6. SUMMARY
- •SUGGESTED READINGS
- •1. General Introduction
- •1.1. Cytotoxic lymphocytes and apoptosis
- •2. CYTOTOXIC GRANULES AND GRANULE EXOCYTOSIS
- •2.1. Synthesis and loading of the cytotoxic granule proteins into the secretory granules
- •2.2. The immunological synapse
- •2.3. Secretion of granule proteins
- •2.4. Uptake of proapoptotic proteins into the target cell
- •2.5. Activation of death pathways by granzymes
- •3. GRANULE-BOUND CYTOTOXIC PROTEINS
- •3.1. Perforin
- •3.2. Granulysin
- •3.3. Granzymes
- •3.3.1. GrB-mediated apoptosis
- •3.3.2. GrA-mediated cell death
- •3.3.3. Orphan granzyme-mediated cell death
- •5. CONCLUSIONS
- •REFERENCES
- •Part II Cell Death in Tissues and Organs
- •1.1. Death by trophic factor deprivation
- •1.2. Key molecules regulating neuronal apoptosis during development
- •1.2.1. Roles of caspases and Apaf-1 in neuronal cell death
- •1.2.2. Role of Bcl-2 family members in neuronal cell death
- •1.3. Signal transduction from neurotrophins and neurotrophin receptors
- •1.3.1. Signals for survival
- •1.3.2. Signals for death
- •2.1. Apoptosis in neurodegenerative diseases
- •2.1.4. Amyotrophic lateral sclerosis
- •2.2. Necrotic cell death in neurodegenerative diseases
- •2.2.1. Calpains
- •2.2.2. Cathepsins
- •3. CONCLUSIONS
- •ACKNOWLEDGMENT
- •SUGGESTED READINGS
- •ACKNOWLEDGMENT
- •SUGGESTED READINGS
- •1. INTRODUCTION
- •5. S-NITROSYLATION OF PARKIN
- •7. POTENTIAL TREATMENT OF EXCESSIVE NMDA-INDUCED Ca2+ INFLUX AND FREE RADICAL GENERATION
- •8. FUTURE THERAPEUTICS: NITROMEMANTINES
- •9. CONCLUSIONS
- •Acknowledgments
- •SUGGESTED READINGS
- •3. MITOCHONDRIAL PERMEABILITY TRANSITION ACTIVATED BY Ca2+ AND OXIDATIVE STRESS
- •4.1. Mitochondrial apoptotic pathways
- •4.2. Bcl-2 family proteins
- •4.3. Caspase-dependent apoptosis
- •4.4. Caspase-independent apoptosis
- •4.5. Calpains in ischemic neural cell death
- •5. SUMMARY
- •ACKNOWLEDGMENTS
- •SUGGESTED READINGS
- •1. INTRODUCTION
- •2. HISTORICAL ANTECEDENTS
- •7.1. Activation of p21 waf1/cip1: Targeting extrinsic and intrinsic pathways to death
- •8. CONCLUSION
- •ACKNOWLEDGMENTS
- •REFERENCES
- •16 Apoptosis and Homeostasis in the Eye
- •1.1. Lens
- •1.2. Retina
- •2. ROLE OF APOPTOSIS IN DISEASES OF THE EYE
- •2.1. Glaucoma
- •2.2. Age-related macular degeneration
- •4. APOPTOSIS AND OCULAR IMMUNE PRIVILEGE
- •5. CONCLUSIONS
- •SUGGESTED READINGS
- •17 Cell Death in the Inner Ear
- •3. THE COCHLEA IS THE HEARING ORGAN
- •3.1. Ototoxic hair cell death
- •3.2. Aminoglycoside-induced hair cell death
- •3.3. Cisplatin-induced hair cell death
- •3.4. Therapeutic strategies to prevent hair cell death
- •3.5. Challenges to studies of hair cell death
- •4. SPIRAL GANGLION NEURON DEATH
- •4.1. Neurotrophic support from sensory hair cells and supporting cells
- •4.2. Afferent activity from hair cells
- •4.3. Molecular manifestations of spiral ganglion neuron death
- •4.4. Therapeutic interventions to prevent SGN death
- •ACKNOWLEDGMENTS
- •SUGGESTED READINGS
- •18 Cell Death in the Olfactory System
- •1. Introduction
- •2. Anatomical Aspects
- •3. Life and Death in the Olfactory System
- •3.1. Olfactory epithelium
- •3.2. Olfactory bulb
- •REFERENCES
- •1. Introduction
- •3.1. Beta cell death in the development of T1D
- •3.2. Mechanisms of beta cell death in type 1 diabetes
- •3.2.1. Apoptosis signaling pathways downstream of death receptors and inflammatory cytokines
- •3.2.2. Oxidative stress
- •3.3. Mechanisms of beta cell death in type 2 diabetes
- •3.3.1. Glucolipitoxicity
- •3.3.2. Endoplasmic reticulum stress
- •5. SUMMARY
- •Acknowledgments
- •REFERENCES
- •20 Apoptosis in the Physiology and Diseases of the Respiratory Tract
- •1. APOPTOSIS IN LUNG DEVELOPMENT
- •2. APOPTOSIS IN LUNG PATHOPHYSIOLOGY
- •2.1. Apoptosis in pulmonary inflammation
- •2.2. Apoptosis in acute lung injury
- •2.3. Apoptosis in chronic obstructive pulmonary disease
- •2.4. Apoptosis in interstitial lung diseases
- •2.5. Apoptosis in pulmonary arterial hypertension
- •2.6. Apoptosis in lung cancer
- •SUGGESTED READINGS
- •21 Regulation of Cell Death in the Gastrointestinal Tract
- •1. INTRODUCTION
- •2. ESOPHAGUS
- •3. STOMACH
- •4. SMALL AND LARGE INTESTINE
- •5. LIVER
- •6. PANCREAS
- •7. SUMMARY AND CONCLUDING REMARKS
- •SUGGESTED READINGS
- •22 Apoptosis in the Kidney
- •1. NORMAL KIDNEY STRUCTURE AND FUNCTION
- •3. APOPTOSIS IN ADULT KIDNEY DISEASE
- •4. REGULATION OF APOPTOSIS IN KIDNEY CELLS
- •4.1. Survival factors
- •4.2. Lethal factors
- •4.2.1. TNF superfamily cytokines
- •4.2.2. Other cytokines
- •4.2.3. Glucose
- •4.2.4. Drugs and xenobiotics
- •4.2.5. Ischemia-reperfusion and sepsis
- •5. THERAPEUTIC APPROACHES
- •SUGGESTED READINGS
- •1. INTRODUCTION
- •2. APOPTOSIS IN THE NORMAL BREAST
- •2.1. Occurrence and role of apoptosis in the developing breast
- •2.2.2. Death ligands and death receptor pathway
- •2.2.4. LIF-STAT3 proapoptotic signaling
- •2.2.5. IGF survival signaling
- •2.2.6. Regulation by adhesion
- •2.2.7. PI3K/AKT pathway: molecular hub for survival signals
- •2.2.8. Downstream regulators of apoptosis: the BCL-2 family members
- •3. APOPTOSIS IN BREAST CANCER
- •3.1. Apoptosis in breast tumorigenesis and cancer progression
- •3.2. Molecular dysregulation of apoptosis in breast cancer
- •3.2.1. Altered expression of death ligands and their receptors in breast cancer
- •3.2.2. Deregulation of prosurvival growth factors and their receptors
- •3.2.3. Alterations in cell adhesion and resistance to anoikis
- •3.2.4. Enhanced activation of the PI3K/AKT pathway in breast cancer
- •3.2.5. p53 inactivation in breast cancer
- •3.2.6. Altered expression of BCL-2 family of proteins in breast cancer
- •5. CONCLUSION
- •SUGGESTED READINGS
- •1. INTRODUCTION
- •2. DETECTING CELL DEATH IN THE FEMALE GONADS
- •4. APOPTOSIS AND FEMALE REPRODUCTIVE AGING
- •6. CONCLUDING REMARKS
- •REFERENCES
- •25 Apoptotic Signaling in Male Germ Cells
- •1. INTRODUCTION
- •3.1. Murine models
- •3.2. Primate models
- •3.3. Pathways of caspase activation and apoptosis
- •3.4. Apoptotic signaling in male germ cells
- •5. P38 MITOGEN-ACTIVATED PROTEIN KINASE (MAPK) AND NITRIC OXIDE (NO)–MEDIATED INTRINSIC PATHWAY SIGNALING CONSTITUTES A CRITICAL COMPONENT OF APOPTOTIC SIGNALING IN MALE GERM CELLS AFTER HORMONE DEPRIVATION
- •11. CONCLUSIONS AND PERSPECTIVES
- •REFERENCES
- •26 Cell Death in the Cardiovascular System
- •1. INTRODUCTION
- •2. CELL DEATH IN THE VASCULATURE
- •2.1. Apoptosis in the developing blood vessels
- •2.2. Apoptosis in atherosclerosis
- •2.2.1. Vascular smooth muscle cells
- •2.2.2. Macrophages
- •2.2.3. Regulation of apoptosis in atherosclerosis
- •2.2.4. Necrosis and autophagy in atherosclerosis
- •3. CELL DEATH IN THE MYOCARDIUM
- •3.1. Cell death in myocardial infarction
- •3.1.1. Apoptosis in myocardial infarction
- •3.1.2. Necrosis in myocardial infarction
- •3.1.3. Autophagy in myocardial infarction
- •3.2. Cell death in heart failure
- •3.2.1. Apoptosis in heart failure
- •3.2.2. Necrosis in heart failure
- •3.2.3. Autophagy in heart failure
- •4. CONCLUDING REMARKS
- •ACKNOWLEDGMENTS
- •REFERENCES
- •27 Cell Death Regulation in Muscle
- •1. INTRODUCTION TO MUSCLE
- •1.1. Skeletal muscle adaptation to endurance training
- •1.2. Myonuclear domains
- •2. MITOCHONDRIALLY MEDIATED APOPTOSIS IN MUSCLE
- •2.1. Skeletal muscle apoptotic susceptibility
- •4. APOPTOSIS IN MUSCLE DURING AGING AND DISEASE
- •4.1. Aging
- •4.2. Type 2 diabetes mellitus
- •4.3. Cancer cachexia
- •4.4. Chronic heart failure
- •6. CONCLUSION
- •SUGGESTED READINGS
- •28 Cell Death in the Skin
- •1. INTRODUCTION
- •2. CELL DEATH IN SKIN HOMEOSTASIS
- •2.1. Cornification and apoptosis
- •2.2. Death receptors in the skin
- •3. CELL DEATH IN SKIN PATHOLOGY
- •3.1. Sunburn
- •3.2. Skin cancer
- •3.3. Necrolysis
- •3.4. Pemphigus
- •3.5. Eczema
- •3.6. Graft-versus-host disease
- •4. CONCLUDING REMARKS AND PERSPECTIVES
- •ACKNOWLEDGMENTS
- •SUGGESTED READINGS
- •29 Apoptosis and Cell Survival in the Immune System
- •2.1. Survival of early hematopoietic progenitors
- •2.2. Sizing of the T-cell population
- •2.2.1. Establishing central tolerance
- •2.2.2. Peripheral tolerance
- •2.2.3. Memory T cells
- •2.3. Control of apoptosis in B-cell development
- •2.3.1. Early B-cell development
- •2.3.2. Deletion of autoreactive B cells
- •2.3.3. Survival and death of activated B cells
- •3. IMPAIRED APOPTOSIS AND LEUKEMOGENESIS
- •4. CONCLUSIONS
- •ACKNOWLEDGMENTS
- •REFERENCES
- •30 Cell Death Regulation in the Hematopoietic System
- •1. INTRODUCTION
- •2. HEMATOPOIETIC STEM CELLS
- •4. ERYTHROPOIESIS
- •5. MEGAKARYOPOIESIS
- •6. GRANULOPOIESIS
- •7. MONOPOIESIS
- •8. CONCLUSION
- •ACKNOWLEDGMENTS
- •REFERENCES
- •31 Apoptotic Cell Death in Sepsis
- •1. INTRODUCTION
- •2. HOST INFLAMMATORY RESPONSE TO SEPSIS
- •3. CLINICAL OBSERVATIONS OF CELL DEATH IN SEPSIS
- •3.1. Sepsis-induced apoptosis
- •3.2. Necrotic cell death in sepsis
- •4.1. Central role of apoptosis in sepsis mortality: immune effector cells and gut epithelium
- •4.2. Apoptotic pathways in sepsis-induced immune cell death
- •4.3. Investigations implicating the extrinsic apoptotic pathway in sepsis
- •4.4. Investigations implicating the intrinsic apoptotic pathway in sepsis
- •5. THE EFFECT OF APOPTOSIS ON THE IMMUNE SYSTEM
- •5.1. Cellular effects of an increased apoptotic burdens
- •5.2. Network effects of selective loss of immune cell types
- •5.3. Studies of immunomodulation by apoptotic cells in other fields
- •7. CONCLUSION
- •REFERENCES
- •32 Host–Pathogen Interactions
- •1. INTRODUCTION
- •2. FROM THE PATHOGEN PERSPECTIVE
- •2.1. Commensals versus pathogens
- •2.2. Pathogen strategies to infect the host
- •3. HOST DEFENSE
- •3.1. Antimicrobial peptides
- •3.2. PRRs and inflammation
- •3.2.1. TLRs
- •3.2.2. NLRs
- •3.2.3. The Nod signalosome
- •3.2.4. The inflammasome
- •3.3. Cell death
- •3.3.1. Apoptosis and pathogen clearance
- •3.3.2. Pyroptosis
- •3.2.3. Caspase-independent cell death
- •3.2.4. Autophagy and autophagic cell death
- •4. CONCLUSIONS
- •REFERENCES
- •Part III Cell Death in Nonmammalian Organisms
- •1. PHENOTYPE AND ASSAYS OF YEAST APOPTOSIS
- •2.1. Pheromone-induced cell death
- •2.1.1. Colony growth
- •2.1.2. Killer-induced cell death
- •3. EXTERNAL STIMULI THAT INDUCE APOPTOSIS IN YEAST
- •4. THE GENETICS OF YEAST APOPTOSIS
- •5. PROGRAMMED AND ALTRUISTIC AGING
- •SUGGESTED READINGS
- •34 Caenorhabditis elegans and Apoptosis
- •1. Overview
- •2. KILLING
- •3. SPECIFICATION
- •4. EXECUTION
- •4.1. DNA degradation
- •4.2. Mitochondrial elimination
- •4.3. Engulfment
- •5. SUMMARY
- •SUGGESTED READINGS
- •35 Apoptotic Cell Death in Drosophila
- •2. DROSOPHILA CASPASES AND PROXIMAL REGULATORS
- •6. CLOSING COMMENTS
- •SUGGESTED READINGS
- •36 Analysis of Cell Death in Zebrafish
- •1. INTRODUCTION
- •2. WHY USE ZEBRAFISH TO STUDY CELL DEATH?
- •2.2. Molecular techniques to rapidly assess gene function in embryos
- •2.2.1. Studies of gene function using microinjections into early embryos
- •2.2.2. In situ hybridization and immunohistochemistry
- •2.3. Forward genetic screening
- •2.4. Drug and small-molecule screening
- •2.5. Transgenesis
- •2.6. Targeted knockouts
- •3.1. Intrinsic apoptosis
- •3.2. Extrinsic apoptosis
- •3.3. Chk-1 suppressed apoptosis
- •3.4. Anoikis
- •3.5. Autophagy
- •3.6. Necrosis
- •4. DEVELOPMENTAL CELL DEATH IN ZEBRAFISH EMBRYOS
- •5. THE P53 PATHWAY
- •6. PERSPECTIVES AND FUTURE DIRECTIONS
- •SUGGESTED READING
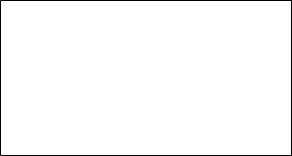
APOPTOTIC CELL DEATH IN SEPSIS |
367 |
Table 31-2. Impact of apoptosis on immune function
Decreased presentation of antigen to T cells
Decreased macrophage activation and depressed phagocytic capability
Impaired adaptive immune response
Disrupted cross-talk between adaptive and innate immune systems
Release of anti-inflammatory cytokines T-cell anergy
and increased survival in murine models of sepsis,34,35 a finding that has been recapitulated by others.43
The antiapoptotic Bcl-2 family members, of which Bcl-2 is a member, contain four Bcl-2 homology (BH) domains with sequence and structural homology. The hallmark of this protein family is the BH4 domain, a conserved N-terminal helical domain that is required for these proteins to perform their antiapoptotic functions.33 The remaining Bcl-2 family members have proapoptotic activity. These proteins comprise two subfamilies classified by their BH domain composition. A handful of proteins contain BH1-BH3 domains, and the remainder (the largest Bcl-2 subfamily) contains only the BH3 domain. The BH1-BH3 containing family members have been shown to oligomerize and permeabilize the mitochondrial outer membrane, which leads to cytochrome c release and ultimately apoptosis.33 Under basal conditions, these proteins are kept in an inactive reservoir, primarily through interactions with BH4 containing Bcl-2 family members. Although it is accepted that the BH3-only proteins trigger the activation of Bax and Bak, there is currently a controversy regarding the precise mechanism BH3 proteins employ to activate Bax/Bak.44 Regardless of the mechanism, supporters of both models have demonstrated that Bim, Bid, and p53upregulated modulator of apoptosis (PUMA) are the most potent BH-3 only proteins.33
In CLP, knockout of Bim prevented apoptotic loss of lymphocytes in sepsis and improved survival, whereas knockout of PUMA provided modest protection against apoptosis but no survival advantage.39 Bid is unique among the BH3-only proteins in that it mediates cross-talk between the extrinsic and intrinsic pathways. Caspase-8 cleaves Bid into truncated (t)Bid, which reinforces death receptor signaling by activating the intrinsic apoptotic pathway. Knockout of Bid in mice rendered lymphocytes resistant to sepsis-induced apoptosis and increased survival.45
Examination of the apoptotic pathways in sepsis reveals that there is no single mediator or pathway that is responsible for lymphocyte apoptosis. Indeed, these
data reveal that lymphocytes have extensive apoptotic machinery and that sepsis creates an environment rich in death stimuli that can activate these processes. The type of cell, its activation state and phase of the cell cycle, and type of microorganism all appear to influence whether a lymphocyte will undergo apoptosis. On the basis of findings in animal models of sepsis, the BCL-2 family plays a central role in sepsis-induced apoptosis.
5. THE EFFECT OF APOPTOSIS ON THE IMMUNE SYSTEM
Lymphocyte apoptosis may play a role in the evolution of the inflammatory response that is seen in septic patients by modulating cellular function directly and also by disrupting the network of immune cell interactions that are necessary to mount an effective immune response (Table 31-2).
5.1. Cellular effects of an increased apoptotic burdens
Apoptotic cells themselves are immunosuppressive. Macrophages and dendritic cells that take up and eliminate apoptotic cells release anti-inflammatory (Th2inducing) cytokines such as IL-10 and transforming growth factor beta (TGF-β) and suppress proinflammatory cytokines.46 T cells that come into contact with these macrophages and dendritic cells become anergized or undergo apoptosis themselves. A systemic burden of apoptotic cells has been found to worsen survival in septic mice. Mice receiving adoptive transfer of apoptotic lymphocytes before CLP have worse survival in sepsis, whereas transfer of necrotic cells improves survival.47 This differential may be due in part to how macrophages clear cellular debris and the resulting effect on IFN- γ production; phagocytic uptake of apoptotic cells by macrophages leads to a decrease IFN-γ production, whereas uptake of necrotic cells increases it.
5.2. Network effects of selective loss of immune cell types
Apoptosis of dendritic cells cripples the innate immune system by reducing the capacity to process and present antigen to the adaptive system. Loss of T and B cells disrupts the adaptive immune response and impairs the communication between the adaptive and innate arms of the immune system. The role of the adaptive immune system in sepsis has been described in studies using mice that lack T and B cells (Rag 1−/– mice). Adoptive transfer of lymphocytes over-expressing Bcl-2 to Rag−/– mice attenuates mortality owing to
368 |
PAVAN BRAHMAMDAM, JARED T. MUENZER, RICHARD S. HOTCHKISS, AND JONATHAN E. MCDUNN |
sepsis.48 Survival of immune cells in apoptosis improves survival in sepsis and may also promote the survival of other types of cells, such as neutrophils.43 Adoptive transfer of myeloid (CD11b+) cells from transgenic mice over-expressing Bcl-2 in myeloid cells was found to improve survival, decrease gut epithelial apoptosis, and increase peritoneal neutrophil counts in Rag 1−/– mice when compared with Rag 1−/– receiving cells from wild-type C57/BL6 mice. These data suggest that survival of lymphocytes and myeloid cells may exert a “bystander” effect by releasing some sort of cytoprotective molecule that inhibits apoptosis of gut epithelium and other immune effector cells.
5.3. Studies of immunomodulation by apoptotic cells in other fields
The impact of apoptotic cells in sepsis is consistent with data from the transplant literature. The immunomodulatory effects of apoptotic cells were determined in a study using infusion of apoptotic cells in mice that later received bone marrow transplants.49 The investigators found that apoptotic cell infusion caused a TGF-β dependent T-regulatory cell expansion, which in turn caused immunosuppressive effects via a cell contact mediated mechanism. In their model, the downregulation of the immune system had beneficial effects of decreasing graft-versus-host disease and increasing engraftment of donor bone marrow cells. Although beneficial in the case of bone marrow transplant, in an animal model of sepsis or in patients dying from sepsis, downregulation of the immune system could be catastrophic.
6. DEVELOPING THERAPIES TO AMELIORATE
SEPSIS-INDUCED LYMPHOCYTE APOPTOSIS
As discussed previously, research over the last decade has established the important role that immune cell apoptosis plays in the response to sepsis. These findings have encouraged the development and application of several technologies to modulate apoptosis. Two different approaches to antiapoptotic therapies are under investigation – rational therapies using biologic agents (RNA, peptides, cytokines, and proteins) and screeningbased discovery of novel cytoprotective agents. Rational therapies have been based on the knockout and transgenic mice that have well-documented survival advantages in animal models of sepsis.
Therapeutic recapitulation of the survival advantage observed in transgenic mice has required detailed molecular mechanistic understanding of apoptosis
pathways. For example, mice that over-express Bcl-2 or Bcl-xL in lymphocytes have a profound survival advantage in sepsis. Bcl-2 and Bcl-xL are cytoplasmic proteins that undergo BH4 domain-dependent trafficking from the endoplasmic reticulum to the mitochondrial surface with the help of FKBP38.50 Unfortunately, these proteins and their BH4 effector domain are unable to cross cell membranes, making them unlikely therapeutic candidates.
However, intracellular delivery of membrane-imper- meant materials was enabled by the discovery of cell permeation peptides including the Antennapedia homeodomain peptide and a short, polycationic peptide from the HIV protein Tat.51 Previous work revealed that Tat-mediated delivery of the BH4 domain of either Bcl-2 or Bcl-xL could protect cells from diverse apoptogenic stimuli, including chemotherapy,52 ischemiareperfusion injury,53,54 and radiation injury.55 Administration of antiapoptotic proteins such as BCL-2 and BCL-xl or their BH4 domains conjugated to the Tat peptide has been shown to be effective in preventing sepsisinduced lymphocyte apoptosis.56
Therapies aimed at recapitulating the phenotypes of knockout mice require inhibiting the synthesis of proapoptotic proteins. Small interfering RNA is one exciting modality that has been shown to be effective in preventing lymphocyte apoptosis and improving survival in sepsis models. siRNA to Fas, caspase-8, and Bim have all been reported to decrease sepsis-induced apoptosis and mortality after CLP.57,58 Although the basic principles of RNA interference are well worked out, clinical applications will require additional technology. Most importantly, tissue-specific delivery agents that target the biodistribution and uptake of therapeutic nucleic acids must be developed to prevent undesirable side effects. At the writing of this chapter, two approaches appear to hold promise, biologic targeting via ligand (e.g., transferrin59,60) or antibody (e.g., anti-CD461) and materials-based targeting using different polymer compositions.62,63
An alternative approach to rational biologic therapy is to develop pharmacological (small-molecule) modulators of essential apoptosis proteins. Previous work has shown that small-molecule caspase inhibitors can reduce sepsis-induced apoptosis48; however, these molecules have not entered clinical study. One potential difficulty with caspase inhibition to prevent apoptosis is that caspases have recently been found to play a key role in the cell cycle.64 Another potential challenge is that once executioner caspases are activated, cells have accumulated enough damage to no longer respond appropriately to their environment.
APOPTOTIC CELL DEATH IN SEPSIS |
369 |
Cytoprotection can be accomplished in other ways besides caspase activation; however, with the exception of Bcl-2 family members and FADD, molecular mechanisms that contravene sepsis-induced lymphocyte apoptosis are not well-established. Instead of targeting a single enzyme or protein–protein interaction, high throughput screening of compound libraries using a cell phenotype assay can be employed to discover novel cytoprotective agents that can serve as lead compounds for drug development.
Immunotherapy is also emerging as a potential therapeutic strategy for the treatment of sepsis. Administration of cytokines, such as IL-7 or IL-15, or antibodies to negative co-stimulatory molecules (Programmed Death receptor-1) have been shown to prevent apoptosis, reverse immunosupression, and improve survival in mice after CLP. Through modulation of BCL-2 and the prevention of lymphocyte apoptosis, these therapies may improve immune dysfunction seen in sepsis. 65,66,67
Although encouraging preclinical data have been developed for peptide, RNA, and small-molecule therapies, translating these findings from the laboratory to the clinic remains a significant challenge. It is likely that at least three key areas will need further research before clinical trials of antiapoptotic therapies. First, further development of lead compounds will need to be pursued. Second, we need a better understanding of how sepsis alters drug metabolism, pharmacokinetics, and pharmacodynamics. And finally, any antiapoptotic agent, even if administered a single time, has the theoretical risk of facilitating cellular transformation. Therefore, there will need to be a demonstration that shortterm treatment with a candidate antiapoptotic therapy does not significantly increase the risk of cancer.
7. CONCLUSION
From premature infants to elderly patients requiring elective surgeries, the risk of sepsis continues to be a significant cause of morbidity and mortality around the world. Over the last decade, our understanding of the pathophysiology of sepsis has grown tremendously. Most patients dying from sepsis are now thought to die during a state of “immunoparalysis,” not the initial inflammatory response. One of the key findings in the pathophysiology of sepsis has been the connection between immune effector cell apoptosis and prolonged immune suppression. Several research groups have reported dramatic improvements in survival in animal models of sepsis when apoptosis is prevented. These findings represent a significant impetus for pursuing clinical trials with antiapoptotic, immuno-
supportive agents to determine whether prevention of sepsis-induced apoptosis can change the course of disease in human patients. Hopefully, strategies to block sepsis-induced apoptosis will able to decrease the morbidity and mortality from this highly lethal disorder.
REFERENCES
1.Zeni, F., B. Freeman, and C. Natanson. Anti-inflammatory therapies to treat sepsis and septic shock: a reassessment.
Crit Care Med, 1997. 25(7): p. 1095–100.
2.Angus, D.C., et al. Epidemiology of severe sepsis in the United States: analysis of incidence, outcome, and associated costs of care. Crit Care Med, 2001. 29(7): p. 1303–10.
3.Bone, R.C., et al. Definitions for sepsis and organ failure and guidelines for the use of innovative therapies in sepsis. The ACCP/SCCM Consensus Conference Committee. American College of Chest Physicians/Society of Critical Care Medicine. Chest, 1992. 101(6): p. 1644–55.
4.Dombrovskiy, V.Y., et al. Rapid increase in hospitalization and mortality rates for severe sepsis in the United States: a trend analysis from 1993 to 2003. Crit Care Med, 2007. 35(5): p. 1244–50.
5.Girardin, E., et al. Tumor necrosis factor and interleukin-1 in the serum of children with severe infectious purpura. N Engl J Med, 1988. 319(7): p. 397–400.
6.Hotchkiss, R.S. and I.E. Karl. The pathophysiology and treatment of sepsis. N Engl J Med, 2003. 348(2): p. 138–50.
7.Monneret, G., et al. Persisting low monocyte human leukocyte antigen-DR expression predicts mortality in septic shock. Intensive Care Med, 2006. 32(8): p. 1175–83.
8.Oberholzer, A., C. Oberholzer, and L.L. Moldawer. Sepsis syndromes: understanding the role of innate and acquired immunity. Shock, 2001. 16(2): p. 83–96.
9.Remick, D.G. Pathophysiology of sepsis. Am J Pathol, 2007. 170(5): p. 1435–44.
10.Meakins, J.L., et al. Delayed hypersensitivity: indicator of acquired failure of host defenses in sepsis and trauma. Ann Surg, 1977. 186(3): p. 241–50.
11.Heidecke, C.D., et al. Selective defects of T lymphocyte function in patients with lethal intraabdominal infection.
Am J Surg, 1999. 178(4): p. 288–92.
12.Lederer, J.A., M.L. Rodrick, and J.A. Mannick. The effects of injury on the adaptive immune response. Shock, 1999. 11(3): p. 153–9.
13.Wisnoski, N., et al. The contribution of CD4+CD25+T- regulatory-cells to immune suppression in sepsis. Shock, 2007. 27(3): p. 251–7.
14.Monneret, G., et al. Marked elevation of human circulating CD4+CD25+regulatory T cells in sepsis-induced immunoparalysis. Crit Care Med, 2003. 31(7): p. 2068–71.
15.Ertel, W., et al. Interferon-gamma attenuates hemorrhageinduced suppression of macrophage and splenocyte functions and decreases susceptibility to sepsis. Surgery, 1992. 111(2): p. 177–87.
370 |
PAVAN BRAHMAMDAM, JARED T. MUENZER, RICHARD S. HOTCHKISS, AND JONATHAN E. MCDUNN |
16.Docke, W.D., et al. Monocyte deactivation in septic patients: restoration by IFN-gamma treatment. Nat Med, 1997. 3(6): p. 678–81.
17.Manjuck, J., et al. Decreased response to recall antigens is associated with depressed costimulatory receptor expression in septic critically ill patients. J Lab Clin Med, 2000. 135(2): p. 153–60.
18.Hynninen, M., et al. Predictive value of monocyte histocompatibility leukocyte antigen-DR expression and plasma interleukin-4 and -10 levels in critically ill patients with sepsis. Shock, 2003. 20(1): p. 1–4.
19.Hotchkiss, R.S., et al. Apoptotic cell death in patients with sepsis, shock, and multiple organ dysfunction. Crit Care Med, 1999. 27(7): p. 1230–51.
20.Hotchkiss, R.S., et al. Sepsis-induced apoptosis causes progressive profound depletion of B and CD4+ T lymphocytes in humans. J Immunol, 2001. 166(11): p. 6952–63.
21.Hotchkiss, R.S., et al. Depletion of dendritic cells, but not macrophages, in patients with sepsis. J Immunol, 2002. 168(5): p. 2493–500.
22.Felmet, K.A., et al. Prolonged lymphopenia, lymphoid depletion, and hypoprolactinemia in children with nosocomial sepsis and multiple organ failure. J Immunol, 2005. 174(6): p. 3765–72.
23.Toti, P., et al. Spleen depletion in neonatal sepsis and chorioamnionitis. Am J Clin Pathol, 2004. 122(5): p. 765–71.
24.Le Tulzo, Y., et al. Early circulating lymphocyte apoptosis in human septic shock is associated with poor outcome.
Shock, 2002. 18(6): p. 487–94.
25.Weber, S.U., et al. Induction of Bim and Bid gene expression during accelerated apoptosis in severe sepsis. Crit Care, 2008. 12(5): p. R128.
26.Clark, J.A. and C.M. Coopersmith. Intestinal crosstalk: a new paradigm for understanding the gut as the “motor” of critical illness. Shock, 2007. 28(4): p. 384–93.
27.Deitch, E.A. Animal models of sepsis and shock: a review and lessons learned. Shock, 1998. 9(1): p. 1–11.
28.Hubbard, W.J., et al. Cecal ligation and puncture. Shock, 2005. 24 Suppl 1: p. 52–7.
29.Mizgerd, J.P. and S.J. Skerrett. Animal models of human pneumonia. Am J Physiol Lung Cell Mol Physiol, 2008. 294(3): p. L387–98.
30.Muenzer, J.T., et al. Pneumonia after cecal ligation and puncture: a clinically relevant “two-hit” model of sepsis.
Shock, 2006. 26(6): p. 565–70.
31.Newton, K. and A. Strasser. Cell death control in lymphocytes. Adv Immunol, 2000. 76: p. 179–226.
32.Marsden, V.S. and A. Strasser. Control of apoptosis in the immune system: Bcl-2, BH3-only proteins and more. Annu Rev Immunol, 2003. 21: p. 71–105.
33.Strasser, A. The role of BH3-only proteins in the immune system. Nat Rev Immunol, 2005. 5(3): p. 189–200.
34.Hotchkiss, R.S., et al. Overexpression of Bcl-2 in transgenic mice decreases apoptosis and improves survival in sepsis.
J Immunol, 1999. 162(7): p. 4148–56.
35.Hotchkiss, R.S., et al. Prevention of lymphocyte cell death in sepsis improves survival in mice. Proc Natl Acad Sci U S A, 1999. 96(25): p. 14541–6.
36.Coopersmith, C.M., et al. Inhibition of intestinal epithelial apoptosis and survival in a murine model of pneumoniainduced sepsis. JAMA, 2002. 287(13): p. 1716–21.
37.Coopersmith, C.M., et al. Sepsis from Pseudomonas aeruginosa pneumonia decreases intestinal proliferation and induces gut epithelial cell cycle arrest. Crit Care Med, 2003. 31(6): p. 1630–7.
38.Hotchkiss, R.S., et al. Accelerated lymphocyte death in sepsis occurs by both the death receptor and mitochondrial pathways. J Immunol, 2005. 174(8): p. 5110–8.
39.Chang, K.C., et al. Multiple triggers of cell death in sepsis: death receptor and mitochondrial-mediated apoptosis. FASEB J, 2007. 21(3): p. 708–19.
40.Chung, C.S., et al. Inhibition of Fas/Fas ligand signaling improves septic survival: differential effects on macrophage apoptotic and functional capacity. J Leukoc Biol, 2003. 74(3): p. 344–51.
41.Ayala, A., et al. Increased inducible apoptosis in CD4+ T lymphocytes during polymicrobial sepsis is mediated by Fas ligand and not endotoxin. Immunology, 1999. 97(1):
p.45–55.
42.Peck-Palmer, O.M., et al. Deletion of MyD88 markedly attenuates sepsis-induced T and B lymphocyte apoptosis but worsens survival. J Leukoc Biol, 2008. 83(4): p. 1009–18.
43.Iwata, A., et al. Over-expression of Bcl-2 provides protection in septic mice by a trans effect. J Immunol, 2003. 171(6): p. 3136–41.
44.Fletcher, J.I. and D.C. Huang. Controlling the cell death mediators Bax and Bak: puzzles and conundrums. Cell Cycle, 2008. 7(1): p. 39–44.
45.Yin, X.M., et al. Bid-deficient mice are resistant to Fasinduced hepatocellular apoptosis. Nature, 1999. 400(6747):
p.886–91.
46.Albert, M.L. Death-defying immunity: do apoptotic cells influence antigen processing and presentation? Nat Rev Immunol, 2004. 4(3): p. 223–31.
47.Hotchkiss, R.S., et al. Adoptive transfer of apoptotic splenocytes worsens survival, whereas adoptive transfer of necrotic splenocytes improves survival in sepsis. Proc Natl Acad Sci U S A, 2003. 100(11): p. 6724–9.
48.Hotchkiss, R.S., et al. Caspase inhibitors improve survival in sepsis: a critical role of the lymphocyte. Nat Immunol, 2000. 1(6): p. 496–501.
49.Kleinclauss, F., et al. Intravenous apoptotic spleen cell infusion induces a TGF-beta-dependent regulatory T-cell expansion. Cell Death Differ, 2006. 13(1): p. 41–52.
50.Shirane, M. and K.I. Nakayama. Inherent calcineurin inhibitor FKBP38 targets Bcl-2 to mitochondria and inhibits apoptosis. Nat Cell Biol, 2003. 5(1): p. 28–37.
51.Gump, J.M. and S.F. Dowdy. TAT transduction: the molecular mechanism and therapeutic prospects. Trends Mol Med, 2007. 13(10): p. 443–8.
APOPTOTIC CELL DEATH IN SEPSIS |
371 |
52.Shimizu, S., et al. BH4 domain of antiapoptotic Bcl-2 family members closes voltage-dependent anion channel and inhibits apoptotic mitochondrial changes and cell death.
Proc Natl Acad Sci U S A, 2000. 97(7): p. 3100–5.
53.Sugioka, R., et al. BH4-domain peptide from Bcl-xL exerts anti-apoptotic activity in vivo. Oncogene, 2003. 22(52): p. 8432–40.
54.Chen, M., et al. Calpain and mitochondria in ischemia/ reperfusion injury. J Biol Chem, 2002. 277(32): p. 29181–6.
55.McConnell, K.W., et al. Anti-apoptotic peptides protect against radiation-induced cell death. Biochem Biophys Res Commun, 2007. 355(2): p. 501–7.
56.Hotchkiss, R.S., et al. TAT-BH4 and TAT-Bcl-xL peptides protect against sepsis-induced lymphocyte apoptosis in vivo. J Immunol, 2006. 176(9): p. 5471–7.
57.Wesche-Soldato, D.E., et al. In vivo delivery of caspase-8 or Fas siRNA improves the survival of septic mice. Blood, 2005. 106(7): p. 2295–301.
58.Schwulst, S.J., et al. Bim siRNA decreases lymphocyte apoptosis and improves survival in sepsis. Shock, 2008. 30(2): p. 127–34.
59.Heidel, J.D., et al. Administration in non-human primates of escalating intravenous doses of targeted nanoparticles containing ribonucleotide reductase subunit M2 siRNA.
Proc Natl Acad Sci U S A, 2007. 104(14): p. 5715–21.
60.Brahmamdam, P., et al. Targeted delivery of siRNA to cell death proteins in sepsis. Shock, 2008. 32(2): p. 131–9.
61.Song, E., et al. Antibody mediated in vivo delivery of small interfering RNAs via cell-surface receptors. Nat Biotechnol, 2005. 23(6): p. 709–17.
62.Thomas, M., et al. Identification of novel superior polycationic vectors for gene delivery by high-throughput synthesis and screening of a combinatorial library. Pharm Res, 2007. 24(8): p. 1564–71.
63.Akinc, A., et al. A combinatorial library of lipid-like materials for delivery of RNAi therapeutics. Nat Biotechnol, 2008. 26(5): p. 561–9.
64.Woo, M., et al. Caspase-3 regulates cell cycle in B cells: a consequence of substrate specificity. Nat Immunol, 2003. 4(10): p. 1016–22.
65.Unsinger, J., et al. IL-7 promotes T cell viability, trafficking, and functionality and improves survival in sepsis. J Immunol, 2010. 184(7):p. 3768–79.
66.Inoue, S., et al. IL-15 prevents apoptosis, reverses innate and adaptive immune dysfunction, and improves survival in sepsis. J Immunolol, 2010. 184(3): p. 1401–9.
67.Brahmamdam, P., et al. Delayed administration of anti-PD-1 antibody reverses immune dysfunction and improves survival during sepsis. J Leukoc Biol, 2010. 88(2): p. 233–40.