
- •Contents
- •Contributors
- •Part I General Principles of Cell Death
- •1 Human Caspases – Apoptosis and Inflammation Signaling Proteases
- •1.1. Apoptosis and limited proteolysis
- •1.2. Caspase evolution
- •2. ACTIVATION MECHANISMS
- •2.2. The activation platforms
- •2.4. Proteolytic maturation
- •3. CASPASE SUBSTRATES
- •4. REGULATION BY NATURAL INHIBITORS
- •REFERENCES
- •2 Inhibitor of Apoptosis Proteins
- •2. CELLULAR FUNCTIONS AND PHENOTYPES OF IAP
- •3. IN VIVO FUNCTIONS OF IAP FAMILY PROTEINS
- •4. SUBCELLULAR LOCATIONS OF IAP
- •8. IAP–IAP INTERACTIONS
- •10. ENDOGENOUS ANTAGONISTS OF IAP
- •11. IAPs AND DISEASE
- •SUGGESTED READINGS
- •1. INTRODUCTION
- •2.1. The CD95 (Fas/APO-1) system
- •2.1.1. CD95 and CD95L: discovery of the first direct apoptosis-inducing receptor-ligand system
- •2.1.2. Biochemistry of CD95 apoptosis signaling
- •2.2. The TRAIL (Apo2L) system
- •3.1. The TNF system
- •3.1.1. Biochemistry of TNF signal transduction
- •3.1.2. TNF and TNF blockers in the clinic
- •3.2. The DR3 system
- •4. THE DR6 SYSTEM
- •6. CONCLUDING REMARKS AND OUTLOOK
- •SUGGESTED READINGS
- •4 Mitochondria and Cell Death
- •1. INTRODUCTION
- •2. MITOCHONDRIAL PHYSIOLOGY
- •3. THE MITOCHONDRIAL PATHWAY OF APOPTOSIS
- •9. CONCLUSIONS
- •SUGGESTED READINGS
- •1. INTRODUCTION
- •3. INHIBITING APOPTOSIS
- •4. INHIBITING THE INHIBITORS
- •6. THE BCL-2 FAMILY AND CANCER
- •SUGGESTED READINGS
- •6 Endoplasmic Reticulum Stress Response in Cell Death and Cell Survival
- •1. INTRODUCTION
- •2. THE ESR IN YEAST
- •3. THE ESR IN MAMMALS
- •4. THE ESR AND CELL DEATH
- •5. THE ESR IN DEVELOPMENT AND TISSUE HOMEOSTASIS
- •6. THE ESR IN HUMAN DISEASE
- •7. CONCLUSION
- •7 Autophagy – The Liaison between the Lysosomal System and Cell Death
- •1. INTRODUCTION
- •2. AUTOPHAGY
- •2.2. Physiologic functions of autophagy
- •2.3. Autophagy and human pathology
- •3. AUTOPHAGY AND CELL DEATH
- •3.1. Autophagy as anti–cell death mechanism
- •3.2. Autophagy as a cell death mechanism
- •3.3. Molecular players of the autophagy–cell death cross-talk
- •4. AUTOPHAGY, CELLULAR DEATH, AND CANCER
- •5. CONCLUDING REMARKS AND PENDING QUESTIONS
- •SUGGESTED READINGS
- •8 Cell Death in Response to Genotoxic Stress and DNA Damage
- •1. TYPES OF DNA DAMAGE AND REPAIR SYSTEMS
- •2. DNA DAMAGE RESPONSE
- •2.2. Transducers
- •2.3. Effectors
- •4. CHROMATIN MODIFICATIONS
- •5. CELL CYCLE CHECKPOINT REGULATION
- •6. WHEN REPAIR FAILS: SENESCENCE VERSUS APOPTOSIS
- •6.1. DNA damage response and the induction of apoptosis
- •6.2. p53-independent mechanisms of apoptosis
- •6.3. DNA damage response and senescence induction
- •7. DNA DAMAGE FROM OXIDATIVE STRESS
- •SUGGESTED READINGS
- •9 Ceramide and Lipid Mediators in Apoptosis
- •1. INTRODUCTION
- •3.1. Basic cell signaling often involves small molecules
- •3.2. Sphingolipids are cell-signaling molecules
- •3.2.1. Ceramide induces apoptosis
- •3.2.2. Ceramide accumulates during programmed cell death
- •3.2.3. Inhibition of ceramide production alters cell death signaling
- •4.1. Ceramide is generated through SM hydrolysis
- •4.3. aSMase can be activated independently of extracellular receptors to regulate apoptosis
- •4.4. Controversial aspects of the role of aSMase in apoptosis
- •4.5. De novo ceramide synthesis regulates programmed cell death
- •4.6. p53 and Bcl-2–like proteins are connected to de novo ceramide synthesis
- •4.7. The role and regulation of de novo synthesis in ceramide-mediated cell death is poorly understood
- •5. CONCLUDING REMARKS AND FUTURE DIRECTIONS
- •5.1. Who? (Which enzyme?)
- •5.2. What? (Which ceramide?)
- •5.3. Where? (Which compartment?)
- •5.4. When? (At what steps?)
- •5.5. How? (Through what mechanisms?)
- •5.6. What purpose?
- •6. SUMMARY
- •SUGGESTED READINGS
- •1. General Introduction
- •1.1. Cytotoxic lymphocytes and apoptosis
- •2. CYTOTOXIC GRANULES AND GRANULE EXOCYTOSIS
- •2.1. Synthesis and loading of the cytotoxic granule proteins into the secretory granules
- •2.2. The immunological synapse
- •2.3. Secretion of granule proteins
- •2.4. Uptake of proapoptotic proteins into the target cell
- •2.5. Activation of death pathways by granzymes
- •3. GRANULE-BOUND CYTOTOXIC PROTEINS
- •3.1. Perforin
- •3.2. Granulysin
- •3.3. Granzymes
- •3.3.1. GrB-mediated apoptosis
- •3.3.2. GrA-mediated cell death
- •3.3.3. Orphan granzyme-mediated cell death
- •5. CONCLUSIONS
- •REFERENCES
- •Part II Cell Death in Tissues and Organs
- •1.1. Death by trophic factor deprivation
- •1.2. Key molecules regulating neuronal apoptosis during development
- •1.2.1. Roles of caspases and Apaf-1 in neuronal cell death
- •1.2.2. Role of Bcl-2 family members in neuronal cell death
- •1.3. Signal transduction from neurotrophins and neurotrophin receptors
- •1.3.1. Signals for survival
- •1.3.2. Signals for death
- •2.1. Apoptosis in neurodegenerative diseases
- •2.1.4. Amyotrophic lateral sclerosis
- •2.2. Necrotic cell death in neurodegenerative diseases
- •2.2.1. Calpains
- •2.2.2. Cathepsins
- •3. CONCLUSIONS
- •ACKNOWLEDGMENT
- •SUGGESTED READINGS
- •ACKNOWLEDGMENT
- •SUGGESTED READINGS
- •1. INTRODUCTION
- •5. S-NITROSYLATION OF PARKIN
- •7. POTENTIAL TREATMENT OF EXCESSIVE NMDA-INDUCED Ca2+ INFLUX AND FREE RADICAL GENERATION
- •8. FUTURE THERAPEUTICS: NITROMEMANTINES
- •9. CONCLUSIONS
- •Acknowledgments
- •SUGGESTED READINGS
- •3. MITOCHONDRIAL PERMEABILITY TRANSITION ACTIVATED BY Ca2+ AND OXIDATIVE STRESS
- •4.1. Mitochondrial apoptotic pathways
- •4.2. Bcl-2 family proteins
- •4.3. Caspase-dependent apoptosis
- •4.4. Caspase-independent apoptosis
- •4.5. Calpains in ischemic neural cell death
- •5. SUMMARY
- •ACKNOWLEDGMENTS
- •SUGGESTED READINGS
- •1. INTRODUCTION
- •2. HISTORICAL ANTECEDENTS
- •7.1. Activation of p21 waf1/cip1: Targeting extrinsic and intrinsic pathways to death
- •8. CONCLUSION
- •ACKNOWLEDGMENTS
- •REFERENCES
- •16 Apoptosis and Homeostasis in the Eye
- •1.1. Lens
- •1.2. Retina
- •2. ROLE OF APOPTOSIS IN DISEASES OF THE EYE
- •2.1. Glaucoma
- •2.2. Age-related macular degeneration
- •4. APOPTOSIS AND OCULAR IMMUNE PRIVILEGE
- •5. CONCLUSIONS
- •SUGGESTED READINGS
- •17 Cell Death in the Inner Ear
- •3. THE COCHLEA IS THE HEARING ORGAN
- •3.1. Ototoxic hair cell death
- •3.2. Aminoglycoside-induced hair cell death
- •3.3. Cisplatin-induced hair cell death
- •3.4. Therapeutic strategies to prevent hair cell death
- •3.5. Challenges to studies of hair cell death
- •4. SPIRAL GANGLION NEURON DEATH
- •4.1. Neurotrophic support from sensory hair cells and supporting cells
- •4.2. Afferent activity from hair cells
- •4.3. Molecular manifestations of spiral ganglion neuron death
- •4.4. Therapeutic interventions to prevent SGN death
- •ACKNOWLEDGMENTS
- •SUGGESTED READINGS
- •18 Cell Death in the Olfactory System
- •1. Introduction
- •2. Anatomical Aspects
- •3. Life and Death in the Olfactory System
- •3.1. Olfactory epithelium
- •3.2. Olfactory bulb
- •REFERENCES
- •1. Introduction
- •3.1. Beta cell death in the development of T1D
- •3.2. Mechanisms of beta cell death in type 1 diabetes
- •3.2.1. Apoptosis signaling pathways downstream of death receptors and inflammatory cytokines
- •3.2.2. Oxidative stress
- •3.3. Mechanisms of beta cell death in type 2 diabetes
- •3.3.1. Glucolipitoxicity
- •3.3.2. Endoplasmic reticulum stress
- •5. SUMMARY
- •Acknowledgments
- •REFERENCES
- •20 Apoptosis in the Physiology and Diseases of the Respiratory Tract
- •1. APOPTOSIS IN LUNG DEVELOPMENT
- •2. APOPTOSIS IN LUNG PATHOPHYSIOLOGY
- •2.1. Apoptosis in pulmonary inflammation
- •2.2. Apoptosis in acute lung injury
- •2.3. Apoptosis in chronic obstructive pulmonary disease
- •2.4. Apoptosis in interstitial lung diseases
- •2.5. Apoptosis in pulmonary arterial hypertension
- •2.6. Apoptosis in lung cancer
- •SUGGESTED READINGS
- •21 Regulation of Cell Death in the Gastrointestinal Tract
- •1. INTRODUCTION
- •2. ESOPHAGUS
- •3. STOMACH
- •4. SMALL AND LARGE INTESTINE
- •5. LIVER
- •6. PANCREAS
- •7. SUMMARY AND CONCLUDING REMARKS
- •SUGGESTED READINGS
- •22 Apoptosis in the Kidney
- •1. NORMAL KIDNEY STRUCTURE AND FUNCTION
- •3. APOPTOSIS IN ADULT KIDNEY DISEASE
- •4. REGULATION OF APOPTOSIS IN KIDNEY CELLS
- •4.1. Survival factors
- •4.2. Lethal factors
- •4.2.1. TNF superfamily cytokines
- •4.2.2. Other cytokines
- •4.2.3. Glucose
- •4.2.4. Drugs and xenobiotics
- •4.2.5. Ischemia-reperfusion and sepsis
- •5. THERAPEUTIC APPROACHES
- •SUGGESTED READINGS
- •1. INTRODUCTION
- •2. APOPTOSIS IN THE NORMAL BREAST
- •2.1. Occurrence and role of apoptosis in the developing breast
- •2.2.2. Death ligands and death receptor pathway
- •2.2.4. LIF-STAT3 proapoptotic signaling
- •2.2.5. IGF survival signaling
- •2.2.6. Regulation by adhesion
- •2.2.7. PI3K/AKT pathway: molecular hub for survival signals
- •2.2.8. Downstream regulators of apoptosis: the BCL-2 family members
- •3. APOPTOSIS IN BREAST CANCER
- •3.1. Apoptosis in breast tumorigenesis and cancer progression
- •3.2. Molecular dysregulation of apoptosis in breast cancer
- •3.2.1. Altered expression of death ligands and their receptors in breast cancer
- •3.2.2. Deregulation of prosurvival growth factors and their receptors
- •3.2.3. Alterations in cell adhesion and resistance to anoikis
- •3.2.4. Enhanced activation of the PI3K/AKT pathway in breast cancer
- •3.2.5. p53 inactivation in breast cancer
- •3.2.6. Altered expression of BCL-2 family of proteins in breast cancer
- •5. CONCLUSION
- •SUGGESTED READINGS
- •1. INTRODUCTION
- •2. DETECTING CELL DEATH IN THE FEMALE GONADS
- •4. APOPTOSIS AND FEMALE REPRODUCTIVE AGING
- •6. CONCLUDING REMARKS
- •REFERENCES
- •25 Apoptotic Signaling in Male Germ Cells
- •1. INTRODUCTION
- •3.1. Murine models
- •3.2. Primate models
- •3.3. Pathways of caspase activation and apoptosis
- •3.4. Apoptotic signaling in male germ cells
- •5. P38 MITOGEN-ACTIVATED PROTEIN KINASE (MAPK) AND NITRIC OXIDE (NO)–MEDIATED INTRINSIC PATHWAY SIGNALING CONSTITUTES A CRITICAL COMPONENT OF APOPTOTIC SIGNALING IN MALE GERM CELLS AFTER HORMONE DEPRIVATION
- •11. CONCLUSIONS AND PERSPECTIVES
- •REFERENCES
- •26 Cell Death in the Cardiovascular System
- •1. INTRODUCTION
- •2. CELL DEATH IN THE VASCULATURE
- •2.1. Apoptosis in the developing blood vessels
- •2.2. Apoptosis in atherosclerosis
- •2.2.1. Vascular smooth muscle cells
- •2.2.2. Macrophages
- •2.2.3. Regulation of apoptosis in atherosclerosis
- •2.2.4. Necrosis and autophagy in atherosclerosis
- •3. CELL DEATH IN THE MYOCARDIUM
- •3.1. Cell death in myocardial infarction
- •3.1.1. Apoptosis in myocardial infarction
- •3.1.2. Necrosis in myocardial infarction
- •3.1.3. Autophagy in myocardial infarction
- •3.2. Cell death in heart failure
- •3.2.1. Apoptosis in heart failure
- •3.2.2. Necrosis in heart failure
- •3.2.3. Autophagy in heart failure
- •4. CONCLUDING REMARKS
- •ACKNOWLEDGMENTS
- •REFERENCES
- •27 Cell Death Regulation in Muscle
- •1. INTRODUCTION TO MUSCLE
- •1.1. Skeletal muscle adaptation to endurance training
- •1.2. Myonuclear domains
- •2. MITOCHONDRIALLY MEDIATED APOPTOSIS IN MUSCLE
- •2.1. Skeletal muscle apoptotic susceptibility
- •4. APOPTOSIS IN MUSCLE DURING AGING AND DISEASE
- •4.1. Aging
- •4.2. Type 2 diabetes mellitus
- •4.3. Cancer cachexia
- •4.4. Chronic heart failure
- •6. CONCLUSION
- •SUGGESTED READINGS
- •28 Cell Death in the Skin
- •1. INTRODUCTION
- •2. CELL DEATH IN SKIN HOMEOSTASIS
- •2.1. Cornification and apoptosis
- •2.2. Death receptors in the skin
- •3. CELL DEATH IN SKIN PATHOLOGY
- •3.1. Sunburn
- •3.2. Skin cancer
- •3.3. Necrolysis
- •3.4. Pemphigus
- •3.5. Eczema
- •3.6. Graft-versus-host disease
- •4. CONCLUDING REMARKS AND PERSPECTIVES
- •ACKNOWLEDGMENTS
- •SUGGESTED READINGS
- •29 Apoptosis and Cell Survival in the Immune System
- •2.1. Survival of early hematopoietic progenitors
- •2.2. Sizing of the T-cell population
- •2.2.1. Establishing central tolerance
- •2.2.2. Peripheral tolerance
- •2.2.3. Memory T cells
- •2.3. Control of apoptosis in B-cell development
- •2.3.1. Early B-cell development
- •2.3.2. Deletion of autoreactive B cells
- •2.3.3. Survival and death of activated B cells
- •3. IMPAIRED APOPTOSIS AND LEUKEMOGENESIS
- •4. CONCLUSIONS
- •ACKNOWLEDGMENTS
- •REFERENCES
- •30 Cell Death Regulation in the Hematopoietic System
- •1. INTRODUCTION
- •2. HEMATOPOIETIC STEM CELLS
- •4. ERYTHROPOIESIS
- •5. MEGAKARYOPOIESIS
- •6. GRANULOPOIESIS
- •7. MONOPOIESIS
- •8. CONCLUSION
- •ACKNOWLEDGMENTS
- •REFERENCES
- •31 Apoptotic Cell Death in Sepsis
- •1. INTRODUCTION
- •2. HOST INFLAMMATORY RESPONSE TO SEPSIS
- •3. CLINICAL OBSERVATIONS OF CELL DEATH IN SEPSIS
- •3.1. Sepsis-induced apoptosis
- •3.2. Necrotic cell death in sepsis
- •4.1. Central role of apoptosis in sepsis mortality: immune effector cells and gut epithelium
- •4.2. Apoptotic pathways in sepsis-induced immune cell death
- •4.3. Investigations implicating the extrinsic apoptotic pathway in sepsis
- •4.4. Investigations implicating the intrinsic apoptotic pathway in sepsis
- •5. THE EFFECT OF APOPTOSIS ON THE IMMUNE SYSTEM
- •5.1. Cellular effects of an increased apoptotic burdens
- •5.2. Network effects of selective loss of immune cell types
- •5.3. Studies of immunomodulation by apoptotic cells in other fields
- •7. CONCLUSION
- •REFERENCES
- •32 Host–Pathogen Interactions
- •1. INTRODUCTION
- •2. FROM THE PATHOGEN PERSPECTIVE
- •2.1. Commensals versus pathogens
- •2.2. Pathogen strategies to infect the host
- •3. HOST DEFENSE
- •3.1. Antimicrobial peptides
- •3.2. PRRs and inflammation
- •3.2.1. TLRs
- •3.2.2. NLRs
- •3.2.3. The Nod signalosome
- •3.2.4. The inflammasome
- •3.3. Cell death
- •3.3.1. Apoptosis and pathogen clearance
- •3.3.2. Pyroptosis
- •3.2.3. Caspase-independent cell death
- •3.2.4. Autophagy and autophagic cell death
- •4. CONCLUSIONS
- •REFERENCES
- •Part III Cell Death in Nonmammalian Organisms
- •1. PHENOTYPE AND ASSAYS OF YEAST APOPTOSIS
- •2.1. Pheromone-induced cell death
- •2.1.1. Colony growth
- •2.1.2. Killer-induced cell death
- •3. EXTERNAL STIMULI THAT INDUCE APOPTOSIS IN YEAST
- •4. THE GENETICS OF YEAST APOPTOSIS
- •5. PROGRAMMED AND ALTRUISTIC AGING
- •SUGGESTED READINGS
- •34 Caenorhabditis elegans and Apoptosis
- •1. Overview
- •2. KILLING
- •3. SPECIFICATION
- •4. EXECUTION
- •4.1. DNA degradation
- •4.2. Mitochondrial elimination
- •4.3. Engulfment
- •5. SUMMARY
- •SUGGESTED READINGS
- •35 Apoptotic Cell Death in Drosophila
- •2. DROSOPHILA CASPASES AND PROXIMAL REGULATORS
- •6. CLOSING COMMENTS
- •SUGGESTED READINGS
- •36 Analysis of Cell Death in Zebrafish
- •1. INTRODUCTION
- •2. WHY USE ZEBRAFISH TO STUDY CELL DEATH?
- •2.2. Molecular techniques to rapidly assess gene function in embryos
- •2.2.1. Studies of gene function using microinjections into early embryos
- •2.2.2. In situ hybridization and immunohistochemistry
- •2.3. Forward genetic screening
- •2.4. Drug and small-molecule screening
- •2.5. Transgenesis
- •2.6. Targeted knockouts
- •3.1. Intrinsic apoptosis
- •3.2. Extrinsic apoptosis
- •3.3. Chk-1 suppressed apoptosis
- •3.4. Anoikis
- •3.5. Autophagy
- •3.6. Necrosis
- •4. DEVELOPMENTAL CELL DEATH IN ZEBRAFISH EMBRYOS
- •5. THE P53 PATHWAY
- •6. PERSPECTIVES AND FUTURE DIRECTIONS
- •SUGGESTED READING
226 |
CHRISTIAN TAUBE AND MARTIN SCHULER |
in tumor biopsies correlates with improved outcome of NSCLC patients undergoing chemotherapy. Hence the functionality of apoptotic signal transduction pathways appears to determine – at least in part – the preclinical and clinical efficacy of cytotoxic and molecularly targeted lung cancer therapies.
Although most, if not all, current anticancer drugs primarily induce apoptosis via the intrinsic pathway of caspase activation, the extrinsic pathway also provides targets for lung cancer therapy. The TNF-related apoptosis-inducing ligand (TRAIL) and its receptors are of particular interest, as recombinant TRAIL exhibits antitumor activity in preclinical models, including lung cancer. Whereas TRAIL monotherapy has modest activity, it highly sensitizes xenografted lung cancers to cytotoxic drug-induced apoptosis in vivo. In agreement, the anti-TRAIL receptor antibody mapatumumab has no apparent antitumor activity in patients with advanced lung cancers; however, due to its favorable tolerability, further development in combination therapies seems warranted. In summary, further understanding of the role of apoptosis in lung cancer will likely improve therapeutic options and outcome of patients suffering from this devastating disease.
SUGGESTED READINGS
ATS/ERS (2002). American Thoracic Society/European Respiratory Society International Multidisciplinary Consensus Classification of the Idiopathic Interstitial Pneumonias. This joint statement of the American Thoracic Society (ATS), and the European Respiratory Society (ERS) was adopted by the ATS board of directors, June 2001 and by the ERS Executive Committee, June 2001. Am J Respir Crit Care Med. 165, 277–304.
Albertine, K.H., Soulier, M.F., Wang, Z., Ishizaka, A., Hashimoto, S., Zimmerman, G.A., Matthay, M.A., and Ware, L.B. (2002). Fas and fas ligand are up-regulated in pulmonary edema fluid and lung tissue of patients with acute lung injury and the acute respiratory distress syndrome. Am J Pathol. 161, 1783– 96.
Aoshiba, K., Yokohori, N., and Nagai, A. (2003). Alveolar wall apoptosis causes lung destruction and emphysematous changes. Am J Respir Cell Mol Biol. 28, 555–62.
Apolinario, R.M., Van Der Valk, P., de Jong, J.S., Deville, W., van Ark-Otte, J., Dingemans, A.-M.C., van Mourik, J.C., Postmus, P.E., Pinedo, H.M., and Giaccone, G. (1997). Prognostic value of the expression of p53, bcl-2, and bax oncoproteins, and neovascularization in patients with radically resected nonsmall cell lung cancer. J Clin Oncol. 15, 2456–66.
Ashkenazi, A., Pai, R.C., Fong, S., Leung, S., Lawrence, D.A., Marsters, S.A., Blackie, C., Chang, L., McMurtrey, A.E., Hebert, A., DeForge, L., Koumenis, I.L., Lewis, D., Harris, L., Koeppen, H., Shahrokh, Z., and Schwall, R.H. (1999). Safety and antitu-
mor activity of recombinant soluble Apo2 ligand. J Clin Invest. 104, 155–62.
Barbas-Filho, J.V., Ferreira, M.A., Sesso, A., Kairalla, R.A., Carvalho, C.R., and Capelozzi, V.L. (2001). Evidence of type II pneumocyte apoptosis in the pathogenesis of idiopathic pulmonary fibrosis (IFP)/usual interstitial pneumonia (UIP).
J Clin Pathol. 54, 132–8.
Barnes, P.J., Shapiro, S.D., and Pauwels, R.A. (2003). Chronic obstructive pulmonary disease: molecular and cellular mechanisms. Eur Respir J. 22, 672–88.
Beer, D.G., Kardia, S.L.R., Huang, C.-C., Giordano, T.J., Levin, A.M., Misek, D.E., Lin, L., Gharib, T.G., Thomas, D.G., Lizyness, M.L., Kuick, R., Hayasaka, S., Taylor, J.M.G., Iannettoni, M.D., Orringer, M.B., and Hanash, S. (2002). Gene-expression profiles predict survial of patients with lung adenocarcinoma.
Nat Med. 8, 816–824.
Besse, B., Cande,´ C., Spano, J.P., Martin, A., Khayat, D., Le Chevalier, T., Tursz, T., Sabatier, L., Soria, J.C., and Kroemer, G. (2004). Nuclear localization of apoptosis protease activating factor-1 predicts survival after tumor resection in earlystage non-small cell lung cancer. Clin Cancer Res. 10, 5665–9.
Bruce, M.C., Honaker, C.E., and Cross, R.J. (1999). Lung fibroblasts undergo apoptosis following alveolarization. Am J Respir Cell Mol Biol. 20, 228–36.
Bull, T.M., Coldren, C.D., Geraci, M.W., and Voelkel, N.F. (2007). Gene expression profiling in pulmonary hypertension. Proc Am Thorac Soc. 4, 117–20.
Bykov, V.J.N., Issaeva, N., Shilov, A., Multcrantz, M., Pugacheva, E., Chumakov, P., Bergman, J., Wiman, K.G., and Selivanova, G. (2002). Restoration of the tumor suppressor function to mutant p53 by a low-molecular-weight compound. Nat Med. 8, 282–8.
Calabrese, F., Giacometti, C., Beghe, B., Rea, F., Loy, M., Zuin, R., Marulli, G., Baraldo, S., Saetta, M., and Valente, M. (2005). Marked alveolar apoptosis/proliferation imbalance in endstage emphysema. Respir Res. 6:14., 14.
Checinska, A., Hoogeland, B.S., Rodriguez, J.A., Giaccone, G., and Kruyt, F.A. (2007). Role of XIAP in inhibiting cisplatininduced caspase activation in non-small cell lung cancer cells: a small molecule Smac mimic sensitizes for chemotherapy-induced apoptosis by enhancing caspase-3 activation. Exp Cell Res. 313, 1215–24.
Chipuk, J.E., Maurer, U., Green, D.R., and Schuler, M. (2003). Pharmacologic activation of p53 elicits Bax-dependent apoptosis in the absence of transcription. Cancer Cell 4, 371–381.
Cvetanovic, M., Mitchell, J.E., Patel, V., Avner, B.S., Su, Y., Van Der Saag, P.T., Witte, P.L., Fiore, S., Levine, J.S., and Ucker, D.S. (2006). Specific recognition of apoptotic cells reveals a ubiquitous and unconventional innate immunity. J Biol Chem. 281, 20055–67.
De Paepe, M.E., Johnson, B.D., Papadakis, K., and Luks, F.I. (1999a). Lung growth response after tracheal occlusion in fetal rabbits is gestational age-dependent. Am J Respir Cell Mol Biol. 21, 65–76.
De Paepe, M.E., Johnson, B.D., Papadakis, K., Sueishi, K., and Luks, F.I. (1998). Temporal pattern of accelerated lung growth
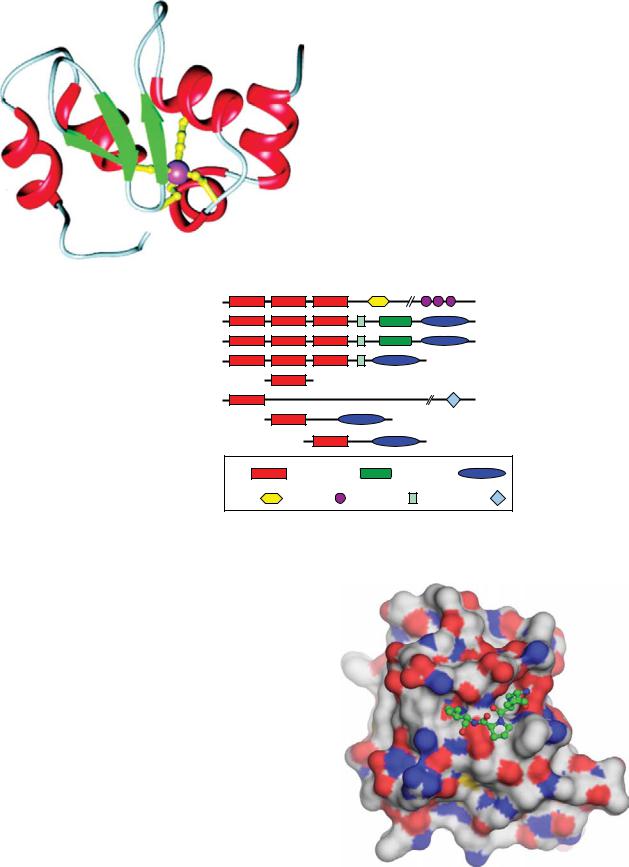
Plate 1. 3D structure of XIAP BIR3. See Figure 2-1 for details.
NAIP/BIRC1 |
|
|
1403 |
c-IAP1/BIRC2 |
|
|
604 |
c-IAP2/BIRC3 |
|
|
612 |
XIAP/BIRC4 |
|
|
497 |
Survivin/BIRC5 |
|
|
142 |
Apollon/Bruce/BIRC6 |
|
|
4830 |
Livin/ML-IAP/BIRC7 |
|
|
298 |
Ts-IAP/ILP-2/BIRC8 |
|
|
237 |
BIR |
CARD |
|
RING |
NBD |
LRR |
UBA |
UBC |
Plate 2. Domain organization of the human IAP protein family. See Figure 2-2 for details.
Plate 3. Structure of the XIAP BIR3 domain complexed with SMAC tetrapeptide. SMAC peptide bound to BIR3 of XIAP. The BIR3 domain of XIAP (shown as a space-filling model) complexed with the SMAC tetrapeptide, AVPI. See Figure 2-4 for details.

CD95 and TRAIL signaling complex
|
|
|
FADD |
Bax |
|
|
|
|
|
c-Flip |
|
Bak |
|
|
|
mitochondria |
tBid |
Bid |
caspase-8/10 |
|
|||
Cytochrome C |
|
|
ac ve caspase-8/10 |
Apaf-1 |
|
|
|
apoptosome |
ac ve caspase-9 |
ac ve caspase-3 |
Smac/DIABLO
XIAP
Apoptosis
Plate 4. Schematic representation of apoptotic signaling by the CD95 and TRAIL systems. See Figure 3-2 for details.
TNF-R1 signaling complex
RIP1 |
TRADD |
|
TRAF2/5 |
TAB2 |
|
TAK1 |
|
TAB1 |
|
NEMO IKKβ |
cIAP1/2 |
|
|
IKKα |
|
NF-κB JNK p38
Gene induction
Plate 5. Schematic representation of immunostimulatory, pro-inflammatory signaling by the TNF-R and DR3 systems. Binding of TNF and TL1A to their respective receptor leads to receptor trimerisation and formation of a receptor signaling complex. See Figure 3-3 for details.
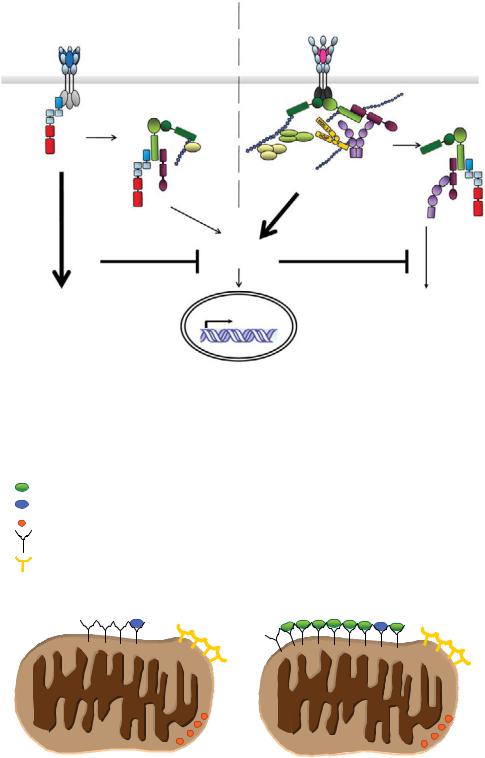
Primarily apoptotic signaling systems |
Primarily immunostimulatory, proinflammatory |
|||
(CD95 and TRAIL systems) |
signaling systems (TNF and DR3 systems) |
|||
Complex I |
|
|
Complex I |
|
FADD |
RIP1 |
TRADD |
|
|
|
|
|||
Complex II |
|
|
TRAF2/5 |
Complex II |
Caspase- 8 |
TAB2 |
TAK1 |
|
|
|
TAB1 |
|
|
|
NEMO |
NEMOIKKβ |
|
cIAP1/2 |
|
|
IKKα |
|
|
|
NF-κB
MAPK
APOPTOSIS |
Gene induction |
APOPTOSIS |
Plate 6. Complex I and complex II: spatial dissociation between proapoptotic and proinflammatory signaling in death receptor signal transduction. See Figure 3-4 for details.
- BIM or BID
- sensitizer BH3-only proteins - cytochrome c
- BCL-2 protein
- BAX/BAK protein
Normal cell |
“Idealized” cancer cell |
Plate 7. Cartoon representation of an unprimed mitochondrion versus a primed mitochondrion. See Figure 5-3 for details.
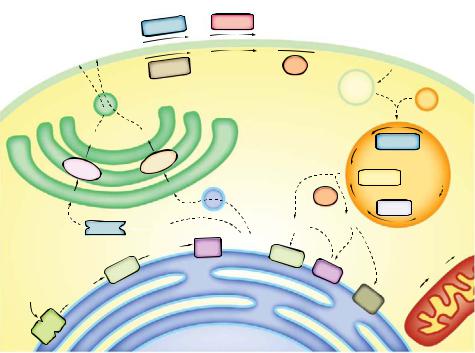
Plasma membrane
|
|
|
|
|
se |
nCDase |
|
|
|
Ma |
|
||
|
|
|
aS |
|
Cer |
Sph |
|
|
SM |
|
|
||
|
L |
|
|
Cer |
Sph |
|
|
|
|
|
|||
M |
GS |
|
|
|
||
S |
|
SM |
|
|
e |
|
|
|
|
|
|
||
|
|
|
|
Mas |
|
|
|
|
|
nS |
|
|
S1P
SK
SM
SM
GSL
Golgi
SM
L S G
GSL
Lysosomes
Cer
aSMase
SM
S |
|
CS |
|
MS |
G |
|
|
|
|
||
|
|
|
|
|
|
|
r |
|
|
e |
|
C |
|
C |
|
er |
|
|
CERT
Serine
+
palmitoyl-CoA
h p S H d
rS e C
|
|
r |
Des |
|
e |
|
|
C |
|
|
|
dH |
|
|
|
ER
Cer
Sph
CerS
SK
SPP
hpS |
aCDase |
GSL |
C |
|
|
|
|
r |
|
|
e |
GCase |
|
|
|
e |
|
|
r |
|
|
C |
|
|
||
|
|
|
lc |
|
|
|
|
S1P |
|
G |
|
|
|
|
|
|
|
|
|
|
ia |
||
|
|
|
|
|
|
||
|
|
|
|
|
|
r |
|
|
|
|
|
|
|
d |
|
|
|
|
|
|
n |
h |
|
|
|
|
|
o |
|
p |
|
|
|
|
h |
|
? |
||
|
|
|
|
S |
|||
|
|
c |
|
|
|
||
|
|
|
|
|
|
||
|
to |
|
|
|
r |
|
|
|
i |
|
|
|
e |
|
|
|
M |
|
|
|
|
||
|
? |
C |
|
|
|||
|
|
|
|
||||
|
|
|
|
|
|
||
S |
|
M |
|
|
|
|
|
P |
S |
|
|
|
|
|
|
L |
|
|
|
|
|
|
S |
P |
T |
|
||
|
|
Nucleus
M A
M s
Plate 8. Compartmentalization of sphingolipid metabolism. See Figure 9-2 for details.
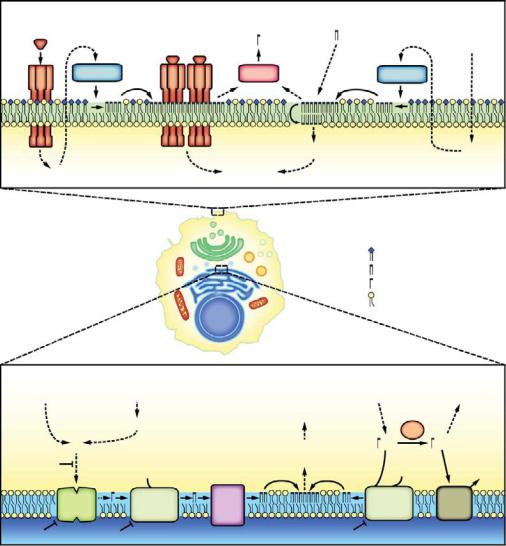
extracellular ligand e.g., CD95L
aSMase
SM Cer
?
(a)
ExogenousCer |
UV, IR, |
Sph |
DNA-damaging |
agents |
|
Receptor clustering |
|
CDase |
aSMase |
|
Cer SM |
flip-flop? |
|
? |
? |
promotion of |
|
apoptosis |
|
Sphingomyelin
Ceramide
Sphingosine
Glycerophospholipid
extracellular ligands |
cellular stresses |
|
salvage |
(e.g., cannabinoids) |
(e.g., DNA damage) |
|
pathway |
|
|
|
promotion of |
|
|
|
apoptosis |
|
p53 |
|
SK |
|
|
|
|
? |
|
|
Sph |
|
|
PP1, PP2A, |
|
|
|
|
|
Bcl-2-like |
|
|
SR proteins, p8, ??? |
proteins |
acyl-CoA |
|
acyl-CoA |
|
|
|
|
|
dhSph |
dhCer |
Cer |
SPT |
CerS |
Des |
CerS |
Myriocin |
FB1 |
|
FB1 |
pro-survival pathways
|
ethano- |
S1P |
lamine |
|
phosphate |
|
+ |
|
hexa- |
|
decenal |
SPL
(b)
Plate 9. Summary of ceramide-mediated pathways. See Figure 9-8 for details.
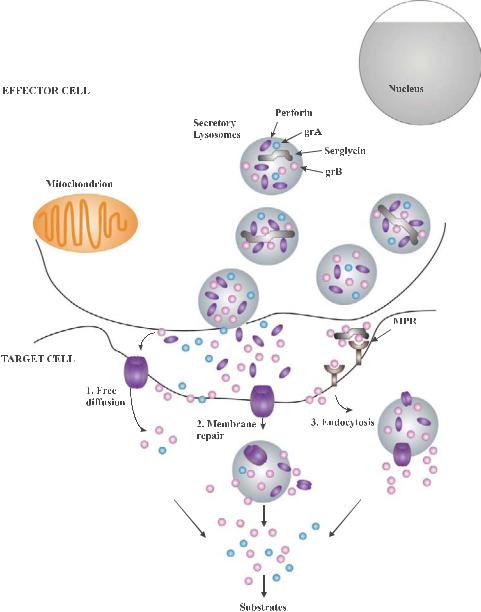
Plate 10. Several hypotheses have been proposed to explain how granzymes enter the target cell to mediate their cell death functions. See Figure 10-1 for details.
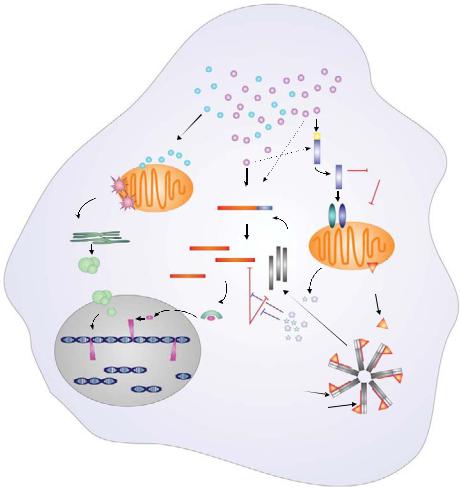
TARGET CELL
grA |
grB |
Human grB
Bid
Mouse grB
Bcl2
tBid
ROS
Procaspase-3
Bax / Bak
ER
Caspase-3
Caspase-9
SET complex
Cytochrome c
CAD |
ICAD |
IAP |
|
|
SMAC/DIABLO
DNAse
Procaspase-9
Apaf-1
APOPTOSOME
Plate 11. GrA and grB show di erent substrate specificities within the target cell. GrA induces the release of ROS from the mitochondrial inner membrane, which mediates the translocation of the SET complex from the ER to the nucleus. See Figure 10-2 for details.

Plate 12. The unfolded protein response (UPR), a coordinated regulated response involving three sensor proteins: PERK (PKR-like ER kinase), ATF6 (activating transcription factor 6), and IRE1 (inositol requiring transmembrane kinase/endoribonuclease). See Figure 12-1 for details.
Plate 13. Proteins implicated in ER stress-pcd pathways. See Figure 12-2 for details.

Isolated intact mito
subcellular |
Purified intact nuclei |
fractionation |
Intact DNA |
Ca2+ overload
supernatant Supernatant containing AIF
~50 kb DNA fragments
pellet
Swollen, permeabilized
mito Condensed, fragmented nuclei
Plate 14. Identification of a caspase-independent mitochondrial apoptosis-inducing factor using an in vitro reconstitution assay with subcellular fractions. See Figure 14-4 for details.
Plate 15. Extrinsic and intrinsic signals of cell death and survival after spinal cord injury. See Figure 15-1 for details.

Plate 16. Retinal neovascularization in age-related macular degeneration (AMD). See Figure 16-2 for details.

Plate 17. The ear. See Figure 17-1 for details.
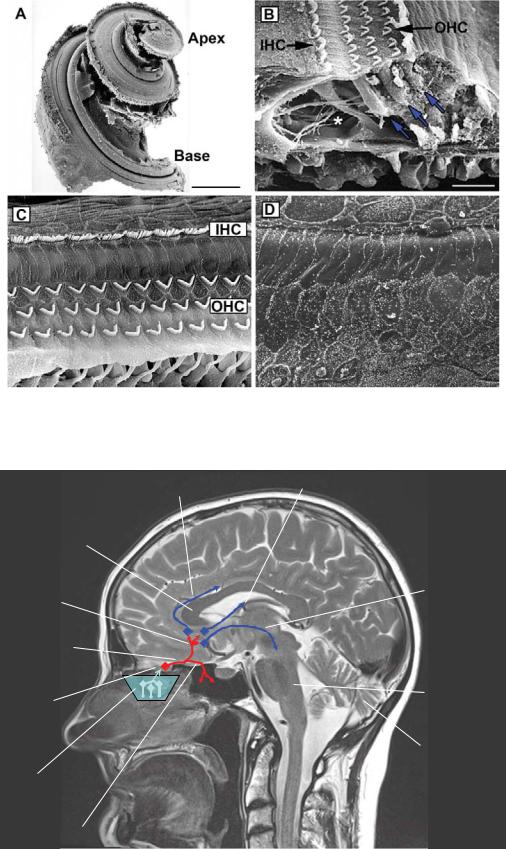
Plate 18. The cochlea. See Figure 17-2 for details.
corpus callosum
medial olfactory stria
olfactory tract
olfactory bulb
olfactory epithelium
lateral olfactory stria
striae medullares
longitudinal striae
medial forebrain bundle
to amygdala |
brainstem |
and prepyriform cortex |
cerebellum
Plate 19. Gross anatomy. See Figure 18-1 for details.
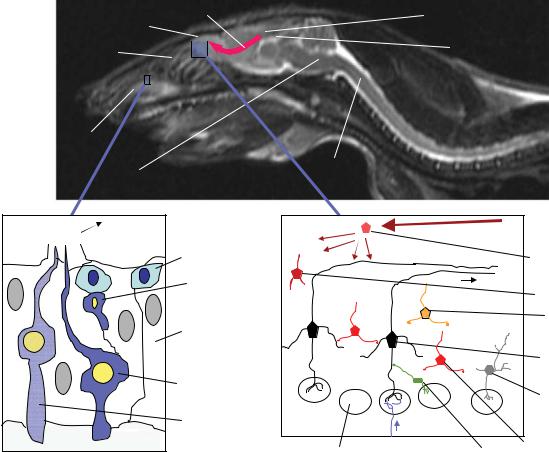
olf. bulb |
RMS |
SVZ |
||
|
|
|
||
|
|
|
|
|
fila |
|
|
|
lateral ventricle |
|
|
|
olfactoria
olf. epithelium
brainstem |
spinal |
|
cord |
|
|
olf. bulb |
RMS |
|
fila olf. |
|
|
basal cell |
|
newborn |
|
granule cell |
|
developing |
olf. tract |
newly integrating |
ORN |
|
granule cell |
supporting |
|
apoptotic |
|
granule cell |
|
cell |
|
|
|
|
|
|
|
mitral cell |
ORN |
|
tufted cell |
|
|
|
apoptotic |
ORN axon |
|
ORN |
|
|
mucus |
|
|
glomerulum |
periglomerular cell mature granule cell |
Plate 20. Olfactory life and death on a microscopic level. See Figure 18-2 for details.
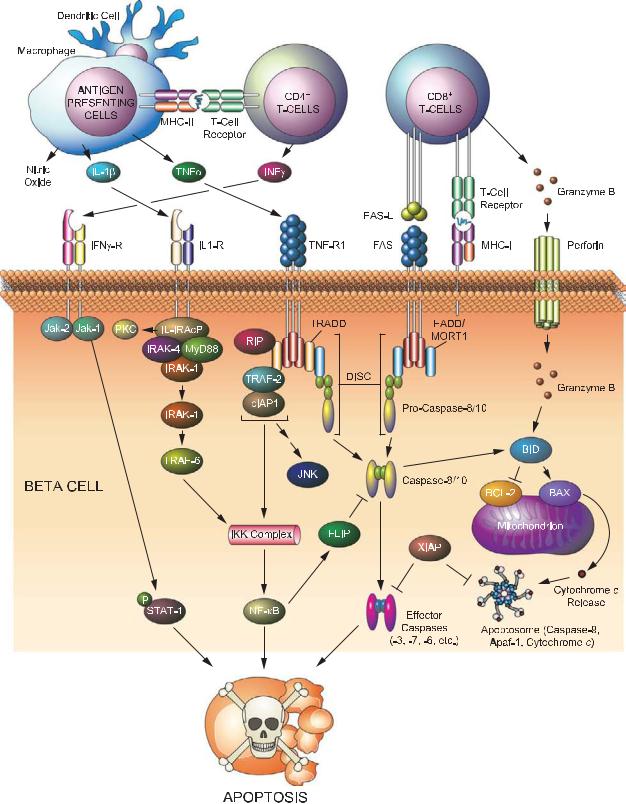
Plate 21. Signaling pathways leading to beta cell destruction in type 1 diabetes. See Figure 19-1 for details.

Plate 22. Shift in lipid partitioning associated with apoptosis in diabetic beta cells. See Figure 19-2 for details.
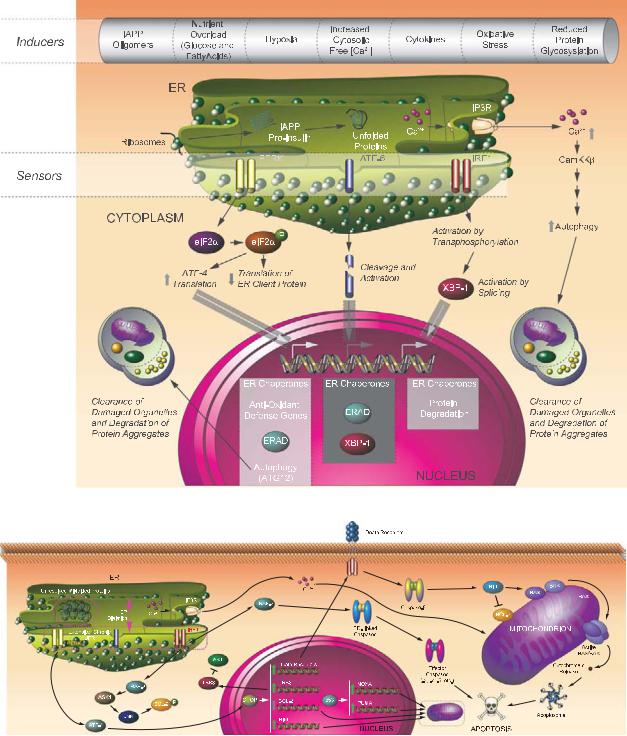
(a)
(b)
Plate 23. Signaling pathways in response to unfolded proteins. See Figure 19-3 for details.

A) Alteration in the BAX/BCL-2 ratio |
C) Activation of caspase-9 |
|
|||
CON |
2d |
5d |
14d |
|
|
M |
M |
M |
M |
|
|
BCL-2 |
|
|
|
|
|
BAX |
|
|
D) Activation of caspase-3 |
|
|
|
|
|
|
||
COX IV |
|
|
|
|
|
B) Release of cytochrome c and DIABLO |
|
|
|||
CON |
2d |
5d |
14d |
|
|
DIABLO |
|
|
TUNEL |
Caspase-3 |
Merged |
|
|
|
|||
|
|
|
E) PARP cleavage |
|
|
Cyt.C |
|
|
|
CON 2d |
5d 14d |
|
|
|
|
89 kDa
Actin
Actin
Plate 24. Hormonal deprivation results in activation of the intrinsic pathway signaling. See Figure 25-3 for details.
A) |
CON 2d |
5d 14d |
p-ATF-2
Total ATF-2
B)
I |
II |
III |
IV |
V |
IV |
TUNEL |
p-p38 MAPK |
Merged |
Plate 25. Activation of p38 MAPK in rat testes after GnRH-A treatment. See Figure 25-4 for details.

Plate 26. Testicular hyperthermia results in serine phosphorylation of BCL-2 in germ cells. See Figure 25-5 for details.
A B C
XII
XII
XII
Plate 27. Activation of ERK in the Sertoli cells. See Figure 25-6 for details.

Tunica adventitia connective tissue
Plate 28. Normal human artery consists of three layers. See Figure 26-1 for details.
Tunica intima
endothelium
internal elastic lamina
external elastic lamina
smooth muscle cells
Tunica media
a
b
Control Apoe-/- |
SM22α-hDTR Apoe-/- |
Control Apoe-/- |
SM22α-hDTR Apoe-/- |
Plate 29. Vascular smooth muscle cell apoptosis accelerates atherosclerotic plaque progression and induces plaque vulnerability. See Figure 26-3 for details.

a |
b |
Percent survival
d
LVEDP (mmHg)
100 |
|
|
|
C360A (n = 19) |
80 |
|
|
WT (n = 197) |
|
60 |
|
|
Line 169 (n = 34) |
|
|
|
|
|
|
40 |
|
|
|
|
20 |
|
|
|
|
0 |
50 100 150 200 250 300 |
|||
0 |
||||
|
|
Time (d) |
|
|
|
|
|
|
|
30 |
|
|
(mmHg/s) |
20000 |
|
|
|
||
|
|
|
15000 |
|
|
|
|
|
|
20 |
|
|
|
10000 |
|
|
|
+dP/dt |
|
10 |
|
|
5000 |
|
|
|
|
||
|
|
|
|
|
0 |
WT |
Tg |
|
0 |
|
|
|
||
n |
7 |
6 |
|
n |
IVS
LV-cavity
PW
– + – + WT Tg
7 |
5 |
6 |
3 |
e |
f |
– |
– |
WT |
Tg |
–dP/dt (mmHg/s)
IVS
LVcavity PW
10000 |
|
|
|
|
7500 |
|
|
|
|
5000 |
|
|
|
|
2500 |
|
|
|
|
0 |
– |
+ |
– |
+ |
|
||||
n |
WT |
|
Tg |
|
7 |
5 |
6 |
3 |
|
|
– |
|
– |
TUNEL-positive myocytes per |
WT |
|
|
Tg |
|
|
c |
4 |
|
|
|
|
(mm) |
3 |
|
|
|
|
|
|
|
|
|
|
|
|
EDD |
2 |
|
|
|
|
|
1 |
|
|
|
|
||
|
|
|
|
|
|
|
|
|
0 |
WT |
Line 7 |
Line 169 |
C360A |
|
|
n |
||||
|
|
9 |
9 |
5 |
3 |
|
|
|
100 |
|
|
|
|
(%) |
75 |
|
|
|
|
|
50 |
|
|
|
|
||
FS |
|
|
|
|
||
25 |
|
|
|
|
||
|
|
|
|
|
|
|
|
|
0 |
WT |
Line 7 |
Line 169 |
C360A |
|
|
n |
||||
|
|
9 |
9 |
5 |
3 |
|
nuclei |
30 |
|
|
|
||
20 |
|
|
|
|
||
cardiac |
|
|
|
|
||
10 |
|
|
|
|
||
|
|
|
|
|
||
5 |
|
|
|
|
|
|
10 |
|
0 |
|
|
|
|
|
|
WT |
Line 7 C360A |
|
||
|
|
|
|
|||
|
|
n |
5 |
6 |
4 |
|
Plate 30. Modest, but elevated, rates of cardiac myocyte apoptosis are su cient over time to induce lethal heart failure. See Figure 26-4 for details.
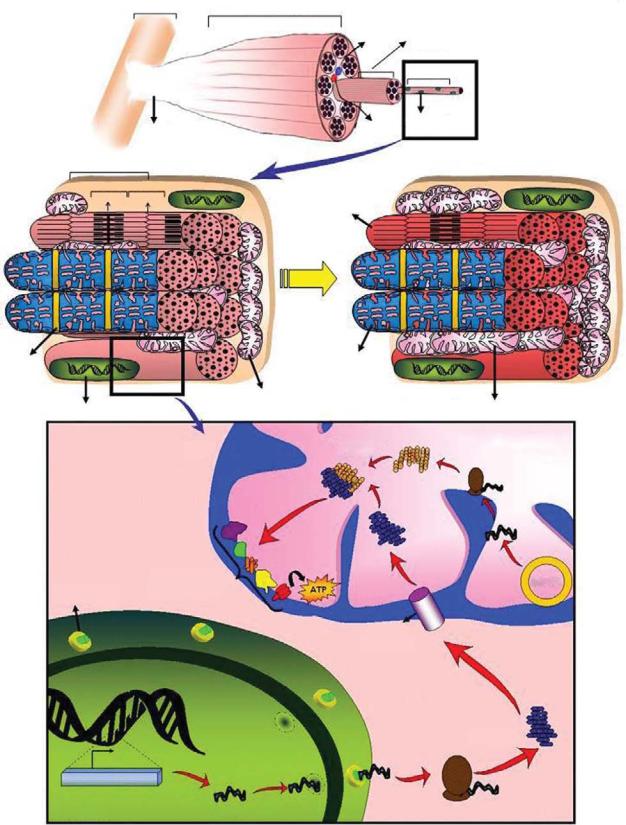
|
Bone |
Skeletal Muscle |
|
|
|
|
|
|
|
Vein |
Muscle |
A |
|
Fascicle |
|
|
|
||
|
|
|
|
|
|
|
Myofiber |
|
Tendon |
Capillary Myonucleus |
|
|
|
|
|
|
Sarcomere |
|
|
|
A-band I-band |
|
|
|
M-line Z-line |
Myofibril |
|
|
|
|
|
|
|
ENDURANCE |
|
|
|
TRAINING |
|
T-tubule |
|
Sarcoplasmic |
|
|
reticulum |
|
|
|
|
|
|
Myonucleus |
Subsarcolemmal |
|
|
(SS) mitochondria |
|
Intermyofibrillar (IMF) mitochondria |
|
B |
OMM |
Holoenzyme |
MITOCHONDRION |
|
assembly |
|
|
|
IMM |
|
|
|
Incorporation |
|
|
|
into ETC |
|
|
Electron transport |
mtDNA |
|
chain (ETC) |
||
ATP |
||
|
Nuclear pore
Import machinery
Translation
+1 |
Transcription |
NUGEMPS |
|
NUCLEUS |
mRNA |
Plate 31. Unique morphology of skeletal muscle and exercise-induced mitochondrial biogenesis. See Figure 27-1 for details.
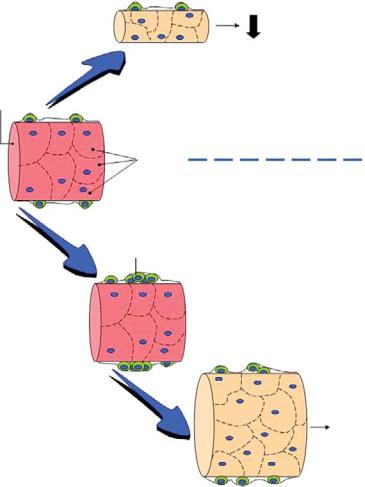
Loss of Myonuclei +
Satellite cells
Myonuclear
domain
Myofiber
Myonuclear
Domains
Satellite cell activation, proliferation
Atrophy
Hypertrophy
Fusion of satellite cells, myonuclear addition and resultant fiber hypertrophy
Myonuclear Domain is constant
Plate 32. Myonuclear domains during muscle hypertrophy and atrophy. See Figure 27-2 for details.

Plate 33. UVB signaling in keratinocytes. UVB can lead to di erent e ects in keratinocytes, ranging from cell cycle arrest, apoptosis, and inflammasome activation. UVB radiation primarily damages nuclear DNA as a result of direct absorption and generates ROS that can induce oxidative damage to DNA and cellular proteins. See Figure 28-3 for details.
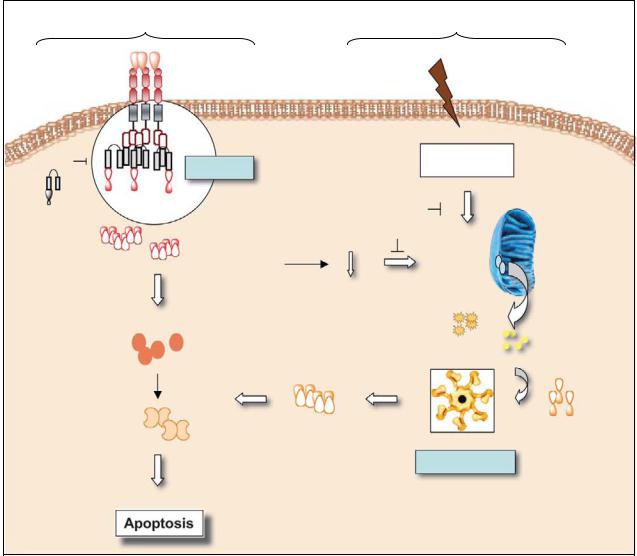
Extrinsic pathway
Ligand
|
Death |
|
receptors |
|
FADD |
FLIP |
DISC |
|
|
|
Procaspases |
|
-8 and -10 |
|
Active caspases-8 |
|
and -10 |
Pro-caspases-3, -6, -7
Intrinsic pathway
Cytokine withdrawal, chemotherapeutic drugs, radiations…
BH3-only
proteins
Anti-apoptotic Bcl-2 family members
Bid
Bax/Bak
tBid
Cytochrome C |
Apaf-1 |
Active effector |
|
|
caspases-3, -6 and -7 |
Active caspase-9 |
Pro-caspase-9 |
|
Apoptosome
Plate 34. Two signaling pathways leading to apoptosis. See Figure 29-1 for details.

Plate 35. Lymphocyte apoptosis in spleen of septic patient. Hematoxylin and eosin staining of spleen of septic patient (400 × magnification). See Figure 31-1 for details.
Plate 36. Colonic epithelial apoptosis in a septic patient. See Figure 31-2 for details.
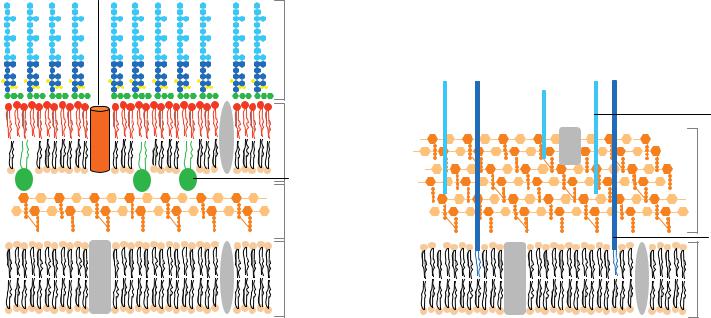
Gram-negative bacteria
Porin
Gram-positive bacteria
Lipopolysaccharide
Outer membrane
lipoprotein
Peptidoglycan
Plasma membrane
Plate 37. A representation of the cell wall from Gram-negative and Gram-positive bacteria. See Figure 32-2 for details.
teichoic acid
Peptidoglycan
Lipoteichoic acid
Plasma membrane

Mammalian |
Insect |
|
TNF TNF |
TNF |
|
|
|
|
|
|
|
|
|
|
|
TNFRTNFRTNFR |
|
|
|
|
|
|
|
|
|
||
DD |
DD |
DD |
DD |
DD |
|
|
|
|
|
|
|
|
|
|
|
|
|
|
|
|
|
|
|
||
DD |
|
|
|
DD |
|
|
|
Complex II |
|
|||
Complex I |
|
|
|
|
DISC |
|
|
|||||
TRADD |
|
TRADD |
|
|
|
|
|
|
|
|||
RIP1 |
|
RIP1 |
DED |
DED |
DD |
DD |
DD |
TRADD RIP1 |
TRAF2 |
RING |
||
|
|
|
||||||||||
|
|
|
|
DED |
|
|
|
|
|
|
||
|
TRAF2 |
TRAF2 |
|
|
|
|
|
|
|
|
||
|
RING |
|
RING |
|
p20 |
|
|
|
|
|
|
|
|
|
|
|
|
DED |
FADD |
DD |
DD |
TRADD RIP1 |
TRAF2 |
RING |
|
|
|
|
|
|
DEDDED |
DD |
||||||
BIR BIR BIRCARD RING |
p10 |
|
|
|
|
|
|
|
||||
|
cIAP1/2 |
|
|
|
|
|
|
|
|
|
||
|
TAB2 |
|
TAK1 |
|
|
|
|
|
|
|
|
|
|
|
|
|
p20 |
|
|
|
|
|
|
|
|
|
|
|
|
|
Pro-caspase-8/10 |
|
|
|
||||
|
IKK |
IKK |
|
p10 |
|
|
|
|
|
|
|
|
|
|
|
|
|
|
|
|
|
|
|||
|
NEMO |
|
|
|
|
|
|
|
|
|
||
|
|
|
|
|
Caspase-3/6/7 |
|
|
|
|
|
||
|
I B |
|
|
|
p10p10 |
|
|
|
|
|
|
|
|
|
|
|
p20 |
|
p20 |
|
|
|
|
|
|
|
|
|
|
|
|
|
|
|
|
|
||
|
p50 |
|
RelA |
|
|
|
|
|
|
|
|
|
Substrate processing
Apoptosis
DAP-type
Peptidoglycan
derivatives
PGRP
-LC
IMD
DD
BIR BIR BIRCARD RING
DIAP2
TAB2
TAK1
IKK Ird5
Kenny
Rel
Gram-negative
bacteria
dFADD
|
DED |
DD |
DED |
DED |
|
p20
Dredd
p10
Relish
ANK
Rel
|
NF B |
|
|
NF B |
p50 |
RelA |
|
Rel |
Rel |
Induction of survival and |
DNA fragmentation |
Induction of Diptericin and other |
||
inflammatory genes |
Chromatin condensation |
antimicrobial genes |
Plate 38. Schematic representation of the mammalian TNF and insect Imd pathways. See Figure 32-6 for details.
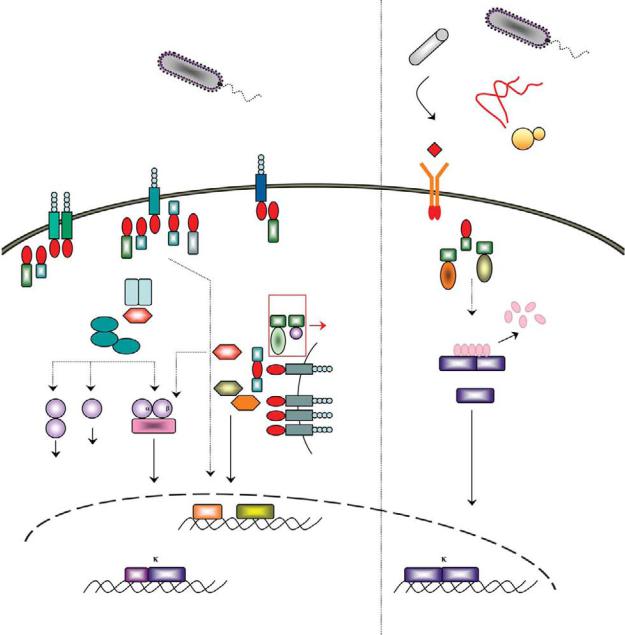
Mammalian
Gram-positive and Gram-negative
bacteria
Insect
|
|
Gram-positive |
|
|
bacteria |
- |
Lysine-type |
|
PGRP |
S |
|
|
Peptidoglycan |
derivatives
|
|
|
|
|
|
|
|
|
Flagellin |
|
|
|
|
|
|
|
|
LPS |
|
|
|
|
|
|
|
|
Bacterial |
TIR |
TIR |
TLR4TIR |
TIR |
TIR |
TIRTLR5 |
TIR |
|
|
||
|
|
TLR1/6 |
TLR2 |
|
|
|||||||
|
lipoproteins |
|
|
|
|
|
|
|
|
|
||
TIR |
TIR |
TIR TIR |
|
Mal |
TRIF |
|
MyD88 |
|
|
|||
|
|
|
|
|
|
|
|
|
|
|
||
|
|
|
MyD88 |
|
|
TRAM |
|
|
|
|
||
|
|
|
|
|
|
|
|
|
|
|||
MyD88 |
|
|
IRAK1 |
IRAK4 |
|
|
|
|
|
|
|
|
|
Mal |
|
|
|
|
|
|
|
|
|
|
|
|
|
|
|
TRAF6 |
|
|
|
|
FADD |
|
||
|
|
|
|
|
|
|
|
DD |
DD |
|
||
|
|
|
TAB2 |
|
|
|
|
|
|
Apoptosis |
||
|
|
|
|
|
|
|
|
|
|
DED |
||
|
|
|
TAK1 |
|
|
|
|
|
TRIF RIP1 |
|
||
|
|
|
|
|
|
|
|
|
|
|||
|
|
|
|
TAB1 |
|
|
|
|
|
|
||
|
|
|
|
|
|
|
TRAF6 |
|
|
|
|
|
|
|
|
|
|
|
|
|
|
|
|
|
|
|
|
|
|
|
|
|
|
|
TIR |
TIR |
TLR3 |
|
|
|
|
|
|
|
|
|
TRAF3 |
|
|
|
Nucleic |
|
|
MKK3 |
MKK7 |
|
IKK |
IKK |
|
TBK1 |
TIR |
TLR7 |
acids |
|
|
|
|
|
|
|
TIR |
TLR8 |
|
||||
|
|
|
|
|
|
|
|
|
|
|
||
|
|
MKK6 |
|
|
NEMO |
|
|
|
TIR |
TLR9 |
|
|
|
|
|
|
|
|
|
|
|
|
|||
|
|
p38 |
JNK |
|
|
|
|
|
|
|
Endosome |
|
|
|
|
|
|
|
|
|
|
|
|
|
|
|
|
|
|
|
|
|
|
IRF3 |
IRF7 |
|
|
|
Fungi
Spätzle
Yeast
Toll
TIR |
|
|
|
|
dMyD88 |
TIR |
|
|
|
DD |
|
|
||
|
|
DD |
|
|
|
|
|
|
|
|
DD |
|
|
Pelle |
Tube |
|
|
|
|
|
|
|
|
|
|
Cactus |
|
||
|
|
ANK |
|
|
Dif |
Rel |
Rel |
Dorsal |
Rel
|
Induction of the interferon |
|
response |
NF B |
NF B |
p50 RelA
Induction of survival and inflammatory genes
Rel Rel
Induction of Drosomycin and other antimicrobial genes
Plate 39. A schematic representation of the mammalian TLR and insect Toll pathways. See Figure 32-7 for details.

NOD1 |
|
|
CARD |
NBD |
|
NOD2 |
|
CARD CARD |
NBD |
|
|
NOD3/9/27 |
|
|
X |
NBD |
|
(NLRC3/X1/5) |
|
|
|
||
|
|
|
|
|
|
|
|
|
|
|
|
NALP1 |
|
|
PYD |
NBD |
FIIND CARD |
(NLRP1) |
|
|
|||
|
|
|
|
|
|
NALP2-14 |
|
|
PYD |
NBD |
|
(NLRP2-14) |
|
|
|
||
|
|
|
|
|
|
|
|
|
|
|
|
IPAF |
|
|
CARD |
NBD |
|
(NLRC4) |
|
|
|
||
|
|
|
|
|
|
NAIP |
BIR |
BIR BIR |
CARD |
NBD |
|
(NLRB) |
|
||||
|
|
|
|
|
|
|
|
|
|
|
|
CIITA |
|
|
AD |
NBD |
|
|
|
|
|
|
|
APAF1 |
|
|
CARD |
NBD |
|
Ced4 |
|
|
CARD |
NBD |
|
|
|
|
|
|
|
|
|
|
|
|
|
ASC |
|
PYD |
CARD |
|
|
CARDINAL |
|
FIIND |
CARD |
|
|
|
|
|
|
|
|
|
|
|
|
|
|
Caspase- |
|
|
|
p20 |
p10 |
3/6/7 |
|
|
|
|
|
Caspase-8/10 |
|
DED DED |
p20 |
p10 |
|
|
|
|
|
|
|
cIAP1/2 |
BIR |
BIR |
BIR |
CARD |
RING |
|
|
|
|
XIAP |
BIR |
BIR |
BIR |
|
RING |
|
|
|
|
TRAF2 |
|
RING |
|
TZ |
TZ |
|
CC |
MATH |
|
TRAF6 |
|
RING |
|
TZ |
TZ |
|
CC |
MATH |
|
RIP1 |
|
|
|
Kinase domain |
|
DD |
|
||
RIP2 |
|
|
|
Kinase domain |
|
CARD |
|
||
IKK / |
|
|
|
Kinase domain |
LZ |
HLH |
Nemo BD |
||
IKK |
|
|
|
|
CC1 |
|
CC2 |
LZ |
ZF |
FADD |
|
|
|
|
|
|
DED |
DD |
|
TRADD |
|
|
|
|
|
|
|
DD |
|
TNFR1 |
|
EC |
|
EC |
EC |
EC |
TM |
DD |
|
Caspase-1 |
|
|
|
CARD |
|
p20 |
p12 |
|
|
Caspase-9 |
|
|
|
CARD |
|
p20 |
p10 |
|
|
Ced3 |
|
|
|
CARD |
|
p20 |
p10 |
|
Plate 40. E ectors of inflammation. The domain structures are shown. See Figure 32-8 for details.
Cytochrome C PAMPs
Ced9 |
Ced4 |
Apaf1 |
NLRs |
Bcl-2, |
|
|
|
|
Bcl-xl |
|
|
|
? |
|
|
? |
Apoptosome |
|
Inflammasome |
|
|
|
Active |
Active |
Active |
Ced3 |
Caspase-9 |
Caspase-1 |
APOPTOSIS |
APOPTOSIS |
PYROPTOSIS & |
|
|
INFLAMMATION |
Plate 41. A parallel between mitochondrial apoptosis and NLR innate immunity pathways. See Figure 32-9 for details.
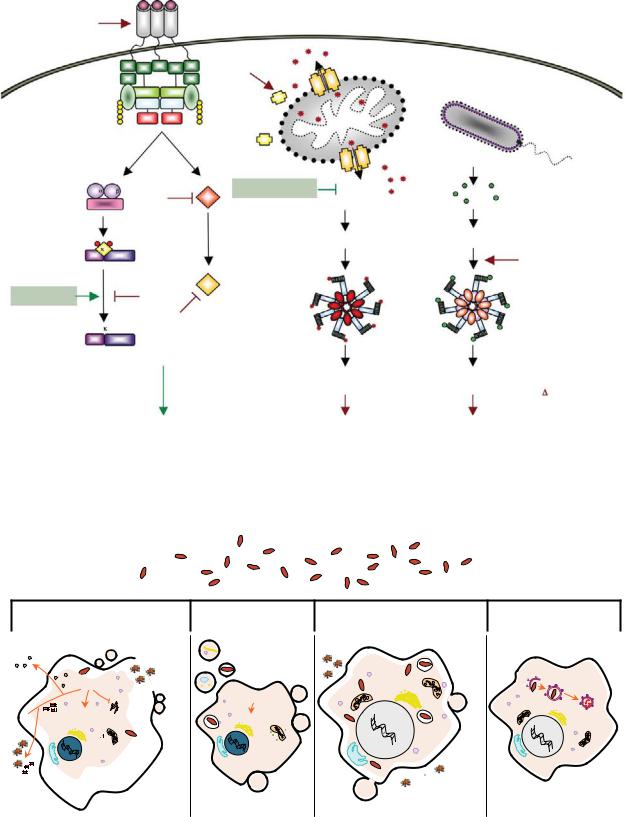
|
FasL FasL FasL |
|
||
H. pylori |
Fas Fas Fas |
|
||
|
|
|||
P. aeruginosa |
|
|
|
|
DD |
DD |
DD |
DD |
DD |
|
|
|
||
DD |
|
|
|
DD |
|
TRADD |
|
TRADD |
|
RIP1 |
|
|
RIP1 |
|
Ub |
TRAF2 TRAF2 |
Ub |
||
b |
|
|
|
Ub |
U |
|
|
|
|
b |
|
|
|
Ub |
U |
|
|
|
|
b |
RING |
|
RING |
Ub |
U |
|
|||
b |
|
Ub |
||
|
|
|
||
U |
|
|
|
S. pneumoniae
BH3
BH3
IKK |
IKK |
B. Anthracis |
Chlamydia spp. |
Cytochrome c |
PAMPs |
Nigericin |
|
|
|
|
|
|
|
|
|
(LT) |
MKK |
|
|
Maitotoxin |
NEMO |
|
|
|
|||
|
|
|
|
|
|
Aerolysin |
|
|
|
|
|
|
F. tularensis |
P |
P |
|
|
Apaf1 |
NLRs |
S. flexneri |
I B |
|
|
|
|
||
p50 |
RelA |
|
|
|
|
|
|
|
|
Apoptosome |
Inflammasome |
B. Anthracis |
|
|
|
S. typhimurium |
MAPK |
|
|
(LT) |
R. rickettsii |
|
(AvrA) |
|
|
|
L. monocytogenes |
|
Y. pestis |
|
|
|
||
|
|
|
|
|
||
|
|
|
|
|
(LLO) |
|
|
|
(YopJ) |
|
|
|
|
|
|
|
|
|
|
|
NF B |
|
|
|
|
S. typhimurium |
|
p50 |
RelA |
|
|
|
(TTSS) |
|
|
|
|
|
|
|
L. pneumophila |
|
|
|
|
Active |
Active |
P. aeruginosa |
|
|
|
|
Caspase-9 |
Caspase-1 |
|
|
|
|
|
( Exo U) |
||
|
|
|
|
|
|
|
|
|
SURVIVAL |
|
APOPTOSIS |
PYROPTOSIS & |
|
|
|
|
|
|
INFLAMMATION |
|
Plate 42. Modulation of cell survival/death pathways by microbial e ectors. See Figure 32-10 for details.
Pyroptosis |
|
Apoptosis |
Oncosis |
|
Autophagy |
||
Unconventional |
|
|
|
Apoptotic |
|
|
|
protein secretion |
|
|
Membrane |
|
|
|
|
|
|
bodies |
|
|
|
||
|
|
|
rupture |
|
|
|
|
|
|
|
|
|
|
|
|
|
|
|
|
Membrane |
|
Membrane |
Atg7 |
|
|
|
|
blebbing |
|
blebbing |
|
|
Caspase-1 |
|
Organelle |
Atg8/LC3 |
|||
|
|
|
|
||||
|
|
|
|
Caspase-3, 7 |
swelling |
|
Atg8/LC3 |
|
|
|
|
|
|
Autophagosome |
|
|
|
|
|
|
|
|
|
Immature |
Other |
Glycolysis |
|
Cyto C |
|
|
Autophago- |
|
Substrates release |
|
|
||||
Membrane |
|
|
lysosome |
||||
cytokines |
substrates |
enzymes |
|
|
|||
|
|
|
vesicles |
|
|
Nuclear |
|
|
|
m |
|
|
|
swelling |
|
|
Nuclear |
|
Nuclear |
|
|
|
|
|
|
condensation |
|
|
|
||
|
condensation |
|
|
|
|
||
|
|
DNA |
|
|
|
||
|
|
|
|
|
|
|
|
DNA |
|
|
fragmentation |
|
|
|
|
fragmentation |
|
|
Cell |
|
Membrane |
|
|
Mature |
|
|
Membrane |
|
|
||
|
|
shrinkage |
|
permeability |
|
||
cytokines |
|
|
swelling |
|
|
|
|
Plate 43. Pathogen-induced host cell death. See Figure 32-11 for details.
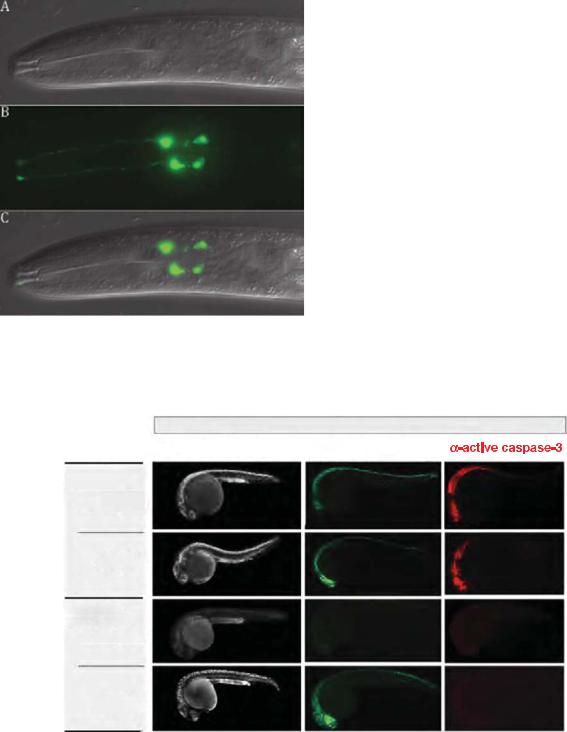
Plate 44. The four male-specific chemosensory (CEM) neurons located in the cephalic region of the animal undergo programmed cell death in hermaphrodites. See Figure 34-3 for details.
12.5 Gy
acridine orange |
TUNEL |
p53 +/+
non-injected
chk1 MO
p53e7/e7
non-injected
chk1 MO
Plate 45. A rapid morpholino loss-of-function screen identifies chk1 knockdown as a caspase-3–independent radiation sensitizer in p53 mutant embryos. See Figure 36-3 for details.
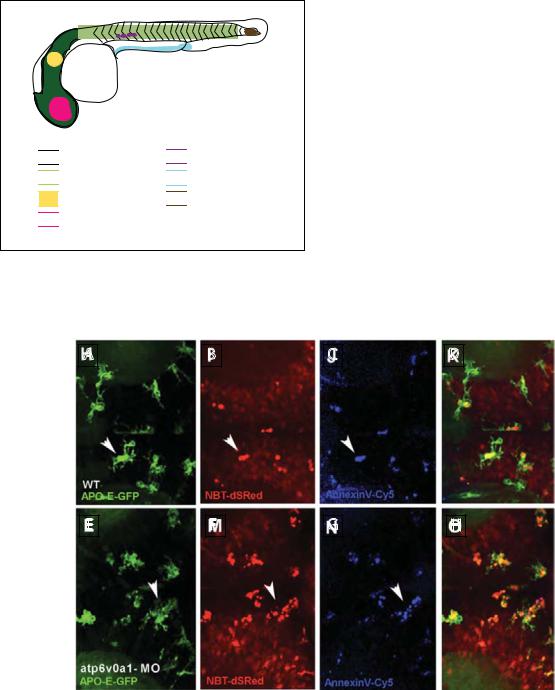
Regions of developmental cell death
Brain
Spinal cord
Ear
Eye
Plate 46. Cell death zones in developing zebrafish embryos. See Figure 36-5 for details.
Germ cells
Excretory system
Tail bud
A |
|
B |
|
C |
|
D |
E |
|
F |
|
G |
|
H |
Plate 47. Images of microglia consuming dying neurons by phagocytosis in wild-type and atpv0a1 morphant larvae. See Figure 36-6 for details.
APOPTOSIS IN THE PHYSIOLOGY AND DISEASES OF THE RESPIRATORY TRACT |
227 |
after tracheal occlusion in the fetal rabbit. Am J Pathol. 152, 179–90.
De Paepe, M.E., Mao, Q., Chao, Y., Powell, J.L., Rubin, L.P., and Sharma, S. (2005). Hyperoxia-induced apoptosis and Fas/FasL expression in lung epithelial cells. Am J Physiol Lung Cell Mol Physiol. 289, L647–59.
De Paepe, M.E., Sardesai, M.P., Johnson, B.D., Lesieur-Brooks, A.M., Papadakis, K., and Luks, F.I. (1999b). The role of apoptosis in normal and accelerated lung development in fetal rabbits. J Pediatr Surg. 34, 863–70.
Del Riccio, V., van Tuyl, M., and Post, M. (2004). Apoptosis in lung development and neonatal lung injury. Pediatr Res. 55, 183–9.
Droemann, D., Aries, S.P., Hansen, F., Moellers, M., Braun, J., Katus, H.A., and Dalhoff, K. (2000). Decreased apoptosis and increased activation of alveolar neutrophils in bacterial pneumonia. Chest. 117, 1679–84.
Fadok, V.A., Bratton, D.L., Konowal, A., Freed, P.W., Westcott, J.Y., and Henson, P.M. (1998). Macrophages that have ingested apoptotic cells in vitro inhibit proinflammatory cytokine production through autocrine/paracrine mechanisms involving TGF-beta, PGE2, and PAF. J Clin Invest. 101, 890–8.
Fedorov, L.M., Tyrsin, O.Y., Papadopoulos, T., Camarero, G., Gotz,¨ R., and Rapp, U.R. (2002). Bcl-2 determines susceptibility to induction of lung cancer by oncogenic C-Raf. Cancer Res. 62, 6297–303.
Fujita, M., Kuwano, K., Kunitake, R., Hagimoto, N., Miyazaki, H., Kaneko, Y., Kawasaki, M., Maeyama, T., and Hara, N. (1998). Endothelial cell apoptosis in lipopolysaccharideinduced lung injury in mice. Int Arch Allergy Immunol. 117, 202–8.
Galie, N., Hoeper, M.M., Humbert, M., Torbicki, A., Vachiery, J-L., Barbera, J.A., Beghetti, M., Corris, P., Gaine, S., Gibbs, J.S., Gomez-Sanchez, M.A., Jondeau, G., Klepetko, W., Opitz, C., Peacock, A., Rubin, L., Zellweger, M., and Simonneau, G. (2009). Guidelines for the diagnosis and treatment of pulmonary hypertension. Eur Respir J; 34: 1219–1263.
Greco, F.A., Bonomi, P., Crawford, J., Kelly, K., Oh, Y., Halpern, W., Lo, L., Gallant, G., and Klein, J. (2008). Phase 2 study of mapatumumab, a fully human agonistic monoclonal antibody which targets and activates the TRAIL receptor-1, in patients with advanced non-small cell lung cancer. Lung Cancer. 61, 82–90.
Hagimoto, N., Kuwano, K., Miyazaki, H., Kunitake, R., Fujita, M., Kawasaki, M., Kaneko, Y., and Hara, N. (1997). Induction of apoptosis and pulmonary fibrosis in mice in response to ligation of Fas antigen. Am J Respir Cell Mol Biol. 17, 272–8.
Hargitai, B., Szabo, V., Hajdu, J., Harmath, A., Pataki, M., Farid, P., Papp, Z., and Szende, B. (2001). Apoptosis in various organs of preterm infants: histopathologic study of lung, kidney, liver, and brain of ventilated infants. Pediatr Res. 50, 110– 14.
Hodge, S., Hodge, G., Ahern, J., Jersmann, H., Holmes, M., and Reynolds, P.N. (2007). Smoking alters alveolar macrophage recognition and phagocytic ability: implications in chronic
obstructive pulmonary disease. Am J Respir Cell Mol Biol. 37, 748–55.
Hodge, S., Hodge, G., Holmes, M., and Reynolds, P.N. (2005). Increased airway epithelial and T-cell apoptosis in COPD remains despite smoking cessation. Eur Respir J. 25, 447–54.
Hodge, S., Hodge, G., Nairn, J., Holmes, M., and Reynolds, P.N. (2006). Increased airway granzyme B and perforin in current and ex-smoking COPD subjects. COPD. 3, 179–87.
Hoffarth, S., Zitzer, A., Wiewrodt, R., Hahnel,¨ P.S., Beyer, V., Kreft, A., Biesterfeld, S., and Schuler, M. (2008). pp32/PHAPI determines the apoptosis response of non-small-cell lung cancer. Cell Death Differ. 15, 170.
Holopainen, R., Aho, H., Laine, J., Peuravuori, H., Soukka, H., and Kaapa, P. (1999). Human meconium has high phospholipase A2 activity and induces cellular injury and apoptosis in piglet lungs. Pediatr Res. 46, 626–32.
Huynh, M.L., Fadok, V.A., and Henson, P.M. (2002). Phos- phatidylserine-dependent ingestion of apoptotic cells promotes TGF-beta1 secretion and the resolution of inflammation. J Clin Invest. 109, 41–50.
Imai, K., Mercer, B.A., Schulman, L.L., Sonett, J.R., and D’Armiento, J.M. (2005). Correlation of lung surface area to apoptosis and proliferation in human emphysema. Eur Respir J. 25, 250–8.
Jiang, X., Kim, H.-E., Shu, H., Zhao, Y., Zhang, H., Kofron, J., Donnelly, J., Burns, D., Ng, S.-C., Rosenberg, S., and Wang, X. (2003). Distinctive roles of PHAP proteins and prothymosin-a in a death regulatory pathway. Science. 299, 223–6.
Jin, H., Yang, R., Fong, S., Totpal, K., Lawrence, D., Zheng, Z., Ross, J., Koeppen, H., Schwall, R., and Ashkenazi, A. (2004). Apo2 Ligand/tumor necrosis factor-related apoptosisinducing ligand cooperates with chemotherapy to inhibit orthopic lung tumor growth and improve survival. Cancer Res. 64, 4900–5.
Johnson, L., Mercer, K., Greenbaum, D., Bronson, R.T., Crowley, D., Tuveson, D.A., and Jacks, T. (2001). Somatic activation of the K-ras oncogene causes early onset lung cancer in mice.
Nature. 410, 1111–16.
Johnstone, R.W., Ruefli, A.A., and Lowe, S.W. (2002). Apoptosis: a link between cancer genetics and chemotherapy. Cell. 108, 153–64.
Karrasch, S., Holz, O., and Jorres, R.A. (2008). Aging and induced senescence as factors in the pathogenesis of lung emphysema. Respir Med. 102, 1215–30.
Kasahara, Y., Tuder, R.M., Cool, C.D., Lynch, D.A., Flores, S.C., and Voelkel, N.F. (2001a). Endothelial cell death and decreased expression of vascular endothelial growth factor and vascular endothelial growth factor receptor 2 in emphysema. Am J Respir Crit Care Med. 163, 737–44.
Kasahara, Y., Tuder, R.M., Cool, C.D., Lynch, D.A., Flores, S.C., and Voelkel, N.F. (2001b). Endothelial cell death and decreased expression of vascular endothelial growth factor and vascular endothelial growth factor receptor 2 in emphysema. Am J Respir Crit Care Med. 163, 737–44.
Kasahara, Y., Tuder, R.M., Taraseviciene-Stewart, L., Le Cras, T.D., Abman, S., Hirth, P.K., Waltenberger, J., and Voelkel, N.F.
228 |
CHRISTIAN TAUBE AND MARTIN SCHULER |
(2000). Inhibition of VEGF receptors causes lung cell apoptosis and emphysema. J Clin Invest. 106, 1311–19.
Kawasaki, M., Kuwano, K., Hagimoto, N., Matsuba, T., Kunitake, R., Tanaka, T., Maeyama, T., and Hara, N. (2000). Protection from lethal apoptosis in lipopolysaccharide-induced acute lung injury in mice by a caspase inhibitor. Am J Pathol. 157, 597–603.
Kitamura, Y., Hashimoto, S., Mizuta, N., Kobayashi, A., Kooguchi, K., Fujiwara, I., and Nakajima, H. (2001). Fas/ FasL-dependent apoptosis of alveolar cells after lipo- polysaccharide-induced lung injury in mice. Am J Respir Crit Care Med. 163, 762–9.
Kresch, M.J., Christian, C., Wu, F., and Hussain, N. (1998). Ontogeny of apoptosis during lung development. Pediatr Res. 43, 426–31.
Kuwano, K., Hagimoto, N., Kawasaki, M., Yatomi, T., Nakamura, N., Nagata, S., Suda, T., Kunitake, R., Maeyama, T., Miyazaki, H., and Hara, N. (1999). Essential roles of the Fas-Fas ligand pathway in the development of pulmonary fibrosis. J Clin Invest. 104, 13–19.
Kuwano, K., Kunitake, R., Kawasaki, M., Nomoto, Y., Hagimoto, N., Nakanishi, Y., and Hara, N. (1996). P21Waf1/Cip1/Sdi1 and p53 expression in association with DNA strand breaks in idiopathic pulmonary fibrosis. Am J Respir Crit Care Med. 154, 477–83.
Kuwano, K., Kunitake, R., Maeyama, T., Hagimoto, N., Kawasaki, M., Matsuba, T., Yoshimi, M., Inoshima, I., Yoshida, K., and Hara, N. (2001). Attenuation of bleomycin-induced pneumopathy in mice by a caspase inhibitor. Am J Physiol Lung Cell Mol Physiol. 280, L316–25.
Lane, K.B., Machado, R.D., Pauciulo, M.W., Thomson, J.R., Phillips, J.A., III, Loyd, J.E., Nichols, W.C., and Trembath, R.C. (2000). Heterozygous germline mutations in BMPR2, encoding a TGF-beta receptor, cause familial primary pulmonary hypertension. The International PPH Consortium. Nat Genet. 26, 81–4.
Lee, C.G., Cho, S.J., Kang, M.J., Chapoval, S.P., Lee, P.J., Noble, P.W., Yehualaeshet, T., Lu, B., Flavell, R.A., Milbrandt, J., Homer, R.J., and Elias, J.A. (2004). Early growth response gene 1-mediated apoptosis is essential for transforming growth factor beta1-induced pulmonary fibrosis. J Exp Med. 200, 377–89.
Levesque, B.M., Vosatka, R.J., and Nielsen, H.C. (2000). Dihydrotestosterone stimulates branching morphogenesis, cell proliferation, and programmed cell death in mouse embryonic lung explants. Pediatr Res. 47, 481–91.
Lu, Q., Xu, D.Z., Davidson, M.T., Hasko, G., and Deitch, E.A. (2004). Hemorrhagic shock induces endothelial cell apoptosis, which is mediated by factors contained in mesenteric lymph. Crit Care Med. 32, 2464–70.
Lukkarinen, H.P., Laine, J., and Kaapa, P.O. (2003). Lung epithelial cells undergo apoptosis in neonatal respiratory distress syndrome. Pediatr Res. 53, 254–9.
Mantell, L.L., Horowitz, S., Davis, J.M., and Kazzaz, J.A. (1999). Hyperoxia-induced cell death in the lung–the correlation of
apoptosis, necrosis, and inflammation. Ann N Y Acad Sci. 887, 171–80.
Martin, T.R., Hagimoto, N., Nakamura, M., and Matute-Bello, G. (2005). Apoptosis and epithelial injury in the lungs. Proc Am Thorac Soc. 2, 214–20.
Matute-Bello, G., Liles, W.C., Frevert, C.W., Nakamura, M., Ballman, K., Vathanaprida, C., Kiener, P.A., and Martin, T.R. (2001). Recombinant human Fas ligand induces alveolar epithelial cell apoptosis and lung injury in rabbits. Am J Physiol Lung Cell Mol Physiol. 281, L328–35.
Matute-Bello, G., Liles, W.C., Radella, F., Steinberg, K.P., Ruzinski, J.T., Jonas, M., Chi, E.Y., Hudson, L.D., and Martin, T.R. (1997). Neutrophil apoptosis in the acute respiratory distress syndrome. Am J Respir Crit Care Med. 156, 1969–77.
Matute-Bello, G., Liles, W.C., Steinberg, K.P., Kiener, P.A., Mongovin, S., Chi, E.Y., Jonas, M., and Martin, T.R. (1999). Soluble Fas ligand induces epithelial cell apoptosis in humans with acute lung injury (ARDS). J Immunol. 163, 2217–25.
May, M., Strobel, P., Preisshofen, T., Seidenspinner, S., Marx, A., and Speer, C.P. (2004). Apoptosis and proliferation in lungs of ventilated and oxygen-treated preterm infants. Eur Respir J. 23, 113–21.
McDonnell, T.J., Fang, B., Yu, R., Kagawa, S., Hunt, K.K., McDonnell, T.J., Roth, J.A., and Swisher, S.G. (2000). Adenoviral Bak overexpression mediates caspase-dependent tumor killing.
Cancer Res. 60, 788–92.
McMurtry, M.S., Archer, S.L., Altieri, D.C., Bonnet, S., Haromy, A., Harry, G., Bonnet, S., Puttagunta, L., and Michelakis, E.D. (2005). Gene therapy targeting survivin selectively induces pulmonary vascular apoptosis and reverses pulmonary arterial hypertension. J Clin Invest. 115, 1479–91.
Metzger, R.J., Klein, O.D., Martin, G.R., and Krasnow, M.A. (2008). The branching programme of mouse lung development. Nature. 453, 745–50.
Morrell, N.W., Yang, X., Upton, P.D., Jourdan, K.B., Morgan, N., Sheares, K.K., and Trembath, R.C. (2001). Altered growth responses of pulmonary artery smooth muscle cells from patients with primary pulmonary hypertension to transforming growth factor-beta(1) and bone morphogenetic proteins.
Circulation. 104, 790–5.
Nakamura, M., Matute-Bello, G., Liles, W.C., Hayashi, S., Kajikawa, O., Lin, S.M., Frevert, C.W., and Martin, T.R. (2004). Differential response of human lung epithelial cells to fasinduced apoptosis. Am J Pathol. 164, 1949–58.
Oltersdorf, T., Elmore, S.W., Shoemaker, A.R., Armstrong, R.C., Augeri, D.J., Belli, B.A., Bruncko, M., Deckwerth, T.L., Dinges, J., Hajduk, P.J., Joseph, M.K., Kitada, S., Korsmeyer, S.J., Kunzer, A.R., Letai, A., Li, C., Mitten, M.J., Nettesheim, D.G., Ng, S., Nimmer, P.M., O’Connor, J.M., Oleksijew, A., Petros, A.M., Reed, J.C., Shen, W., Tahir, S.K., Thompson, C.B., Tomaselli, K.J., Wang, B., Wendt, M.D., Zhang, H., Fesik, S.W., and Rosenberg, S.H. (2005). An inhibitor of Bcl-2 family proteins induces regression of solid tumours. Nature. 435, 677–81.
Petrache, I., Natarajan, V., Zhen, L., Medler, T.R., Richter, A.T., Cho, C., Hubbard, W.C., Berdyshev, E.V., and Tuder, R.M.
APOPTOSIS IN THE PHYSIOLOGY AND DISEASES OF THE RESPIRATORY TRACT |
229 |
(2005). Ceramide upregulation causes pulmonary cell apoptosis and emphysema-like disease in mice. Nat Med. 11, 491–8.
Plataki, M., Koutsopoulos, A.V., Darivianaki, K., Delides, G., Siafakas, N.M., and Bouros, D. (2005). Expression of apoptotic and antiapoptotic markers in epithelial cells in idiopathic pulmonary fibrosis. Chest. 127, 266–74.
Potti, A., Mukherjee, S., Petersen, R., Dressman, H.K., Bild, A., Koontz, J., Kratzke, R., Watson, M.A., Kelley, M., Ginsburg, G.S., West, M., Harpole, D.H., and Nevins, J.R. (2006). A genomic strategy to refine prognosis in early-stage non- small-cell lung cancer. N Engl J Med. 355, 570–80.
Rabe, K.F., Hurd, S., Anzueto, A., Barnes, P.J., Buist, S.A., Calverley, P., Fukuchi, Y., Jenkins, C., Rodriguez-Roisin, R., van, W.C., and Zielinski, J. (2007). Global strategy for the diagnosis, management, and prevention of chronic obstructive pulmonary disease: GOLD executive summary. Am J Respir Crit Care Med. 176, 532–55.
Rabinovitch, M. (2008). Molecular pathogenesis of pulmonary arterial hypertension. J Clin Invest. 118, 2372–9.
Radetzki, S., Kohne,¨ C.H., von Haefen, C., Gillisen, B., Sturm, I., Dorken,¨ B., and Daniel, P.T. (2002). The apoptosis promoting Bcl-2 homologues Bak and Nbk/Bik overcome drug resistance in Mdr-1-negative and Mdr-1-overexpressing breast cancer cell lines. Oncogene. 21, 227–38.
Ranger, A.M., Malynn, B.A., and Korsmeyer, S.J. (2001). Mouse models of cell death. Nat Genet. 28, 113–18.
Roth, J.A., Nguyen, D., Lawrence, D.D., Kemp, B.L., Carrasco, C.H., Ferson, D.Z., Hong, W.K., Komaki, R., Lee, J.J., Nesbitt, J.C., Pisters, K.M.W., Putnam, J.B., Schea, R., Shin, D.M., Walsh, G.L., Dolormente, M.M., Han, C.-I., Martin, F.D., Yen, N., Xu, K., Stephens, L.C., McDonnell, T.J., Mukhopadhyay, T., and Cai, D. (1996). Retrovirus-mediated wild-type p53 gene transfer to tumors of patients with lung cancer. Nat Med. 2, 985–91.
Scavo, L.M., Ertsey, R., Chapin, C.J., Allen, L., and Kitterman, J.A. (1998). Apoptosis in the development of rat and human fetal lungs. Am J Respir Cell Mol Biol. 18, 21–31.
Schittny, J.C., Djonov, V., Fine, A., and Burri, P.H. (1998). Programmed cell death contributes to postnatal lung development. Am J Respir Cell Mol Biol. 18, 786–93.
Segura-Valdez, L., Pardo, A., Gaxiola, M., Uhal, B.D., Becerril, C., and Selman, M. (2000). Upregulation of gelatinases A and B, collagenases 1 and 2, and increased parenchymal cell death in COPD. Chest. 117, 684–94.
Shapiro, S.D. (2003). Proteolysis in the lung. Eur Respir J Suppl. 44, 30s–2s.
Sordella, R., Bell, D.W., Haber, D.A., and Settleman, J. (2004). Gefitinib-sensitizing EGFR mutations in lung cancer activate anti-apoptotic pathways. Science 305, 1163–7.
Sweet-Cordereo, A., Mukherjee, S., Subramanian, A., You, H., Roix, J.J., Ladd-Acosta, C., Mesirov, J., Golub, T.R., and Jacks, T. (2005). An oncogenic KRAS2 expression signature identified by cross-species gene-expression analysis. Nat Genet. 37, 48–55.
Takata, T., Tanaka, F., Yamada, T., Yanagihara, K., Otake, Y., Kawano, Y., Nakagawa, T., Miyahara, R., Oyanagi, H., Inui, K., and Wada, H. (2001). Clinical significance of caspase-3 expression in pathologic-stage I, nonsmall-cell lung cancer.
Int J Cancer. 96, 54–60.
Tang, K., Rossiter, H.B., Wagner, P.D., and Breen, E.C. (2004). Lung-targeted VEGF inactivation leads to an emphysema phenotype in mice. J Appl Physiol. 97, 1559–66.
Thannickal, V.J. and Horowitz, J.C. (2006). Evolving concepts of apoptosis in idiopathic pulmonary fibrosis. Proc Am Thorac Soc. 3, 350–6.
Tse, C., Shoemaker, A.R., Adickes, J., Anderson, M.G., Chen, J., Jin, S., Johnson, E.F., Marsh, K.C., Mitten, M.J., Nimmer, P., Roberts, L., Tahir, S.K., Xiao, Y., Yang, X., Zhang, H., Fesik, S., Rosenberg, S.H., and Elmore, S.W. (2008). ABT-263: a potent and orally bioavailable Bcl-2 family inhibitor. Cancer Res. 68, 3421–8.
Tuder, R.M., Zhen, L., Cho, C.Y., Taraseviciene-Stewart, L., Kasahara, Y., Salvemini, D., Voelkel, N.F., and Flores, S.C. (2003). Oxidative stress and apoptosis interact and cause emphysema due to vascular endothelial growth factor receptor blockade. Am J Respir Cell Mol Biol. 29, 88–97.
Uhal, B.D., Joshi, I., Hughes, W.F., Ramos, C., Pardo, A., and Selman, M. (1998). Alveolar epithelial cell death adjacent to underlying myofibroblasts in advanced fibrotic human lung.
Am J Physiol. 275, L1192–9.
Vandivier, R.W., Fadok, V.A., Hoffmann, P.R., Bratton, D.L., Penvari, C., Brown, K.K., Brain, J.D., Accurso, F.J., and Henson, P.M. (2002). Elastase-mediated phosphatidylserine receptor cleavage impairs apoptotic cell clearance in cystic fibrosis and bronchiectasis. J Clin Invest. 109, 661–70.
Varfolomeev, E., Blankenship, J.W., Wayson, S.M., Fedorova, A.V., Kayagaki, N., Garg, P., Zobel, K., Dynek, J.N., Elliott, L.O., Wallweber, H.J., Flygare, J.A., Fairbrother, W.J., Deshayes, K., Dixit, V.M., and Vucic, D. (2007). IAP antagonists induce autoubiquitination of c-IAPs, NF-kappaB activation, and TNFalpha-dependent apoptosis. Cell, 131, 669–81.
Ventura, A., Young, A.F., Winslow, M.M., Lintault, L., Meissner, A., Erkeland, S.J., Newman, J., Bronson, R.T., Crowley, D., Stone, J.R., Jaenisch, R., Sharp, P.A., and Jacks, T. (2008). Targeted deletion reveals essential and overlapping functions of the miR-17 through 92 family of miRNA clusters. Cell. 132, 875–86.
Walczak, H., Miller, R.E., Ariail, K., Gliniak, B., Griffith, T.S., Kubin, M., Chin, W., Jones, J., Woodward, A., Le, T., Smith, C., Smolak, P., Goodwin, R.G., Rauch, C.T., Schuh, J.C.L., and Lynch, D.H. (1999). Tumoricidal activity of tumor necrosis factor related apoptosis inducing ligand in vivo. Nat Med. 5, 157–63.
Wallach-Dayan, S.B., Izbicki, G., Cohen, P.Y., Gerstl-Golan, R., Fine, A., and Breuer, R. (2006). Bleomycin initiates apoptosis of lung epithelial cells by ROS but not by Fas/FasL pathway.
Am J Physiol Lung Cell Mol Physiol. 290, L790–6.
Wang, H.C., Shun, C.T., Hsu, S.M., Kuo, S.H., Luh, K.T., and Yang, P.C. (2002). Fas/Fas ligand pathway is involved in the
230 |
CHRISTIAN TAUBE AND MARTIN SCHULER |
resolution of type II pneumocyte hyperplasia after acute lung injury: evidence from a rat model. Crit Care Med. 30, 1528–34.
Wang, R., Ibarra-Sunga, O., Verlinski, L., Pick, R., and Uhal, B.D. (2000). Abrogation of bleomycin-induced epithelial apoptosis and lung fibrosis by captopril or by a caspase inhibitor. Am J Physiol Lung Cell Mol Physiol. 279, L143–51.
Wang, R., Ramos, C., Joshi, I., Zagariya, A., Pardo, A., Selman, M., and Uhal, B.D. (1999). Human lung myofibroblastderived inducers of alveolar epithelial apoptosis identified as angiotensin peptides. Am J Physiol. 277, L1158– 64.
Wesarg, E., Hoffarth, S., Wiewrodt, R., Kroll,¨ M., Biesterfeld, S., Huber, C., and Schuler, M. (2007). Targeting BCL-2 family proteins to overcome drug resistance in non-small cell lung cancer. Int J Cancer. 121, 2387–94.
Yang, L., Mashima, T., Sato, S., Mochizuki, M., Sakamoto, H., Yamori, T., Oh-Hara, T., and Tsuruo, T. (2003). Predominant suppression of apoptosome by inhibitor of apoptosis protein in non-small cell lung cancer H460 cells: therapeutic effect
of a novel polyarginine-conjugated Smac peptide. Cancer Res. 63, 831–7.
Yokohori, N., Aoshiba, K., and Nagai, A. (2004). Increased levels of cell death and proliferation in alveolar wall cells in patients with pulmonary emphysema. Chest. 125, 626–32.
Zagariya, A., Bhat, R., Chari, G., Uhal, B., Navale, S., and Vidyasagar, D. (2005). Apoptosis of airway epithelial cells in response to meconium. Life Sci. 76, 1849–58.
Zhang, W.W., Fang, X., Mazur, W., French, B.A., Georges, R.N., and Roth, J.A. (1994). High-efficiency gene transfer and highlevel expression of wildtype p53 in human lung cancer cells mediated by recombinant adenovirus. Cancer Gene Ther. 1, 5–13.
Zheng, T., Kang, M.J., Crothers, K., Zhu, Z., Liu, W., Lee, C.G., Rabach, L.A., Chapman, H.A., Homer, R.J., Aldous, D., De Sanctis, G.T., Underwood, S., Graupe, M., Flavell, R.A., Schmidt, J.A., and Elias, J.A. (2005). Role of cathepsin S- dependent epithelial cell apoptosis in IFN-gamma-induced alveolar remodeling and pulmonary emphysema. J Immunol. 174, 8106–15.