
- •Contents
- •Contributors
- •Part I General Principles of Cell Death
- •1 Human Caspases – Apoptosis and Inflammation Signaling Proteases
- •1.1. Apoptosis and limited proteolysis
- •1.2. Caspase evolution
- •2. ACTIVATION MECHANISMS
- •2.2. The activation platforms
- •2.4. Proteolytic maturation
- •3. CASPASE SUBSTRATES
- •4. REGULATION BY NATURAL INHIBITORS
- •REFERENCES
- •2 Inhibitor of Apoptosis Proteins
- •2. CELLULAR FUNCTIONS AND PHENOTYPES OF IAP
- •3. IN VIVO FUNCTIONS OF IAP FAMILY PROTEINS
- •4. SUBCELLULAR LOCATIONS OF IAP
- •8. IAP–IAP INTERACTIONS
- •10. ENDOGENOUS ANTAGONISTS OF IAP
- •11. IAPs AND DISEASE
- •SUGGESTED READINGS
- •1. INTRODUCTION
- •2.1. The CD95 (Fas/APO-1) system
- •2.1.1. CD95 and CD95L: discovery of the first direct apoptosis-inducing receptor-ligand system
- •2.1.2. Biochemistry of CD95 apoptosis signaling
- •2.2. The TRAIL (Apo2L) system
- •3.1. The TNF system
- •3.1.1. Biochemistry of TNF signal transduction
- •3.1.2. TNF and TNF blockers in the clinic
- •3.2. The DR3 system
- •4. THE DR6 SYSTEM
- •6. CONCLUDING REMARKS AND OUTLOOK
- •SUGGESTED READINGS
- •4 Mitochondria and Cell Death
- •1. INTRODUCTION
- •2. MITOCHONDRIAL PHYSIOLOGY
- •3. THE MITOCHONDRIAL PATHWAY OF APOPTOSIS
- •9. CONCLUSIONS
- •SUGGESTED READINGS
- •1. INTRODUCTION
- •3. INHIBITING APOPTOSIS
- •4. INHIBITING THE INHIBITORS
- •6. THE BCL-2 FAMILY AND CANCER
- •SUGGESTED READINGS
- •6 Endoplasmic Reticulum Stress Response in Cell Death and Cell Survival
- •1. INTRODUCTION
- •2. THE ESR IN YEAST
- •3. THE ESR IN MAMMALS
- •4. THE ESR AND CELL DEATH
- •5. THE ESR IN DEVELOPMENT AND TISSUE HOMEOSTASIS
- •6. THE ESR IN HUMAN DISEASE
- •7. CONCLUSION
- •7 Autophagy – The Liaison between the Lysosomal System and Cell Death
- •1. INTRODUCTION
- •2. AUTOPHAGY
- •2.2. Physiologic functions of autophagy
- •2.3. Autophagy and human pathology
- •3. AUTOPHAGY AND CELL DEATH
- •3.1. Autophagy as anti–cell death mechanism
- •3.2. Autophagy as a cell death mechanism
- •3.3. Molecular players of the autophagy–cell death cross-talk
- •4. AUTOPHAGY, CELLULAR DEATH, AND CANCER
- •5. CONCLUDING REMARKS AND PENDING QUESTIONS
- •SUGGESTED READINGS
- •8 Cell Death in Response to Genotoxic Stress and DNA Damage
- •1. TYPES OF DNA DAMAGE AND REPAIR SYSTEMS
- •2. DNA DAMAGE RESPONSE
- •2.2. Transducers
- •2.3. Effectors
- •4. CHROMATIN MODIFICATIONS
- •5. CELL CYCLE CHECKPOINT REGULATION
- •6. WHEN REPAIR FAILS: SENESCENCE VERSUS APOPTOSIS
- •6.1. DNA damage response and the induction of apoptosis
- •6.2. p53-independent mechanisms of apoptosis
- •6.3. DNA damage response and senescence induction
- •7. DNA DAMAGE FROM OXIDATIVE STRESS
- •SUGGESTED READINGS
- •9 Ceramide and Lipid Mediators in Apoptosis
- •1. INTRODUCTION
- •3.1. Basic cell signaling often involves small molecules
- •3.2. Sphingolipids are cell-signaling molecules
- •3.2.1. Ceramide induces apoptosis
- •3.2.2. Ceramide accumulates during programmed cell death
- •3.2.3. Inhibition of ceramide production alters cell death signaling
- •4.1. Ceramide is generated through SM hydrolysis
- •4.3. aSMase can be activated independently of extracellular receptors to regulate apoptosis
- •4.4. Controversial aspects of the role of aSMase in apoptosis
- •4.5. De novo ceramide synthesis regulates programmed cell death
- •4.6. p53 and Bcl-2–like proteins are connected to de novo ceramide synthesis
- •4.7. The role and regulation of de novo synthesis in ceramide-mediated cell death is poorly understood
- •5. CONCLUDING REMARKS AND FUTURE DIRECTIONS
- •5.1. Who? (Which enzyme?)
- •5.2. What? (Which ceramide?)
- •5.3. Where? (Which compartment?)
- •5.4. When? (At what steps?)
- •5.5. How? (Through what mechanisms?)
- •5.6. What purpose?
- •6. SUMMARY
- •SUGGESTED READINGS
- •1. General Introduction
- •1.1. Cytotoxic lymphocytes and apoptosis
- •2. CYTOTOXIC GRANULES AND GRANULE EXOCYTOSIS
- •2.1. Synthesis and loading of the cytotoxic granule proteins into the secretory granules
- •2.2. The immunological synapse
- •2.3. Secretion of granule proteins
- •2.4. Uptake of proapoptotic proteins into the target cell
- •2.5. Activation of death pathways by granzymes
- •3. GRANULE-BOUND CYTOTOXIC PROTEINS
- •3.1. Perforin
- •3.2. Granulysin
- •3.3. Granzymes
- •3.3.1. GrB-mediated apoptosis
- •3.3.2. GrA-mediated cell death
- •3.3.3. Orphan granzyme-mediated cell death
- •5. CONCLUSIONS
- •REFERENCES
- •Part II Cell Death in Tissues and Organs
- •1.1. Death by trophic factor deprivation
- •1.2. Key molecules regulating neuronal apoptosis during development
- •1.2.1. Roles of caspases and Apaf-1 in neuronal cell death
- •1.2.2. Role of Bcl-2 family members in neuronal cell death
- •1.3. Signal transduction from neurotrophins and neurotrophin receptors
- •1.3.1. Signals for survival
- •1.3.2. Signals for death
- •2.1. Apoptosis in neurodegenerative diseases
- •2.1.4. Amyotrophic lateral sclerosis
- •2.2. Necrotic cell death in neurodegenerative diseases
- •2.2.1. Calpains
- •2.2.2. Cathepsins
- •3. CONCLUSIONS
- •ACKNOWLEDGMENT
- •SUGGESTED READINGS
- •ACKNOWLEDGMENT
- •SUGGESTED READINGS
- •1. INTRODUCTION
- •5. S-NITROSYLATION OF PARKIN
- •7. POTENTIAL TREATMENT OF EXCESSIVE NMDA-INDUCED Ca2+ INFLUX AND FREE RADICAL GENERATION
- •8. FUTURE THERAPEUTICS: NITROMEMANTINES
- •9. CONCLUSIONS
- •Acknowledgments
- •SUGGESTED READINGS
- •3. MITOCHONDRIAL PERMEABILITY TRANSITION ACTIVATED BY Ca2+ AND OXIDATIVE STRESS
- •4.1. Mitochondrial apoptotic pathways
- •4.2. Bcl-2 family proteins
- •4.3. Caspase-dependent apoptosis
- •4.4. Caspase-independent apoptosis
- •4.5. Calpains in ischemic neural cell death
- •5. SUMMARY
- •ACKNOWLEDGMENTS
- •SUGGESTED READINGS
- •1. INTRODUCTION
- •2. HISTORICAL ANTECEDENTS
- •7.1. Activation of p21 waf1/cip1: Targeting extrinsic and intrinsic pathways to death
- •8. CONCLUSION
- •ACKNOWLEDGMENTS
- •REFERENCES
- •16 Apoptosis and Homeostasis in the Eye
- •1.1. Lens
- •1.2. Retina
- •2. ROLE OF APOPTOSIS IN DISEASES OF THE EYE
- •2.1. Glaucoma
- •2.2. Age-related macular degeneration
- •4. APOPTOSIS AND OCULAR IMMUNE PRIVILEGE
- •5. CONCLUSIONS
- •SUGGESTED READINGS
- •17 Cell Death in the Inner Ear
- •3. THE COCHLEA IS THE HEARING ORGAN
- •3.1. Ototoxic hair cell death
- •3.2. Aminoglycoside-induced hair cell death
- •3.3. Cisplatin-induced hair cell death
- •3.4. Therapeutic strategies to prevent hair cell death
- •3.5. Challenges to studies of hair cell death
- •4. SPIRAL GANGLION NEURON DEATH
- •4.1. Neurotrophic support from sensory hair cells and supporting cells
- •4.2. Afferent activity from hair cells
- •4.3. Molecular manifestations of spiral ganglion neuron death
- •4.4. Therapeutic interventions to prevent SGN death
- •ACKNOWLEDGMENTS
- •SUGGESTED READINGS
- •18 Cell Death in the Olfactory System
- •1. Introduction
- •2. Anatomical Aspects
- •3. Life and Death in the Olfactory System
- •3.1. Olfactory epithelium
- •3.2. Olfactory bulb
- •REFERENCES
- •1. Introduction
- •3.1. Beta cell death in the development of T1D
- •3.2. Mechanisms of beta cell death in type 1 diabetes
- •3.2.1. Apoptosis signaling pathways downstream of death receptors and inflammatory cytokines
- •3.2.2. Oxidative stress
- •3.3. Mechanisms of beta cell death in type 2 diabetes
- •3.3.1. Glucolipitoxicity
- •3.3.2. Endoplasmic reticulum stress
- •5. SUMMARY
- •Acknowledgments
- •REFERENCES
- •20 Apoptosis in the Physiology and Diseases of the Respiratory Tract
- •1. APOPTOSIS IN LUNG DEVELOPMENT
- •2. APOPTOSIS IN LUNG PATHOPHYSIOLOGY
- •2.1. Apoptosis in pulmonary inflammation
- •2.2. Apoptosis in acute lung injury
- •2.3. Apoptosis in chronic obstructive pulmonary disease
- •2.4. Apoptosis in interstitial lung diseases
- •2.5. Apoptosis in pulmonary arterial hypertension
- •2.6. Apoptosis in lung cancer
- •SUGGESTED READINGS
- •21 Regulation of Cell Death in the Gastrointestinal Tract
- •1. INTRODUCTION
- •2. ESOPHAGUS
- •3. STOMACH
- •4. SMALL AND LARGE INTESTINE
- •5. LIVER
- •6. PANCREAS
- •7. SUMMARY AND CONCLUDING REMARKS
- •SUGGESTED READINGS
- •22 Apoptosis in the Kidney
- •1. NORMAL KIDNEY STRUCTURE AND FUNCTION
- •3. APOPTOSIS IN ADULT KIDNEY DISEASE
- •4. REGULATION OF APOPTOSIS IN KIDNEY CELLS
- •4.1. Survival factors
- •4.2. Lethal factors
- •4.2.1. TNF superfamily cytokines
- •4.2.2. Other cytokines
- •4.2.3. Glucose
- •4.2.4. Drugs and xenobiotics
- •4.2.5. Ischemia-reperfusion and sepsis
- •5. THERAPEUTIC APPROACHES
- •SUGGESTED READINGS
- •1. INTRODUCTION
- •2. APOPTOSIS IN THE NORMAL BREAST
- •2.1. Occurrence and role of apoptosis in the developing breast
- •2.2.2. Death ligands and death receptor pathway
- •2.2.4. LIF-STAT3 proapoptotic signaling
- •2.2.5. IGF survival signaling
- •2.2.6. Regulation by adhesion
- •2.2.7. PI3K/AKT pathway: molecular hub for survival signals
- •2.2.8. Downstream regulators of apoptosis: the BCL-2 family members
- •3. APOPTOSIS IN BREAST CANCER
- •3.1. Apoptosis in breast tumorigenesis and cancer progression
- •3.2. Molecular dysregulation of apoptosis in breast cancer
- •3.2.1. Altered expression of death ligands and their receptors in breast cancer
- •3.2.2. Deregulation of prosurvival growth factors and their receptors
- •3.2.3. Alterations in cell adhesion and resistance to anoikis
- •3.2.4. Enhanced activation of the PI3K/AKT pathway in breast cancer
- •3.2.5. p53 inactivation in breast cancer
- •3.2.6. Altered expression of BCL-2 family of proteins in breast cancer
- •5. CONCLUSION
- •SUGGESTED READINGS
- •1. INTRODUCTION
- •2. DETECTING CELL DEATH IN THE FEMALE GONADS
- •4. APOPTOSIS AND FEMALE REPRODUCTIVE AGING
- •6. CONCLUDING REMARKS
- •REFERENCES
- •25 Apoptotic Signaling in Male Germ Cells
- •1. INTRODUCTION
- •3.1. Murine models
- •3.2. Primate models
- •3.3. Pathways of caspase activation and apoptosis
- •3.4. Apoptotic signaling in male germ cells
- •5. P38 MITOGEN-ACTIVATED PROTEIN KINASE (MAPK) AND NITRIC OXIDE (NO)–MEDIATED INTRINSIC PATHWAY SIGNALING CONSTITUTES A CRITICAL COMPONENT OF APOPTOTIC SIGNALING IN MALE GERM CELLS AFTER HORMONE DEPRIVATION
- •11. CONCLUSIONS AND PERSPECTIVES
- •REFERENCES
- •26 Cell Death in the Cardiovascular System
- •1. INTRODUCTION
- •2. CELL DEATH IN THE VASCULATURE
- •2.1. Apoptosis in the developing blood vessels
- •2.2. Apoptosis in atherosclerosis
- •2.2.1. Vascular smooth muscle cells
- •2.2.2. Macrophages
- •2.2.3. Regulation of apoptosis in atherosclerosis
- •2.2.4. Necrosis and autophagy in atherosclerosis
- •3. CELL DEATH IN THE MYOCARDIUM
- •3.1. Cell death in myocardial infarction
- •3.1.1. Apoptosis in myocardial infarction
- •3.1.2. Necrosis in myocardial infarction
- •3.1.3. Autophagy in myocardial infarction
- •3.2. Cell death in heart failure
- •3.2.1. Apoptosis in heart failure
- •3.2.2. Necrosis in heart failure
- •3.2.3. Autophagy in heart failure
- •4. CONCLUDING REMARKS
- •ACKNOWLEDGMENTS
- •REFERENCES
- •27 Cell Death Regulation in Muscle
- •1. INTRODUCTION TO MUSCLE
- •1.1. Skeletal muscle adaptation to endurance training
- •1.2. Myonuclear domains
- •2. MITOCHONDRIALLY MEDIATED APOPTOSIS IN MUSCLE
- •2.1. Skeletal muscle apoptotic susceptibility
- •4. APOPTOSIS IN MUSCLE DURING AGING AND DISEASE
- •4.1. Aging
- •4.2. Type 2 diabetes mellitus
- •4.3. Cancer cachexia
- •4.4. Chronic heart failure
- •6. CONCLUSION
- •SUGGESTED READINGS
- •28 Cell Death in the Skin
- •1. INTRODUCTION
- •2. CELL DEATH IN SKIN HOMEOSTASIS
- •2.1. Cornification and apoptosis
- •2.2. Death receptors in the skin
- •3. CELL DEATH IN SKIN PATHOLOGY
- •3.1. Sunburn
- •3.2. Skin cancer
- •3.3. Necrolysis
- •3.4. Pemphigus
- •3.5. Eczema
- •3.6. Graft-versus-host disease
- •4. CONCLUDING REMARKS AND PERSPECTIVES
- •ACKNOWLEDGMENTS
- •SUGGESTED READINGS
- •29 Apoptosis and Cell Survival in the Immune System
- •2.1. Survival of early hematopoietic progenitors
- •2.2. Sizing of the T-cell population
- •2.2.1. Establishing central tolerance
- •2.2.2. Peripheral tolerance
- •2.2.3. Memory T cells
- •2.3. Control of apoptosis in B-cell development
- •2.3.1. Early B-cell development
- •2.3.2. Deletion of autoreactive B cells
- •2.3.3. Survival and death of activated B cells
- •3. IMPAIRED APOPTOSIS AND LEUKEMOGENESIS
- •4. CONCLUSIONS
- •ACKNOWLEDGMENTS
- •REFERENCES
- •30 Cell Death Regulation in the Hematopoietic System
- •1. INTRODUCTION
- •2. HEMATOPOIETIC STEM CELLS
- •4. ERYTHROPOIESIS
- •5. MEGAKARYOPOIESIS
- •6. GRANULOPOIESIS
- •7. MONOPOIESIS
- •8. CONCLUSION
- •ACKNOWLEDGMENTS
- •REFERENCES
- •31 Apoptotic Cell Death in Sepsis
- •1. INTRODUCTION
- •2. HOST INFLAMMATORY RESPONSE TO SEPSIS
- •3. CLINICAL OBSERVATIONS OF CELL DEATH IN SEPSIS
- •3.1. Sepsis-induced apoptosis
- •3.2. Necrotic cell death in sepsis
- •4.1. Central role of apoptosis in sepsis mortality: immune effector cells and gut epithelium
- •4.2. Apoptotic pathways in sepsis-induced immune cell death
- •4.3. Investigations implicating the extrinsic apoptotic pathway in sepsis
- •4.4. Investigations implicating the intrinsic apoptotic pathway in sepsis
- •5. THE EFFECT OF APOPTOSIS ON THE IMMUNE SYSTEM
- •5.1. Cellular effects of an increased apoptotic burdens
- •5.2. Network effects of selective loss of immune cell types
- •5.3. Studies of immunomodulation by apoptotic cells in other fields
- •7. CONCLUSION
- •REFERENCES
- •32 Host–Pathogen Interactions
- •1. INTRODUCTION
- •2. FROM THE PATHOGEN PERSPECTIVE
- •2.1. Commensals versus pathogens
- •2.2. Pathogen strategies to infect the host
- •3. HOST DEFENSE
- •3.1. Antimicrobial peptides
- •3.2. PRRs and inflammation
- •3.2.1. TLRs
- •3.2.2. NLRs
- •3.2.3. The Nod signalosome
- •3.2.4. The inflammasome
- •3.3. Cell death
- •3.3.1. Apoptosis and pathogen clearance
- •3.3.2. Pyroptosis
- •3.2.3. Caspase-independent cell death
- •3.2.4. Autophagy and autophagic cell death
- •4. CONCLUSIONS
- •REFERENCES
- •Part III Cell Death in Nonmammalian Organisms
- •1. PHENOTYPE AND ASSAYS OF YEAST APOPTOSIS
- •2.1. Pheromone-induced cell death
- •2.1.1. Colony growth
- •2.1.2. Killer-induced cell death
- •3. EXTERNAL STIMULI THAT INDUCE APOPTOSIS IN YEAST
- •4. THE GENETICS OF YEAST APOPTOSIS
- •5. PROGRAMMED AND ALTRUISTIC AGING
- •SUGGESTED READINGS
- •34 Caenorhabditis elegans and Apoptosis
- •1. Overview
- •2. KILLING
- •3. SPECIFICATION
- •4. EXECUTION
- •4.1. DNA degradation
- •4.2. Mitochondrial elimination
- •4.3. Engulfment
- •5. SUMMARY
- •SUGGESTED READINGS
- •35 Apoptotic Cell Death in Drosophila
- •2. DROSOPHILA CASPASES AND PROXIMAL REGULATORS
- •6. CLOSING COMMENTS
- •SUGGESTED READINGS
- •36 Analysis of Cell Death in Zebrafish
- •1. INTRODUCTION
- •2. WHY USE ZEBRAFISH TO STUDY CELL DEATH?
- •2.2. Molecular techniques to rapidly assess gene function in embryos
- •2.2.1. Studies of gene function using microinjections into early embryos
- •2.2.2. In situ hybridization and immunohistochemistry
- •2.3. Forward genetic screening
- •2.4. Drug and small-molecule screening
- •2.5. Transgenesis
- •2.6. Targeted knockouts
- •3.1. Intrinsic apoptosis
- •3.2. Extrinsic apoptosis
- •3.3. Chk-1 suppressed apoptosis
- •3.4. Anoikis
- •3.5. Autophagy
- •3.6. Necrosis
- •4. DEVELOPMENTAL CELL DEATH IN ZEBRAFISH EMBRYOS
- •5. THE P53 PATHWAY
- •6. PERSPECTIVES AND FUTURE DIRECTIONS
- •SUGGESTED READING
35 Apoptotic Cell Death in Drosophila
Kathleen Galindo and John M. Abrams
In animals, programmed cell death (PCD) is a universal feature of development and is critical for adaptive responses during cellular injury. In both vertebrates and invertebrates, dying cells often progress through a series of ultrastructural changes referred to as apoptosis. This form of PCD involves condensation of nuclear material and fragmentation into “apoptotic bodies” that are eventually engulfed by phagocytes. It is well established that apoptosis requires genetic functions within the dying cell and that the underlying molecular machinery is evolutionarily conserved. Studies in model systems have elaborated common control points in pathways that regulate cell death. However, when considered within larger networks of interactions, the importance of these regulatory “linchpins” can vary across different cell types and across different species. Here, we consider these similarities and differences and discuss how the Drosophila model organism may shed light on evolutionary pressures that fundamentally shaped this biological process.
1. CORE MOLECULES SPECIFYING APOPTOTIC CELL
DEATH ARE CONSERVED
Seminal discoveries that illuminated core regulators of cell death were made in Caenorhabditis elegans. Prompted by the cloning of CED genes necessary for PCD in the nematode, our collective understanding of apoptotic cell death has grown at an astonishing pace. In this model system, precisely 131 of 1,090 cells undergo programmed cell death during development. Key molecules that specify worm PCD participate in precise interactions. These include the proapoptotic BH3only Bcl-2 family homolog, egl-1, the antiapoptotic Bcl- 2 family member, ced-9, the Apaf-1 ortholog, ced-4, and
the cysteine protease, ced-3 (Figure 35-1). ced-4 and ced- 3 are required for all cell deaths to occur, whereas, ced-9 is required to prevent cell death. In worms, CED-9 physically binds to and inhibits CED-4, preventing activation of CED-3. The cell death machinery is activated when EGL-1 binds to CED-9 at the mitochondria, displacing bound CED-4, which is now free to bind to and activate CED-3. In this model, EGL-1 is a critical PCD determinant, and its presence or absence in any given cell is tightly controlled by transcriptional regulation.
Family members homologous to CED genes were found in diverse vertebrate models and in Drosophila, establishing that core components of the “apoptotic engine” are highly conserved. Figure 35-1 shows a prevailing model for the functional action of these genes and their counterparts in mammals and flies. In each organism, this genetic circuit controls activation of an apical caspase function via a multimeric complex referred to as the apoptosome. Caspases, in turn, mediate PCD by cleaving selected proteins, thereby reorganizing cellular physiology and promoting self-destruction. The fundamental importance of caspase action during apoptosis is underscored by the discovery of viral antiapoptotic factors, such as p35 and inhibitors of apoptosis proteins (IAPs) that function as potent caspase inhibitors.
2. DROSOPHILA CASPASES AND PROXIMAL REGULATORS
Caspases are synthesized as dormant proenzymes, and during apoptosis, these precursors are processed to their active mature form by complex networks of proteolytic activation. Although caspase function is generally associated with apoptosis and immunity, recent discoveries from Drosophila and other models systems have
407
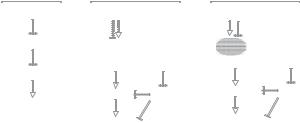
408 |
KATHLEEN GALINDO AND JOHN M. ABRAMS |
Nematodes |
Flies |
|
Mammals |
|
EGL-1 |
Bcl2 family |
|
Bcl2 family |
|
CED-9 |
|
Reaper |
Cyt C |
Smac |
|
|
Grim |
||
|
|
|
||
|
|
Apaf-1 |
Diablo |
|
CED-4 |
Ark |
Hid |
||
|
|
|
|
|
|
Dronc |
DIAP1 |
Caspase-9 |
IAPs |
CED-3 |
|
|
|
|
Effector |
Effector |
|
caspases |
||
caspases |
||
|
Figure 35-1. CED-3 represents the founding member of the proapoptotic cysteine proteases known as caspases, whereas the antiapoptotic gene, CED-9, shows structural and functional homology to the Bcl-2 family of proteins. A third gene, CED-4, bears homology to vertebrate APAF-1 and Drosophila Ark. These proteins activate apical caspase function via a multimeric complex referred to as the “apoptosome.” Caspases in turn mediate PCD by cleaving selected proteins, thereby reorganizing cellular physiology and promoting self-destruction. An emerging picture for apoptosis control is consistent with a “gas and brake” model, whereby concurrent input from APAF-1/Dark adaptors, together with removal of IAP inhibition, drives caspase activation to levels that exceed a threshold necessary for apoptosis. From this viewpoint, a balance of opposing regulatory forces determines the status of apical caspase activity, but the relative contribution from positive regulators (APAF-1/Ced-4/Dark) and negative regulators (the IAP family) can vary among di erent cell types and species. For example, in the worm, mouse, and fly, mutations in positive regulators unanimously cause pronounced cell death defective phenotypes, but this is not consistently true with respect to the negative regulators. Therefore, although underlying components are broadly conserved, the regulatory linchpins in di erent cells and in di erent species can vary.
linked this family of enzymes to other functions, including cell type differentiation, sperm production, and migration. Drosophila is a premiere system for genetics, and in this genome, seven members of the caspase family are found. Flies have three initiator/apical caspases, Dronc, Dredd, and Strica (characterized by the presence of long N-terminal prodomains), and four effector caspases, Drice, Decay, Dcp-1, and Damm (characterized by short prodomains). Of the three apical caspases, Dronc is the primary apical caspase whose main function is in the apoptotic process. Dronc contains an N-terminal caspase recruitment domain (CARD), which binds to the CARD domain of the Drosophila Apaf-1 ortholog, Ark, to form a high-molecular-weight complex. Ark and Dronc, combined with dATP, form this multiprotein complex referred to as the apoptosome. On recruitment to the apoptosome, Dronc is processed and activated, which in turn perpetuates an amplified signal. This mode of recruitment and apoptosome formation is similar from worms to mammals, except that in mammals, cytochrome c is an essential component of this complex. Ark, the Drosophila Apaf-1 homolog,
contains WD40 repeats, a motif known for binding to cytochrome c in mammalian Apaf-1, but curiously, binding to cytochrome c is not required for formation of the fly apoptosome.
Evidence that Ark and Dronc are essential players in the Drosophila cell death pathway comes from genetic studies in which elimination of either gene results in almost complete inhibition of all PCD. Interestingly, however, when ark and dronc function are lost, not all cell deaths are abolished, and a few sporadic cell deaths are observed. Hence it seems likely that other components in the cell death pathway may compensate for the lack of a functional apoptosome, or perhaps redundant and/or parallel PCD pathways exist.
To date, known substrates of Dronc include the effector caspases Dcp-1 and Drice, both of which share homology with the mammalian effector caspase-3 and -7. Additionally, they are also sensitive to inhibition by the baculovirus caspase inhibitor, p35, which inhibits Dronc-dependent cell death, but not Dronc itself. dcp-1 mutant animals are relatively healthy, exhibiting defects only in starvation-induced cell death during oogenesis. On the other hand, drice mutants exhibit marked reduced cell death in a variety of tissues, which produce semi-viable phenotypes. Interestingly, animals doubly mutated for dcp-1 and drice produce cell death defective phenotypes that are far more severe than either single gene mutant alone, indicating that these two effector caspases may share partially redundant roles in
Drosophila PCD.
3. IAPS PARTICIPATE IN CASPASE-DEPENDENT
CELL DEATH
A second critical control point in fly PCD involves negative regulators of caspase activity known as IAPs. These proteins were discovered as potent baculoviral factors that function to suppress host cell death on infection. As a protein family, IAPs are defined by a 70–amino acid signature motif, known as the baculovirus IAP repeat (BIR), which mediates protein–protein interactions. Many members of this family also contain really interesting new gene (RING) finger domains associated with ubiquitin conjugation and E3 ligase activity. IAPs have received considerable attention because some members of this family physically bind – and directly inhibit – apoptotic caspases. The Drosophila genome encodes 3 IAPs: Diap-1, Diap-2, and dBruce. Diap-1 occupies a pivotal role in apoptosis pathways, and as a direct inhibitor, the gene and its protein product are the subject of intense study. In this model
APOPTOTIC CELL DEATH IN DROSOPHILA |
409 |
system, loss of diap-1 causes extensive cell death, and conversely, over-expression of diap-1 blocks cell death. Interestingly, ectopic cell death caused by loss of diap- 1 is suppressed when levels of ark, dronc, or drice are decreased, highlighting its primary prosurvival function as a physiologic inhibitor of caspases. Diap-1 directly binds to Drice and Dcp-1 and physically prevents them from cleaving substrates by steric hindrance. Diap-1 also physically binds Dronc, but in this case, a distinct mechanism of negative regulation is used. Rather than steric hindrance or competitive inhibition, the E3 ligase activity of DIAP1 renders Dronc inactive by promoting ubiquitination and degradation of this apical caspase. Through its E3 ligase activity, Diap-1 also promotes ubiquitination and degradation of other proapoptotic proteins, such as Rpr, and also participates in autoubiquitation, thus targeting itself for proteosome-dependent degradation.
The discovery of BIR-containing genes in fly and vertebrate genomes, combined with discoveries that some IAPs can inhibit activated caspases through direct physical interactions, lends credibility to the idea that viruses acquired antiapoptotic molecules from their host genomes. This premise is strongly supported by genetic and biochemical evidence and is entirely consistent with the finding that DIAP1 is a pivotal apoptotic determinant in the fly. As a protein family, human IAPs are attractive therapeutic targets, and some preclinical findings validate applications for both antagonists and mimetics. In humans, as in flies, IAP-mediated inhibition of caspases involves direct physical contact between BIR domains and zymogenic forms of enzyme, and although the precise in vivo mechanism is not fully understood, it is already clear that ubiquitin-mediated protein degradation is important.
4. RPR PROTEINS ARE IAP ANTAGONISTS WITH
CONSERVED BINDING PROPERTIES
Like many enzyme systems in any given cell, there exists a fine balance in the levels of processed caspases and IAPs. So how is this equilibrium tipped toward irreversible apoptotic cell death? The class of Reaper proteins, Rpr, Grim, Hid, and Skl (also known as RHG proteins), play important roles in tipping the balance in the direction of cell death. These proteins are potent apoptosis activators in Drosophila, and if heterologously expressed in vertebrate or human cell types, Reaper proteins are also sufficient to provoke acute apoptotic death in these systems as well. RHG proteins reside in the genomic interval known as the Reaper region, and when
deleted, nearly all apoptotic cell death is abolished. Reaper proteins are often expressed in patterns that anticipate PCD, and transcriptional regulation appears to be an important mechanism regulating the activity of these genes.
Once expressed, RHG proteins promote cell death through mechanisms that depend on binding to Diap-1. RPR proteins share an N-terminal motif referred to as the RHG motif or the IAP-binding motif (IBM). Residues within this motif can form direct contacts with the BIR domains of DIAP1, suggesting compelling models for how these proteins specify cell death. Although the precise mechanisms are not completely understood, RPR proteins and a fifth RHG containing protein, Jafrac2, are thought to antagonize DIAP1 through direct interactions that displace processed caspases (DRONC, DRICE, and DCP1) from negative regulation imposed by this IAP. In this way, derepression by RPR proteins, each with distinct affinities for a given BIR domain, promote liberation of apical and/or effector caspases from constitutive restraint by IAPs. Another way that RHG proteins regulate Diap-1 function involves ubiquitination (and autoubiquitination) activities, mediated by the RING domain in Diap1 that destabilize the protein. In mammals, orthologous activity is encoded by the Smac proteins, which similarly antagonize IAP function. Where examined, structural parallels and analogous contacts solved for the fly and mammalian complexes underscored common mechanisms of action for the fly and mammalian IAP antagonists.
Hence the balance of Diap-1, Dronc, and RHG proteins defines a critical control point in cell death regulation, where the family of RHG proteins play major roles by modulating DIAP1 stability and competing for binding partners. These protein–protein interactions specify apoptotic death in the sense that they determine whether caspase action surpasses a necessary threshold or exceeds a certain tipping point. Overall, the family of RPR proteins may be viewed as parallel switches that ultimately activate common caspase effectors. According to this model, apoptosis in development is specified by combinatorial expression patterns and selective constraints operating on these and perhaps other death activators.
5. DROSOPHILA: WHAT IS UPSTREAM OF
THE APOPTOSOME?
In worms, the Bcl-2–mediated regulation of PCD occurs through direct physical interactions. Specifically,
410 |
KATHLEEN GALINDO AND JOHN M. ABRAMS |
Ced-9 physically binds and represses Ced-4 from activating Ced-3. However, in mammals, Bcl-2 proteins indirectly impact the apoptosome by regulating mitochondrial outer membrane permeability and specifying release of cytochrome c, a critical factor that promotes apoptosome assembly (see Figure 35-1). Thus, although Bcl-2 counterparts in worms and mammals occupy similar positions in the apoptosis pathway, they exert control over the apoptotic fate through distinct mechanisms. Furthermore, mammals have several members of both the antiand proapoptotic members of Bcl-2 proteins. There also exists a family of proapoptotic Bcl-2 family members known as the BH3-only proteins. These proteins function to specify cell death by either inhibiting the antiapoptotic Bcl-2 proteins directly or by binding to and promoting the proapoptotic Bcl-2 family members. The antiapoptotic family of Bcl-2 proteins has been shown to play roles in preserving mitochondrial membrane integrity to prevent release of cytochrome c into the cytosol. The proapoptotic Bcl-2 proteins have been shown to promote cytochrome c release from the mitochondria, which initiates formation of the apoptosome complex by associating with WD40 repeat domains in monomeric Apaf-1 and inducing a conformational change in Apaf-1.
The Drosophila counterpart of Apaf-1, Ark, also contains WD40 repeats capable of binding to cytochrome c, and there is some evidence suggesting that cytochrome c may be altered during apoptosis. However, it is also clear that cytochrome c is not needed to form the apoptosome in vitro, and in vivo studies indicate that cytochrome c is not rate-limiting for apoptosome cell death. Hence the emerging data indicate little or no role for cytochrome c as a positive regulator of the apoptosome. Although genetic evidence supports this inference, a definitive resolution is complicated by the fact that two cytochrome c genes are encoded in the Drosophila genome. The fly genome also encodes two multidomain Bcl-2 family members, designated debcl and buffy. These share the canonical bcl-2 homology domains that define this protein family. On the basis of over-expression and gene silencing studies, buffy is considered an antiapoptotic gene, and debcl is thought to encode proapoptotic activity; recent genetic studies, however, suggest only limited roles for these in normal PCD.
6. CLOSING COMMENTS
Caspases are thought to be universal effectors of apoptotic cell death. These enzymes are synthesized as dormant “prozymogens,” and during apoptosis, they are
activated through complex cascades of proteolytic activation. By promoting the cleavage of selected substrates, these events ultimately reorganize cellular physiology and promote self-destruction. The network of regulatory molecules that control caspase action appears to be well conserved across species. In flies and mammals, an emerging picture for apoptosis control is consistent with a “gas and brake” model, whereby concurrent input from APAF-1/Dark adaptors, together with removal of IAP inhibition, drives caspase activation to levels that exceed a threshold necessary for apoptosis. From this viewpoint, the balance of opposing regulatory forces determines the status of apical caspase activity, but the relative contribution from positive regulators (APAF-1/ Ced-4/Dark) and negative regulators (the IAP family) can vary among different cell types and species. For example, in the worm, mouse, and fly, mutations in positive regulators cause global cell death defective phenotypes, but this is not consistently true with respect to the negative regulators (IAPs). Therefore, although underlying components are broadly conserved, the regulatory linchpins in different cells and in different species can vary. Likewise, exit of cytochrome c from mitochondria appears to be a critical point of no return in mammalian cells but is unlikely to define the point of no return in either Drosophila or nematode systems. Consistent with this, elimination of the apoptosome or application of broad-spectrum caspase inhibitors preserves cell survival in both the fly and worm – but does not rescue mammalian cells from caspase-independent cell death. These differences among model systems can be exploited to illuminate important pathogenic states. For example, like fly cells in developing tissues, many human cancer cells also appear to rely heavily on IAP regulation as a means to prevent apoptogenic outcomes.
In closing, we note that prevailing models of apoptosis control are valuable, but as sometimes underscored by rigorous genetic analyses, they may also be inadequate in significant ways. For example, mice mutated for core pathway elements expose undefined alternate death pathways, independent of bax/bak, and likewise, both mouse and fly systems suggest that apoptogenic factors other than cytochrome c may impinge on the apoptosome. Other controversies focus on attempts to define the “point of no return,” which in principal represents a transition from a state in which cells could be rescued to a state in which protection is not possible. Here the Drosophila model may be particularly helpful because growing evidence for effector caspase activation without apoptosis suggests that pivotal