
- •Contents
- •Contributors
- •Preface
- •1 Introduction, with the biological basis for cell mechanics
- •Introduction
- •The role of cell mechanics in biological function
- •Maintenance of cell shape
- •Cell migration
- •Mechanosensing
- •Stress responses and the role of mechanical forces in disease
- •Active cell contraction
- •Structural anatomy of a cell
- •The extracellular matrix and its attachment to cells
- •Transmission of force to the cytoskeleton and the role of the lipid bilayer
- •Intracellular structures
- •Overview
- •References
- •2 Experimental measurements of intracellular mechanics
- •Introduction
- •Forces to which cells are exposed in a biological context
- •Methods to measure intracellular rheology by macrorheology, diffusion, and sedimentation
- •Whole cell aggregates
- •Sedimentation of particles
- •Diffusion
- •Mechanical indentation of the cell surface
- •Glass microneedles
- •Cell poker
- •Atomic force microscopy
- •Mechanical tension applied to the cell membrane
- •Shearing and compression between microplates
- •Optical traps
- •Magnetic methods
- •Twisting of magnetized particles on the cell surface and interior
- •Passive microrheology
- •Optically detected individual probes
- •One-particle method
- •Two-particle methods
- •Dynamic light scattering and diffusing wave spectroscopy
- •Fluorescence correlation spectroscopy
- •Optical stretcher
- •Acoustic microscopy
- •Outstanding issues and future directions
- •References
- •3 The cytoskeleton as a soft glassy material
- •Introduction
- •Magnetic Twisting Cytometry (MTC)
- •Measurements of cell mechanics
- •The structural damping equation
- •Reduction of variables
- •Universality
- •Scaling the data
- •Collapse onto master curves
- •Theory of soft glassy rheology
- •What are soft glassy materials
- •Sollich’s theory of SGMs
- •Soft glassy rheology and structural damping
- •Open questions
- •Biological insights from SGR theory
- •Malleability of airway smooth muscle
- •Conclusion
- •References
- •4 Continuum elastic or viscoelastic models for the cell
- •Introduction
- •Purpose of continuum models
- •Principles of continuum models
- •Boundary conditions
- •Mechanical and material characteristics
- •Example of studied cell types
- •Blood cells: leukocytes and erythrocytes
- •Limitations of continuum model
- •Conclusion
- •References
- •5 Multiphasic models of cell mechanics
- •Introduction
- •Biphasic poroviscoelastic models of cell mechanics
- •Analysis of cell mechanical tests
- •Micropipette aspiration
- •Cells
- •Biphasic properties of the pericellular matrix
- •Indentation studies of cell multiphasic properties
- •Analysis of cell–matrix interactions using multiphasic models
- •Summary
- •References
- •6 Models of cytoskeletal mechanics based on tensegrity
- •Introduction
- •The cellular tensegrity model
- •The cellular tensegrity model
- •Do living cells behave as predicted by the tensegrity model?
- •Circumstantial evidence
- •Prestress-induced stiffening
- •Action at a distance
- •Do microtubules carry compression?
- •Summary
- •Examples of mathematical models of the cytoskeleton based on tensegrity
- •The cortical membrane model
- •Tensed cable nets
- •Cable-and-strut model
- •Summary
- •Tensegrity and cellular dynamics
- •Conclusion
- •Acknowledgement
- •References
- •7 Cells, gels, and mechanics
- •Introduction
- •Problems with the aqueous-solution-based paradigm
- •Cells as gels
- •Cell dynamics
- •Gels and motion
- •Secretion
- •Muscle contraction
- •Conclusion
- •Acknowledgement
- •References
- •8 Polymer-based models of cytoskeletal networks
- •Introduction
- •The worm-like chain model
- •Force-extension of single chains
- •Dynamics of single chains
- •Network elasticity
- •Nonlinear response
- •Discussion
- •References
- •9 Cell dynamics and the actin cytoskeleton
- •Introduction: The role of actin in the cell
- •Interaction of the cell cytoskeleton with the outside environment
- •The role of cytoskeletal structure
- •Actin mechanics
- •Actin dynamics
- •The emergence of actin dynamics
- •The intrinsic dynamics of actin
- •Regulation of dynamics by actin-binding proteins
- •Capping protein: ‘decommissioning’ the old
- •Gelsolin: rapid remodeling in one or two steps
- •β4-thymosin: accounting (sometimes) for the other half
- •Dynamic actin in crawling cells
- •Actin in the leading edge
- •Monomer recycling: the other ‘actin dynamics’
- •The biophysics of actin-based pushing
- •Conclusion
- •Acknowledgements
- •References
- •10 Active cellular protrusion: continuum theories and models
- •Cellular protrusion: the standard cartoon
- •The RIF formalism
- •Mass conservation
- •Momentum conservation
- •Boundary conditions
- •Cytoskeletal theories of cellular protrusion
- •Network–membrane interactions
- •Network dynamics near the membrane
- •Special cases of network–membrane interaction: polymerization force, brownian and motor ratchets
- •Network–network interactions
- •Network dynamics with swelling
- •Other theories of protrusion
- •Numerical implementation of the RIF formalism
- •An example of cellular protrusion
- •Protrusion driven by membrane–cytoskeleton repulsion
- •Protrusion driven by cytoskeletal swelling
- •Discussion
- •Conclusions
- •References
- •11 Summary
- •References
- •Index
Cells, gels, and mechanics |
135 |
than sodium. A fuller treatment of this fundamental biological feature is given in the recent book by the author (Pollack, 2001).
Similarly, the gel construct provides an explanation for the cell potential. The cell is filled with negatively charged polymers. These polymers attract cations. The number of cations that can enter the cell (gel) is restricted by the cations’ low solubility in structured water. Those cations that do enter compete with water dipoles for the cell’s fixed anionic charges. Hence, the negative charge in the cell is not fully balanced by cations. The residual charge amounts to approximately 0.3 mol/kg (Wiggins, 1990). With net negative charge, the cytoplasm will have a net negative potential. Indeed, depending on conditions, membrane-free cells can show potentials as large as 50 mV (Collins and Edwards, 1971). And gels made of negatively charged polymers show comparable or larger negative potentials, while gels built of positively charged polymers show equivalent positive potentials (Fig. 7-4). Membranes, pumps, and channels evidently play no role.
Hence, the gel paradigm can go quite far in explaining the cell’s most fundamental attributes – distribution of ions and the presence of a cell potential. These are equilibrium processes; they require no energy for maintenance.
Cell dynamics
The cell is evidently not a static structure, but a machine designed to carry out a multitude of tasks. Such tasks are currently described by a broad variety of mechanisms, apparently lacking any single identifiable underlying theme – at least to this author. For virtually every process there appears to be another mechanism.
Whether a common underlying theme might govern the cell’s many operational tasks is a question worth asking. After all, the cell began as a simple gel and evolved from there. As it specialized, gel structure and processes gained in intricacy. Given such lineage, the potential for a simple, common, underlying, gel-based theme should not necessarily be remote. Finding a common underlying theme has been a longterm quest in other fields. In physics, for example, prot´eg´es of Einstein continue the search for a unifying force. That nature works in a parsimonious manner, employing variations of a few simple principles to carry out multitudinous actions, is an attractive notion, one I do not believe has yet been seriously pursued in the realm of cell function, although simplicity is a guiding principle in engineering.
If the cell is a gel, then a logical approach to the question of a common underlying principle of cell function is to ask whether a common underlying principle governs gel function. Gels do “function.” They undergo transition from one state to another. The process is known as a polymer-gel phase-transition – much like the transition from ice to water – a small change of environment causing a huge change in structure.
Such change can generate work. Just as ice formation has sufficient power to fracture hardened concrete, gel expansion or contraction is capable of many types of work, ranging from solute/solvent separation to force generation (Fig. 7-5). Common examples of useful phase-transitions are the time-release capsule (in which a gel-sol transition releases bioactive drugs), the disposable diaper (where a condensed gel undergoes enormous hydration and expansion to capture the “load”), and various
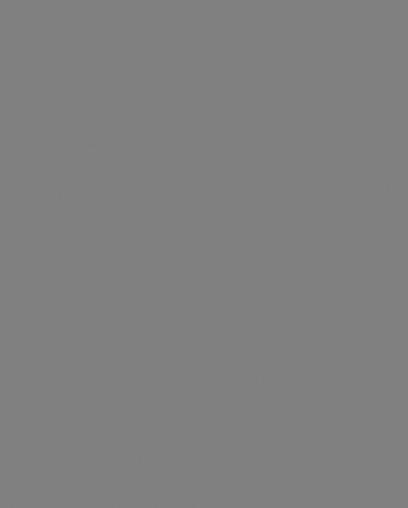
136 G.H. Pollack
Fig. 7-4. KCl-filled microelectrodes stuck into gel strips at slow constant velocity, and then withdrawn. (a) Typical anionic gel, polyacrylamide/polypotassiumacrylate, shows negative potential.
(b) Typical cationic gel, polyacrylamide/polydialyldimethylammonium chloride, shows positive potential. Courtesy of R. Gulch¨ .
artificial muscles. Such behaviors are attractive in that a large change of structure can be induced by a subtle change of environment (Fig. 7-6).
Like synthetic gels, the natural gel of the cell may have the capacity to undergo similarly useful transitions. The question is whether they do. This question is perhaps more aptly stated a bit differently, for the cell is not a homogeneous gel but a collection of gel-like organelles, each of which is assigned a specific task. The more relevant question, then, is whether any or all such organelles carry out their functions by undergoing phase-transition.
The short answer is yes: it appears that this is the case. Pursuing so extensive a theme in a meaningful way in the short space of a review article is challenging; for
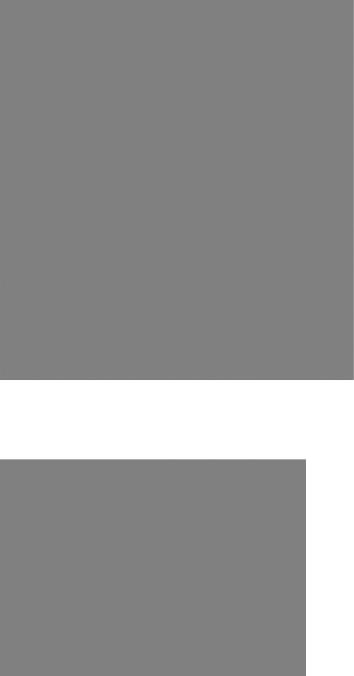
Cells, gels, and mechanics |
137 |
Fig. 7-5. Typical stimuli and responses of artificial polymer hydrogels. After Hoffman, 1991.
Temperature, solvent composition, pH, ions electric field, UV, light, specific molecules, or
chemicals
Fig. 7-6. Phase-transitions are triggered by subtle shifts of environment. After Tanaka et al., 1992.