
- •Contents
- •Contributors
- •Preface
- •1 Introduction, with the biological basis for cell mechanics
- •Introduction
- •The role of cell mechanics in biological function
- •Maintenance of cell shape
- •Cell migration
- •Mechanosensing
- •Stress responses and the role of mechanical forces in disease
- •Active cell contraction
- •Structural anatomy of a cell
- •The extracellular matrix and its attachment to cells
- •Transmission of force to the cytoskeleton and the role of the lipid bilayer
- •Intracellular structures
- •Overview
- •References
- •2 Experimental measurements of intracellular mechanics
- •Introduction
- •Forces to which cells are exposed in a biological context
- •Methods to measure intracellular rheology by macrorheology, diffusion, and sedimentation
- •Whole cell aggregates
- •Sedimentation of particles
- •Diffusion
- •Mechanical indentation of the cell surface
- •Glass microneedles
- •Cell poker
- •Atomic force microscopy
- •Mechanical tension applied to the cell membrane
- •Shearing and compression between microplates
- •Optical traps
- •Magnetic methods
- •Twisting of magnetized particles on the cell surface and interior
- •Passive microrheology
- •Optically detected individual probes
- •One-particle method
- •Two-particle methods
- •Dynamic light scattering and diffusing wave spectroscopy
- •Fluorescence correlation spectroscopy
- •Optical stretcher
- •Acoustic microscopy
- •Outstanding issues and future directions
- •References
- •3 The cytoskeleton as a soft glassy material
- •Introduction
- •Magnetic Twisting Cytometry (MTC)
- •Measurements of cell mechanics
- •The structural damping equation
- •Reduction of variables
- •Universality
- •Scaling the data
- •Collapse onto master curves
- •Theory of soft glassy rheology
- •What are soft glassy materials
- •Sollich’s theory of SGMs
- •Soft glassy rheology and structural damping
- •Open questions
- •Biological insights from SGR theory
- •Malleability of airway smooth muscle
- •Conclusion
- •References
- •4 Continuum elastic or viscoelastic models for the cell
- •Introduction
- •Purpose of continuum models
- •Principles of continuum models
- •Boundary conditions
- •Mechanical and material characteristics
- •Example of studied cell types
- •Blood cells: leukocytes and erythrocytes
- •Limitations of continuum model
- •Conclusion
- •References
- •5 Multiphasic models of cell mechanics
- •Introduction
- •Biphasic poroviscoelastic models of cell mechanics
- •Analysis of cell mechanical tests
- •Micropipette aspiration
- •Cells
- •Biphasic properties of the pericellular matrix
- •Indentation studies of cell multiphasic properties
- •Analysis of cell–matrix interactions using multiphasic models
- •Summary
- •References
- •6 Models of cytoskeletal mechanics based on tensegrity
- •Introduction
- •The cellular tensegrity model
- •The cellular tensegrity model
- •Do living cells behave as predicted by the tensegrity model?
- •Circumstantial evidence
- •Prestress-induced stiffening
- •Action at a distance
- •Do microtubules carry compression?
- •Summary
- •Examples of mathematical models of the cytoskeleton based on tensegrity
- •The cortical membrane model
- •Tensed cable nets
- •Cable-and-strut model
- •Summary
- •Tensegrity and cellular dynamics
- •Conclusion
- •Acknowledgement
- •References
- •7 Cells, gels, and mechanics
- •Introduction
- •Problems with the aqueous-solution-based paradigm
- •Cells as gels
- •Cell dynamics
- •Gels and motion
- •Secretion
- •Muscle contraction
- •Conclusion
- •Acknowledgement
- •References
- •8 Polymer-based models of cytoskeletal networks
- •Introduction
- •The worm-like chain model
- •Force-extension of single chains
- •Dynamics of single chains
- •Network elasticity
- •Nonlinear response
- •Discussion
- •References
- •9 Cell dynamics and the actin cytoskeleton
- •Introduction: The role of actin in the cell
- •Interaction of the cell cytoskeleton with the outside environment
- •The role of cytoskeletal structure
- •Actin mechanics
- •Actin dynamics
- •The emergence of actin dynamics
- •The intrinsic dynamics of actin
- •Regulation of dynamics by actin-binding proteins
- •Capping protein: ‘decommissioning’ the old
- •Gelsolin: rapid remodeling in one or two steps
- •β4-thymosin: accounting (sometimes) for the other half
- •Dynamic actin in crawling cells
- •Actin in the leading edge
- •Monomer recycling: the other ‘actin dynamics’
- •The biophysics of actin-based pushing
- •Conclusion
- •Acknowledgements
- •References
- •10 Active cellular protrusion: continuum theories and models
- •Cellular protrusion: the standard cartoon
- •The RIF formalism
- •Mass conservation
- •Momentum conservation
- •Boundary conditions
- •Cytoskeletal theories of cellular protrusion
- •Network–membrane interactions
- •Network dynamics near the membrane
- •Special cases of network–membrane interaction: polymerization force, brownian and motor ratchets
- •Network–network interactions
- •Network dynamics with swelling
- •Other theories of protrusion
- •Numerical implementation of the RIF formalism
- •An example of cellular protrusion
- •Protrusion driven by membrane–cytoskeleton repulsion
- •Protrusion driven by cytoskeletal swelling
- •Discussion
- •Conclusions
- •References
- •11 Summary
- •References
- •Index
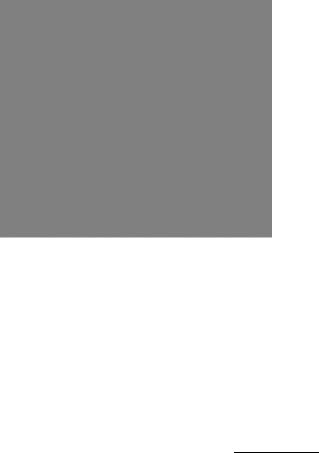
Experimental measurements of intracellular mechanics |
39 |
Fig. 2-12. Sketch of 1-particle and 2-particle microrheology using lasers for trapping and detection. Either one laser beam is focused on one particle at a time, or the two beams, displaced by some distance, are each focused on seperate particles. In both cases the motion of the particle in the two directions normal to the laser propagation direction is measured by projecting the laser light onto quadrant photodiodes downstream from the sample.
A Kramers-Kronig integral can again be used to calculate the real parts, and elastic moduli can be derived according to Levine and Lubensky (2002) from:
|
|
|
α||12(ω) = |
1 |
|
|
(2.18) |
||
|
|
|
4π r µ0(ω) |
||||||
α12 |
(ω) |
= |
1 |
|
|
λ0(ω) + 3µ0(ω) |
, |
(2.19) |
|
|
|
|
|||||||
|
|
8π r µ0(ω) λ0(ω) + 2µ0(ω) |
|
written here (following Levine and Lubensky (2002)) with the Lam´e coefficients λ0(ω) and µ0(ω), where µ0(ω) = G(ω). One can thus measure directly the compressional modulus and the shear modulus in the sample.
The technique can again be implemented using video recording and particle tracking (Crocker et al., 2000) or laser interferometry. In cells, so far only a video-based variant has been used (Lau et al., 2003), exploring the low-frequency regime of the cellular dynamics in mouse macrophages and mouse carcinoma cells. It was found that the low-frequency passive microrheology results were strongly influenced by active transport in the cells, so that the fluctuation-dissipation theorem could not be used for calculating viscoelastic parameters.
Dynamic light scattering and diffusing wave spectroscopy
A well-established method to study the dynamics of large ensembles of particles in solutions is dynamic light scattering (DLS) (Berne and Pecora, 1990). To obtain smooth data and good statistics, it is obviously advantageous to average over a large number of particles. In DLS, a collimated laser beam is typically sent through a sample of milliliter volume, and scattered light is collected under a well-defined

40 P. Janmey and C. Schmidt
angle with a photomultiplier or other sensitive detector. The intensity autocorrelation function:
g |
(τ ) |
|
|
I (t )I (t + τ ) |
|
1 |
|
βe−q2 |
|
r 2(τ ) |
/3 |
(2.20) |
|
= |
|
= |
+ |
|
|
|
|||||||
2 |
|
|
I (t ) |
2 |
|
|
|
|
|||||
|
|
|
|
|
|
|
|
|
|
|
|
|
can be used to calculate the average mean square displacement r 2(τ ) of particles, with the scattering vector q = 4π n sin(θ /2)/λ, wavelength λ, scattering angle θ and index of the solvent n, and a coherence factor β. This relationship assumes that all particles are identical and that the solution is homogeneous across the scattering volume. It also assumes that the particles dominate the scattering intensity compared to the scattering from the embedding medium itself. DLS has also been used extensively to study polymer solutions without added probe particles (Berne and Pecora, 1990). In that case the medium has to be modeled to extract material properties from the observed intensity autocorrelation function. This has been done for example for pure actin networks as models for the cytoskeleton of cells (Isambert et al., 1995; Liverpool and Maggs, 2001; Schmidt et al., 1989). Unfortunately this technique is not well applicable to study the interior of cells, because the cellular environment is highly inhomogeneous and it is not well defined which structures scatter the light in any given location. Larger objects dominate the scattered intensity (Berne and Pecora, 1990). DLS has been applied to red blood cells (Peetermans et al., 1987a; Peetermans et al., 1987b), but results have been qualitative. It is also difficult to introduce external probe particles that scatter light strongly enough in sufficient concentrations without harming the cells.
A related light-scattering technique is diffusing wave spectroscopy (DWS) (Pine et al., 1988; Weitz et al., 1993), which measures again intensity correlation functions of scattered light, but now in very dense opaque media where light is scattered many times before it is detected so that the path of a photon becomes a random walk and resembles diffusion. There is no more scattering-vector dependence in the field correlation function, which is directly related to the average mean square displacementr 2(τ ) of the scattering particles (Weitz and Pine, 1993):
|
∞ |
|
|
2 |
|
2 |
(τ ) |
|
|
g1(τ ) |
|
P (s) exp |
− |
k0 s r |
|
ds. |
(2.21) |
||
|
|
|
|
3l |
|
|
|
||
|
0 |
|
|
|
|
|
|
|
|
P (s) is the probability that the light travels a path length s, k0 = 2π/λ is the wave vector, and l is the transport mean free path. The final steps to extract a complex shear modulus are the same as described above, either using the power spectral density method (Schnurr et al., 1997) or the Laplace transform method (Mason et al., 1997).
The advantage of the technique is that it is sensitive to very small motions (of less than nm) because the path of an individual photon reflects the sum of the motions of the all particles by which it is scattered. The bandwidth of the technique is also high (typically 10 Hz to 1 MHz), so that ensemble-averaged mean-square displacements, and from that viscoelastic response functions, can be measured over many decades in frequency. The technique has been used to study polymer solutions, colloidal systems, and cytoskeletal protein solutions (actin) (Mason et al., 1997; Mason et al., 2000) and
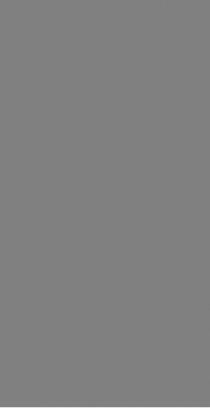
Experimental measurements of intracellular mechanics |
41 |
Fig. 2-13. (a) Experimental set-up for fluorescence correlation spectroscopy. A laser beam is expanded (L1, L2) and focussed through a microscope objective into a fluorescent sample. The fluorescence light is collected through the same objective and split out with a dichroic mirror toward the confocal pinhole
(P) and then the detector. (b) Magnified focal volume with the fluorescent particles (spheres) and the diffusive path of one particle highlighted. From Hess et al., 2002.
results agree with those from conventional methods in the time/frequency regimes where they overlap. The application to cells is hindered, just as in the case of DLS, by the inhomogeneity of the cellular environment. Furthermore, typical cells are more or less transparent, in other words one would need to introduce high concentrations of scattering particles, which likely would disturb the cell’s integrity.
Fluorescence correlation spectroscopy
Many complications can be avoided if specific particles or molecules of interest in a cell can be selected from other structures. A way to avoid collecting signals from unknown cellular structures is to use fluorescent labeling of particular molecules or structures within the cell. This method is extensively used in cell biology to study the localization of certain proteins in the cell. Fluorescence can also be used to measure dynamic processes in video microscopy, but due to low emission intensities of fluorescent molecules and due to their fast Brownian motion when they are not fixed to larger structures, it is difficult to use such data to extract diffusion coefficients or viscoelastic parameters inside cells. A method that is related to dynamic light scattering and is a nonimaging method that can reach much faster time scales is fluorescence correlation spectroscopy (Hess et al., 2002; Webb, 2001), where a laser is focused to a small volume and the fluctuating fluorescence originating from molecules entering and leaving this volume is recorded with fast detectors (Fig. 2-13).
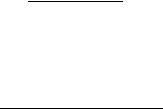
42 P. Janmey and C. Schmidt
From the fluorescence intensity fluctuations δF (t ) = F (t ) − F (t ) one calculates the normalized autocorrelation function:
G(τ ) |
= |
|
δ F (t )δ F (t + τ ) |
, |
(2.22) |
|
F (t ) 2 |
||||||
|
|
|
|
from which one can calculate in the simplest case, in the absence of chemical reactions involving the fluorescent species, the characteristic time τ D a diffusing molecule spends in the focal volume:
G D (τ ) = |
1 |
(2.23) |
N (1 + τ /τD ) (1 + τ /ω2τD )1/2 |
with an axial-to-lateral-dimension ratio ω and the mean number of fluorescent molecules in the focus N. Eq. 2.23 is valid for a molecule diffusing in 3D. The method can also be used in other cases, for example 2D diffusion in a membrane.
With some knowledge of the geometry of the situation – for example 2D membranebound diffusion – one can again extract diffusion coefficients. This has been done on cell surfaces and even inside cells (see references in Hess et al., (2002)). Data typically have been interpreted as diffusion in a purely viscous environment or as diffusion in an inhomogeneous environment with obstacles. Compared with the rheology methods described above, fluorescence correlation spectroscopy is particularly good when studying small particles such as single-enzyme molecules. For the motion of such particles it is not appropriate to model the environment inside a cell as a viscoelastic continuum, because characteristic length scales of the cytoskeleton are as large or larger than the particles.
Optical stretcher
A novel optical method related to optical traps employs two opposing nonfocused laser beams to both immobilize and stretch a suspended cell (Guck et al., 2001; Guck et al., 2000). Viscoelastic properties are determined from the time-dependent change in cell dimensions as a function of optical pressures. This method has the significant advantage over other optical trapping methods that it can be scaled up and automated to allow measurement, and potentially sorting, of many cells within a complex mixture for use in diagnosing abnormal cells and sorting cells on the basis of their rigidity (Lincoln et al., 2004).
Acoustic microscopy
Ultrasound transmission and attenuation through cells and biological tissues can also provide measurements of viscoelasticity, and acoustic microscopy has the potential to provide high-resolution imaging of live cells in a minimally invasive manner (Viola and Walker, 2003). Studies of purified systems such as F-actin (Wagner et al., 2001; Wagner et al., 1999), and alginate capsules (Klemenz et al., 2003), suggest that acoustic signals can be related to changes in material properties of these biopolymer gels, but there are numerous challenges related to interpreting the data and relating them