
- •Contents
- •Preface
- •How to use this book
- •Chapter 1 Units, constants, and conversions
- •1.1 Introduction
- •1.2 SI units
- •1.3 Physical constants
- •1.4 Converting between units
- •1.5 Dimensions
- •1.6 Miscellaneous
- •Chapter 2 Mathematics
- •2.1 Notation
- •2.2 Vectors and matrices
- •2.3 Series, summations, and progressions
- •2.5 Trigonometric and hyperbolic formulas
- •2.6 Mensuration
- •2.8 Integration
- •2.9 Special functions and polynomials
- •2.12 Laplace transforms
- •2.13 Probability and statistics
- •2.14 Numerical methods
- •Chapter 3 Dynamics and mechanics
- •3.1 Introduction
- •3.3 Gravitation
- •3.5 Rigid body dynamics
- •3.7 Generalised dynamics
- •3.8 Elasticity
- •Chapter 4 Quantum physics
- •4.1 Introduction
- •4.3 Wave mechanics
- •4.4 Hydrogenic atoms
- •4.5 Angular momentum
- •4.6 Perturbation theory
- •4.7 High energy and nuclear physics
- •Chapter 5 Thermodynamics
- •5.1 Introduction
- •5.2 Classical thermodynamics
- •5.3 Gas laws
- •5.5 Statistical thermodynamics
- •5.7 Radiation processes
- •Chapter 6 Solid state physics
- •6.1 Introduction
- •6.2 Periodic table
- •6.4 Lattice dynamics
- •6.5 Electrons in solids
- •Chapter 7 Electromagnetism
- •7.1 Introduction
- •7.4 Fields associated with media
- •7.5 Force, torque, and energy
- •7.6 LCR circuits
- •7.7 Transmission lines and waveguides
- •7.8 Waves in and out of media
- •7.9 Plasma physics
- •Chapter 8 Optics
- •8.1 Introduction
- •8.5 Geometrical optics
- •8.6 Polarisation
- •8.7 Coherence (scalar theory)
- •8.8 Line radiation
- •Chapter 9 Astrophysics
- •9.1 Introduction
- •9.3 Coordinate transformations (astronomical)
- •9.4 Observational astrophysics
- •9.5 Stellar evolution
- •9.6 Cosmology
- •Index
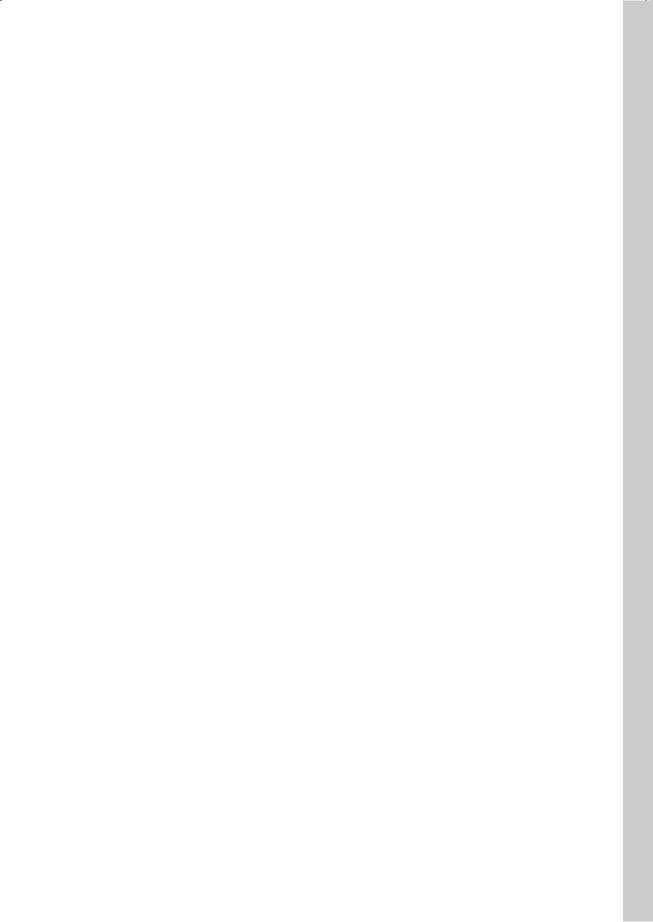
Chapter 9 Astrophysics
9.1Introduction
Many of the formulas associated with astronomy and astrophysics are either too specialised for a general work such as this or are common to other fields and can therefore be found elsewhere in this book. The following section includes many of the relationships that fall into neither of these categories, including equations to convert between various astronomical coordinate systems and some basic formulas associated with cosmology.
Exceptionally, this section also includes data on the Sun, Earth, Moon, and planets. Observational astrophysics remains a largely inexact science, and parameters of these (and other) bodies are often used as approximate base units in measurements. For example, the masses of stars and galaxies are frequently quoted as multiples of the mass of the Sun (1M = 1.989 × 1030 kg), extra-solar system planets in terms of the mass of Jupiter, and so on. Astronomers seem to find it particularly di cult to drop arcane units and conventions, resulting in a profusion of measures and nomenclatures throughout the subject. However, the convention of using suitable astronomical objects in this way is both useful and widely accepted.
9
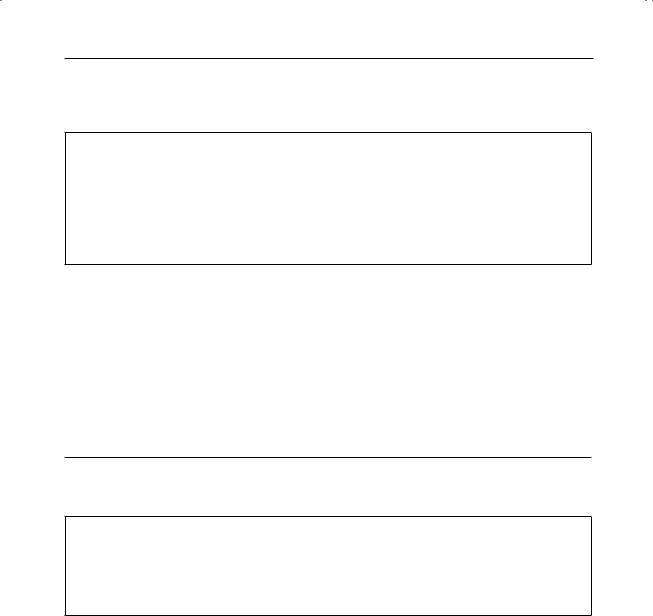
176 Astrophysics
9.2 Solar system data Solar data
equatorial radius |
R |
|
= |
6 |
. |
960 |
× |
10 |
8 m |
|
|
= |
109.1R |
|
|
|
|
|
|
|
|||||||||||
mass |
|
|
= |
|
|
30 |
kg |
= |
|
|
|
|
10 |
5 |
M |
|
|||||||||||||||
M |
|
1.9891 |
× |
10 |
|
|
3.32946 |
× |
|
|
|||||||||||||||||||||
|
|
|
|
|
|
|
|
|
|
|
|
|
|
|
|
|
|
|
|
|
|
|
|
|
|
||||||
polar moment of inertia |
I |
|
= |
5.7 |
× |
10 |
46 |
kgm |
2 |
= |
7.09 |
× |
10 |
8 |
I |
|
|
|
|||||||||||||
|
|
|
|
|
|
|
|
||||||||||||||||||||||||
bolometric luminosity |
|
|
= |
|
|
|
× 10 |
26 |
W |
|
|
|
|
|
|
|
|
|
|
||||||||||||
L |
3.826 |
|
|
|
|
|
|
|
|
|
|
|
|
|
|
||||||||||||||||
e ective surface temperature |
T |
= |
5770K |
|
|
|
|
|
|
|
|
|
|
|
|
|
|
|
|
|
|
|
|
||||||||
solar constanta |
|
|
|
|
|
1.368 |
× 103 Wm−2 |
|
|
|
|
|
|
|
|
|
|
|
|
||||||||||||
absolute magnitude |
MV |
= |
+4.83; |
|
|
|
|
|
|
|
|
Mbol |
= |
+4.75 |
|
|
|
|
|
|
|
|
|
||||||||
apparent magnitude |
mV |
= |
−26.74; |
|
|
|
|
|
|
mbol |
= |
−26.82 |
|
|
|
|
|
|
|
aBolometric flux at a distance of 1 astronomical unit (AU).
Earth data
equatorial radius |
|
R |
|
= |
6.37814 |
× |
106 m |
|
|
= |
9.166 |
× |
10−3R |
|
|||||||||||||||
flattening |
a |
|
|
|
= |
|
|
|
|
|
|
|
|
|
|
|
= |
|
|
|
|
|
|
||||||
|
|
f |
|
0.00335364 |
|
|
|
|
|
1/298.183 |
|
|
|
||||||||||||||||
mass |
|
|
M |
|
= |
5.9742 |
× |
1024 kg |
|
= |
3.0035 |
|
10−6M |
|
|||||||||||||||
polar moment of inertia |
I |
|
= |
8.037 |
|
|
37 |
kgm |
2 |
= |
1.41 |
|
|
|
× 9 |
I |
|
||||||||||||
|
|
× |
10 |
|
|
|
× |
10− |
|
|
|||||||||||||||||||
orbital semi-major axis |
b |
|
|
= |
|
|
|
|
|
|
|
11 |
m |
= |
|
|
|
|
|
|
|||||||||
|
1AU |
1.495979 |
|
4 |
10 |
|
214.9R |
|
|
|
|
||||||||||||||||||
|
|
|
|
|
|
|
|
|
|
|
|
|
|
|
|
|
1 |
|
|
|
|
|
|
|
|
|
|||
mean orbital velocity |
|
|
|
|
|
|
× |
|
|
× |
ms− |
|
|
|
|
|
|
|
|
|
|
|
|
||||||
|
|
|
|
|
2.979 |
10 |
|
|
|
|
|
|
|
|
|
|
|
|
|
||||||||||
equatorial surface gravity |
ge |
|
= |
|
|
|
|
|
|
|
2 |
|
|
|
(includes rotation) |
|
|||||||||||||
|
9.780327ms− |
|
|
|
|
|
|||||||||||||||||||||||
polar surface gravity |
|
gp |
|
= |
9.832186ms−2 |
|
|
|
|
|
|
|
|
|
|
|
|
|
|||||||||||
rotational angular velocity |
ωe |
= |
7.292115 |
× |
10−5 rads−1 |
|
|
|
|
|
|
|
|
|
|||||||||||||||
a |
|
|
|
|
|
|
|
|
|
|
|
|
|
6 |
|
|
|
|
|
|
|
|
|
|
|
|
|
||
bf equals (R − Rpolar)/R . The mean radius of the Earth is 6.3710 × 10 |
|
m. |
|
|
|
|
|
|
|
|
|
|
|
||||||||||||||||
About the Sun. |
|
|
|
|
|
|
|
|
|
|
|
|
|
|
|
|
|
|
|
|
|
|
|
|
|
|
|
|
Moon data
equatorial radius |
|
Rm |
= |
|
|
|
|
6 |
|
|
|
= |
0.27240R |
|
|
|
||||
|
1.7374 × 1022m |
2 |
|
|
||||||||||||||||
mass |
|
Mm |
= |
7.3483 |
× |
10 |
kg |
= |
1.230 |
× |
10− |
M |
|
|||||||
mean orbital radius |
a |
a |
|
= |
|
|
10 |
8 |
m |
= |
|
|
|
|
||||||
|
m |
3.84400 |
× |
|
60.27R |
|
|
|
|
|||||||||||
mean orbital velocity |
|
|
1.03 × 10 |
3 |
ms− |
1 |
|
|
|
|
|
|
||||||||
|
|
|
|
|
|
|
|
|
|
|
|
|
||||||||
orbital period (sidereal) |
|
|
|
27.32166d |
|
|
|
|
|
|
|
|
|
|
|
|||||
equatorial surface gravity |
|
|
|
1.62ms−2 |
|
|
|
|
= |
0.166ge |
|
|
|
|
aAbout the Earth.
Planetary dataa
|
M/M |
R/R |
T (d) |
|
P (yr) |
|
a(AU) |
M |
mass |
||
Mercury |
0.055 |
274 |
0.382 |
51 |
58.646 |
0.240 |
85 |
0.387 10 |
R |
equatorial radius |
|
Venusb |
0.815 |
00 |
0.948 |
83 |
243.018 |
0.615 |
228 |
0.723 35 |
T |
rotational period |
|
Earth |
1 |
|
1 |
|
0.997 |
27 |
1.000 |
04 |
1.000 00 |
P |
orbital period |
Mars |
0.107 |
45 |
0.532 |
60 |
1.025 |
96 |
1.880 |
93 |
1.523 71 |
a |
mean distance |
Jupiter |
317.85 |
11.209 |
0.413 |
54 |
11.861 3 |
5.202 53 |
|
|
|||
M |
5.9742 × 1024 kg |
||||||||||
Saturn b |
95.159 |
9.449 |
1 |
0.444 |
01 |
29.628 2 |
9.575 60 |
R |
6.37814 × 106 m |
||
Uranus |
14.500 |
4.007 |
3 |
0.718 |
33 |
84.746 6 |
19.293 4 |
1d |
86400s |
||
Neptune |
17.204 |
3.882 |
6 |
0.671 |
25 |
166.344 |
30.245 9 |
1yr |
3.15569 × 107 s |
||
Plutob |
0.00251 |
0.187 |
36 |
6.387 |
2 |
248.348 |
39.509 0 |
1AU |
1.495979 × 1011 m |
aUsing the osculating orbital elements for 1998. Note that P is the instantaneous orbital period, calculated from the planet’s daily motion. The radii of gas giants are taken at 1 atmosphere pressure.
bRetrograde rotation.
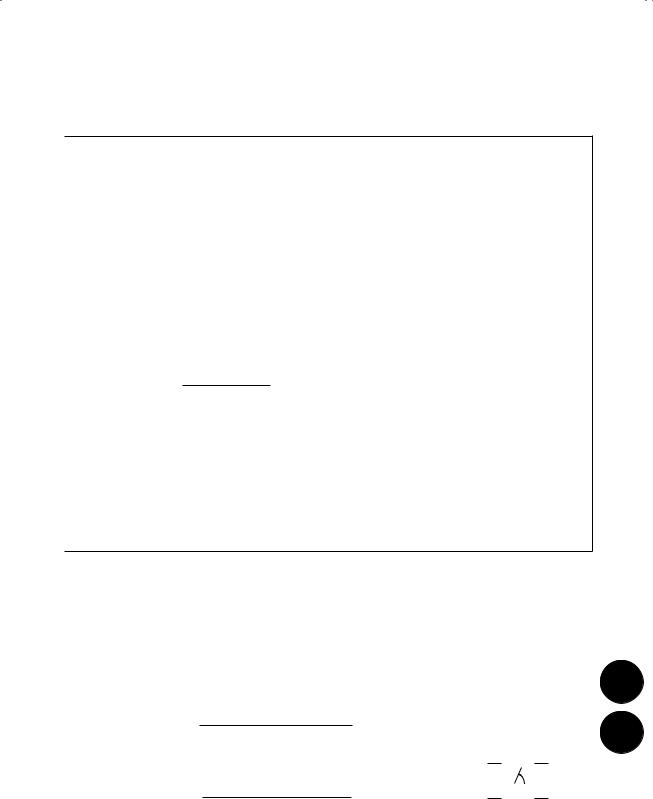
9.3 Coordinate transformations (astronomical) |
177 |
|
|
9.3 Coordinate transformations (astronomical)
Time in astronomy
|
Julian day numbera |
|
|
|
||||
|
|
|
|
|||||
|
JD =D − 32075 + 1461 (Y + 4800 + (M − 14)/12)/4 |
|
||||||
|
+ 367 (M − 2 − (M − 14)/12 12)/12 |
|
||||||
|
− 3 ((Y + 4900 + (M − 14)/12)/100)/4 |
(9.1) |
||||||
|
Modified |
MJD = JD − 2400000.5 |
|
|||||
|
Julian day |
(9.2) |
||||||
|
number |
|
|
|
|
|
|
|
|
|
|
|
|
|
|
|
|
|
Day of |
W = (JD + 1) mod 7 |
(9.3) |
|||||
|
week |
|||||||
|
|
|
|
|
|
|
|
|
|
|
|
|
|
|
|
|
|
|
Local civil |
LCT = UTC + TZC + DSC |
(9.4) |
|||||
|
time |
|||||||
|
|
|
|
|
|
|
|
|
|
|
|
|
|
|
|
|
|
|
Julian |
T = |
JD − 2451545.5 |
|
|
(9.5) |
||
|
centuries |
|
|
|||||
|
36525 |
|
|
|
|
|
||
|
|
|
|
|
|
|||
|
|
GMST =6h41m50s.54841 |
|
|||||
|
Greenwich |
+ 8640184 |
s |
.812866T |
|
|||
|
sidereal |
|
|
|||||
|
|
+ 0s.093104T 2 |
|
|||||
|
time |
|
|
|||||
|
|
− 0s.0000062T 3 |
(9.6) |
|||||
|
|
|
||||||
|
|
|
|
|
|
|
|
|
|
Local |
|
|
λ◦ |
|
|
|
|
|
sidereal |
LST = GMST + |
|
|
|
(9.7) |
||
|
|
|
||||||
|
time |
|
15◦ |
|
|
|
JD Julian day number
Dday of month number
Ycalendar year, e.g., 1963
Mcalendar month (Jan=1)
integer multiply
/integer divide
MJD modified Julian day number
Wday of week (0=Sunday, 1=Monday, ... )
LCT |
local civil time |
UTC |
coordinated universal time |
TZC |
time zone correction |
DSC |
daylight saving correction |
TJulian centuries between 12h UTC 1 Jan 2000 and 0h UTC D/M/Y
GMST Greenwich mean sidereal time at 0h UTC D/M/Y (for later times use
1s = 1.002738 sidereal seconds)
LST |
local sidereal time |
λ◦ |
geographic longitude, |
|
degrees east of Greenwich |
aFor the Julian day starting at noon on the calendar day in question. The routine is designed around integer arithmetic with “truncation towards zero” (so that −5/3 = −1) and is valid for dates from the onset of the Gregorian calendar, 15 October 1582. JD represents the number of days since Greenwich mean noon 1 Jan 4713 BC. For reference, noon, 1 Jan 2000 = JD2451545 and was a Saturday (W = 6).
Horizon coordinatesa
Hour angle |
H = LST − α |
|
|
|
|
(9.8) |
LST |
local sidereal time |
|
|
||||||
|
|
|
|
H |
(local) hour angle |
|
|
|||||||||
|
|
|
|
|
|
|
|
|
α |
right ascension |
|
|
||||
|
sina = sinδ sinφ+ cosδ cosφcosH |
(9.9) |
|
|
||||||||||||
|
9 |
|||||||||||||||
Equatorial |
δ |
declination |
|
|||||||||||||
|
|
− |
cosδ sinH |
|
a |
altitude |
|
|
|
|||||||
to horizon |
tanA |
|
(9.10) |
|
|
|
||||||||||
|
|
|
|
|
A |
|
|
|
|
|
|
|
||||
|
|
≡ sinδ cosφ |
− |
sinφcosδ cosH |
|
|
azimuth (E from N) |
|
|
|||||||
|
|
|
|
|
||||||||||||
|
|
|
|
|
|
|
φ |
observer’s latitude |
|
|
||||||
|
sinδ = sinasinφ+ cosacosφcosA |
(9.11) |
|
+ |
|
+ |
|
|
||||||||
Horizon to |
|
|
− |
|
+ |
|
|
|||||||||
|
|
− cosasinA |
|
|
|
|
|
A, H |
|
|
|
|||||
equatorial |
tanH |
|
(9.12) |
|
|
− |
|
− |
|
|
||||||
|
|
≡ sinacosφ |
− |
sinφcosacosA |
|
|
|
− |
|
+ |
|
|
||||
|
|
|
|
|
|
|
|
|
|
|
|
|
|
|
aConversions between horizon or alt–azimuth coordinates, (a,A), and celestial equatorial coordinates, (δ,α). There are a number of conventions for defining azimuth. For example, it is sometimes taken as the angle west from south rather than east from north. The quadrants for A and H can be obtained from the signs of the numerators and denominators in Equations (9.10) and (9.12) (see diagram).
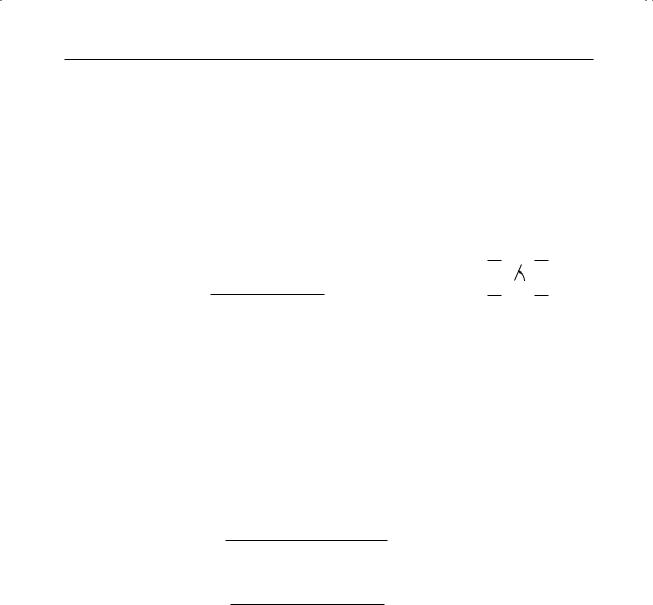
178 Astrophysics
Ecliptic coordinatesa
|
ε = 23◦26 21 .45 |
− |
46 .815T |
|
ε |
mean ecliptic obliquity |
||||||||
Obliquity of |
|
|
|
0 .0006T 2 |
|
|||||||||
|
|
|
− |
|
T |
Julian |
centuries since |
|||||||
the ecliptic |
|
|
|
|
||||||||||
|
|
|
|
|
|
|
|
b |
||||||
|
|
|
|
+ 0 .00181T 3 |
(9.13) |
|
J2000.0 |
|
|
|
||||
|
|
|
|
|
|
|
|
|
|
|
||||
|
sinβ = sinδ cosε− cosδ sinεsinα |
(9.14) |
α |
right ascension |
||||||||||
Equatorial to |
δ |
declination |
||||||||||||
ecliptic |
tanλ ≡ |
sinαcosε+ tanδ sinε |
|
(9.15) |
λ |
ecliptic longitude |
||||||||
|
cosα |
β |
ecliptic latitude |
|||||||||||
|
|
|
|
|
|
|
|
|
+ |
|
|
+ |
||
|
sinδ = sinβ cosε+ cosβ sinεsinλ |
(9.16) |
|
|
|
|||||||||
Ecliptic to |
|
|
− |
|
|
λ, α+ |
||||||||
equatorial |
|
|
sinλcosε− tanβ sinε |
|
|
|
|
|
|
|
|
|
||
tanα |
≡ |
|
(9.17) |
|
|
− |
|
|
− |
|||||
|
|
cosλ |
|
|
|
− |
|
|
+ |
|||||
|
|
|
|
|
|
|
|
|
aConversions between ecliptic, (β,λ), and celestial equatorial, (δ,α), coordinates. β is positive above the ecliptic and λ increases eastwards. The quadrants for λ and α can be obtained from the signs of the numerators and denominators in Equations (9.15) and (9.17) (see diagram).
bSee Equation (9.5).
Galactic coordinatesa
Galactic |
αg = 192◦15 |
|
|
|
|
|
|
|
(9.18) |
αg |
right ascension of |
||||||
δg = 27◦24 |
|
|
|
|
|
|
|
|
(9.19) |
|
north galactic pole |
||||||
frame |
|
|
|
|
|
|
|
|
δg |
declination of north |
|||||||
lg = 33◦ |
|
|
|
|
|
|
|
|
|
(9.20) |
|||||||
|
|
|
|
|
|
|
|
|
|
|
galactic pole |
||||||
|
|
|
|
|
|
|
|||||||||||
Equatorial |
sinb = cosδ cosδg cos(α− αg) + sinδ sinδg |
(9.21) |
lg |
ascending node of |
|||||||||||||
to galactic |
|
− |
|
|
≡ |
tanδ cosδg − cos(α− αg)sinδg |
|
|
galactic plane on |
||||||||
tan(l |
lg) |
(9.22) |
|
equator |
|||||||||||||
|
|
|
|
sin(α |
− |
αg) |
|
|
|
|
|
||||||
|
sinδ = cosbcosδg sin(l − lg) + sinbsinδg |
(9.23) |
δ |
declination |
|||||||||||||
Galactic to |
α |
right ascension |
|||||||||||||||
equatorial |
tan(α |
− |
αg) |
|
cos(l − lg) |
|
lg) |
(9.24) |
b |
galactic latitude |
|||||||
|
|
|
|
|
≡ tanbcosδg |
− |
sinδg sin(l |
− |
|
l |
galactic longitude |
||||||
|
|
|
|
|
|
|
|
|
|
|
|
|
|
|
|
aConversions between galactic, (b,l), and celestial equatorial, (δ,α), coordinates. The galactic frame is defined at epoch B1950.0. The quadrants of l and α can be obtained from the signs of the numerators and denominators in Equations (9.22) and (9.24).
Precession of equinoxesa
|
|
|
|
|
|
|
|
α |
right ascension of date |
|
In right |
|
|
s |
|
s |
|
|
α0 |
right ascension at J2000.0 |
|
ascension |
α |
α0 + (3 |
.075 + 1 |
|
.336sinα0 tanδ0)N |
(9.25) |
N |
number of years since |
||
|
|
|
|
|
|
|
|
|
J2000.0 |
|
|
|
|
|
|
|
|
|
|
|
|
In |
δ |
|
δ0 + (20 .043cosα0)N |
(9.26) |
δ |
declination of date |
||||
declination |
δ0 |
declination at J2000.0 |
||||||||
|
|
|
|
|
|
|||||
|
|
|
|
|
|
|
|
|
|
aRight ascension in hours, minutes, and seconds; declination in degrees, arcminutes, and arcseconds. These equations are valid for several hundred years each side of J2000.0.