
Molecular Fluorescence
.pdf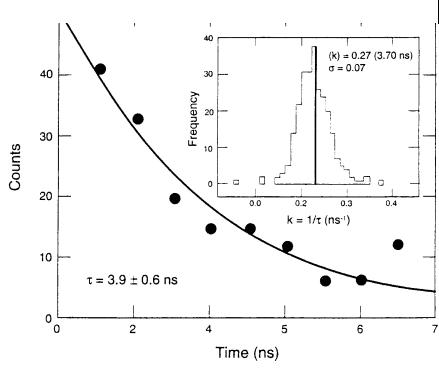
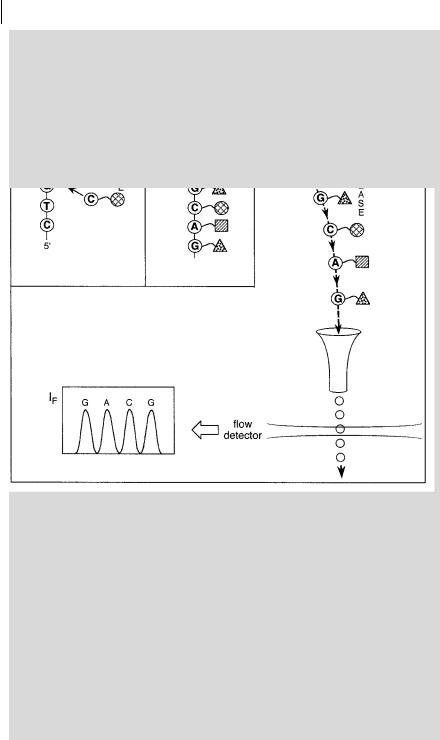
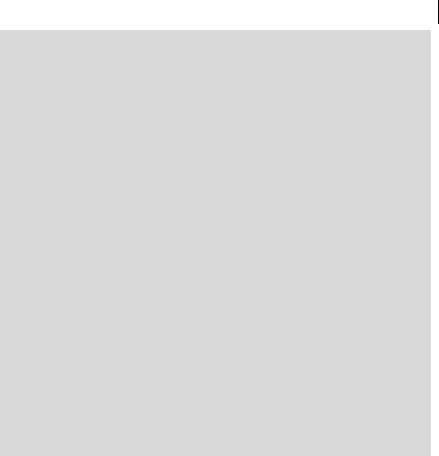
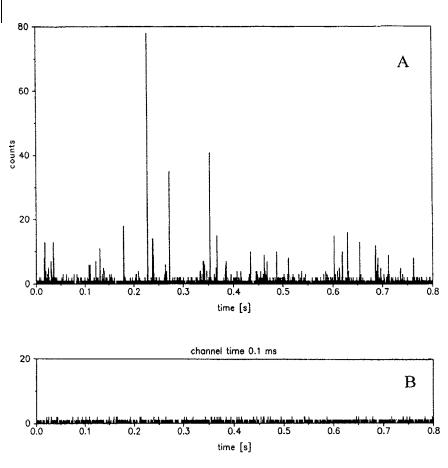
378 11 Advanced techniques in fluorescence spectroscopy
Fig. 11.16. Detection of single molecules of Rhodamine 6G by confocal fluorescence microscopy. A: solution of Rhodamine 6G 2 10 12 M in water; B: pure water (reproduced with permission from Mets and Rigler, 1994, J. Fluorescence 4, 259).
samples where coverage can be controlled. In fact, the advantages of these techniques are the enhanced signal-to-noise ratio and the higher speed of data acquisition.
11.5
Bibliography
Ambrose W. P., Goodwin P. M., Jett J. H., |
Bashe´ T., Moerner W. E., |
Orrit M. and |
Van Horden A., Werner J. H. and Keller |
Wild U. P. (Eds) (1996) |
Single-Molecule |
R. A. (1999) Single Molecule Fluorescence |
Optical Detection, Imaging and Spectroscopy, |
|
Spectroscopy at Ambient Temperature, |
VCH, Weinheim. |
|
Chem. Rev. 99, 2929–56. |
Buehler C., Stoeckli K. and Auer M. (2001) |


380 11 Advanced techniques in fluorescence spectroscopy
Molecules as Observed by Fluorescence |
1: Techniques, Plenum Press, New York, pp. |
Correlation Spectroscopy, in: Wolfbeis O. |
337–78. |
S. (Ed.), Fluorescence Spectroscopy. New |
Wang X. F., Periasamy A. and Herman B. |
Methods and Applications, Springer-Verlag, |
(1992) Fluorescence Lifetime Imaging |
Berlin, pp. 15–24. |
Microscopy (FLIM): Instrumentation and |
So P. T. C., French T., Yu W. M., Berland K. |
Applications, Crit. Rev. Anal. Chem. 23, 369– |
M., Dong C. Y. and Gratton E. (1996) |
95. |
Two-Photon Fluorescence Microscopy: |
Wang X. F., Periasamy A., Wodnicki P., |
Time-Resolved and Intensity Imaging, in: |
Gordon G. W. and Herman B. (1996) |
Wang X. F. and Herman B. (Eds), |
Time-Resolved Fluorescence Lifetime |
Fluorescence Imaging Spectroscopy and |
Imaging Microscopy: Instrumentation and |
Microscopy, Chemical Analysis Series, Vol. |
Biomedical Applications, in: Wang X. F. |
137, John Wiley & Sons, New York, pp. |
and Herman B. (Eds), Fluorescence Imaging |
351–74. |
Spectroscopy and Microscopy, Chemical |
Tan W. and Kopelman R. (1996) Nanoscale |
Analysis Series, Vol. 137, John Wiley & |
Imaging and Sensing by Near-Field Optics, |
Sons, New York, pp. 313–50. |
in: Wang X. F. and Herman B. (Eds), |
Webb W. W. (1976) Applications of |
Fluorescence Imaging Spectroscopy and |
Fluorescence Correlation Spectroscopy, |
Microscopy, Chemical Analysis Series, Vol. |
Quart. Rev. Biophys. 9, 49–68. |
137, John Wiley & Sons, New York, pp. |
¨ |
Widengren J., Mets U. and Rigler R. (1995) |
|
407–75. |
Fluorescence Correlation Spectroscopy of |
Thompson N. L. (1991) Fluorescence |
Triplet States in Solution: A Theoretical and |
Correlation Spectroscopy, in: Lakowicz J. R. |
Experimental Study, J. Phys. Chem. 99, |
(Ed.), Topics in Fluorescence Spectroscopy, Vol. |
13368–79. |
