
Molecular Fluorescence
.pdf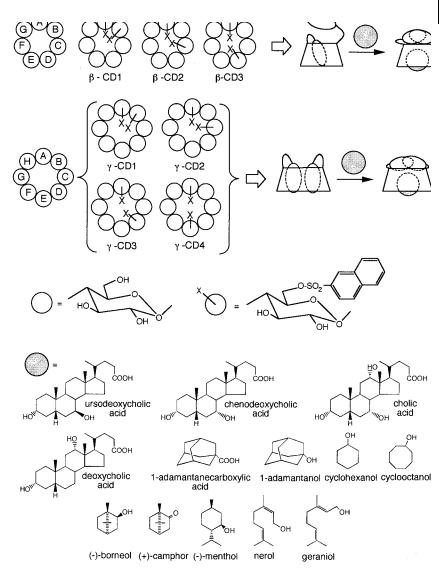
10.5 Fluorescent molecular sensors of neutral molecules and surfactants 325
Fig. 10.38. Modified b- and g-cyclodextrins with two appended 2-naphthylsulfonyl fluorophores (adapted from Minato S. et al. (1991) Photochem. Photobiol. 54, 593).
guest molecule (like those presented in Figure 10.38), exclusion of the fluorophore is accompanied by changes in photophysical properties because the fluorophore moves to a free aqueous medium (Figure 10.39).
Dansyl (dimethylaminonaphthalene sulfonyl) fluorophore is a good candidate as an appended fluorophore because it is very sensitive to solvent polarity (see Chapter 7). Dansyl emits strong fluorescence in a hydrophobic environment, but weak

326 10 Fluorescent molecular sensors of ions and molecules
Fig. 10.39. Modified b-CDs with a single appended fluorophore (adapted from Ueno et al., 1997).
fluorescence in aqueous solutions. A guest-induced decrease in fluorescence intensity of compound b-CD4 with an appended dansyl moiety was indeed observed and the extent of this decrease parallels the a nity of the guest for the cavity. A remarkable result is the very large stability constant of the inclusion complex with ursodeoxycholic acid (> 106). Dansylglycine-, dansyl-L-leucine- and dansyl-L- leucine-modified b-CDs (b-CD5 and b-CD6) have also been prepared and show significantly di erent responses in the presence of the organic molecules mentioned above.
b-CDs bearing an appended p-dimethylaminobenzoyl (DMAB) moiety (called b- CD7) are of special interest. This fluorophore can form a twisted intramolecular charge transfer (TICT) in the excited state, like p-dimethylaminobenzonitrile (see Chapter 3, Section 3.4.4), and exhibits dual fluorescence: it can emit fluorescence from both the locally excited state and the TICT state, and the ratio of the intensities of the two bands depends on the microenvironment and in particular on solvent polarity. Circular dichroism experiments show that the DMAB moiety of b-CD7 is excluded from the cavity by forming an intermolecular inclusion complex with a guest molecule. Inclusion of the guest is accompanied by a decrease in the intensity of the TICT emission, changing the environment around the DMAB
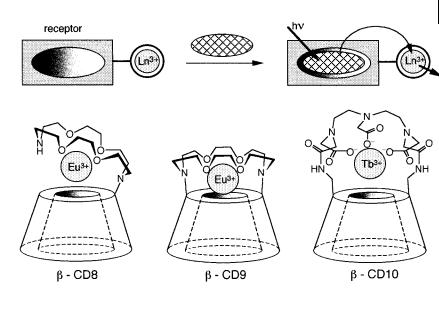
10.5 Fluorescent molecular sensors of neutral molecules and surfactants 327
Fig. 10.40. Modified b-CDs with an appended crown containing a lanthanide ion (adapted from Hartmann et al., 1997).
moiety from the hydrophobic interior to the polar water environment. It is interesting to note that the TICT emission depends on the size, shape and polarity of the guest molecules. Among the guests presented in Figure 10.38, the stability constants range from 2000 for cyclohexanol to 2:2 105 for 1-adamantanecarbox- ylic acid.
Compounds b-CD8, b-CD9 and b-CD10 (Figure 10.40) consisting of a b-CD with an appended azacrown able to complex a lanthanide ion have been designed for sensing small organic molecules that absorb in the UV and form inclusion complexes with b-CDs. The principle is that in the absence of sensitizers, lanthanide ions exhibit very weak luminescence because their molar absorption coe cients are very low (< 1 M 1 cm 1). But when an organic p-electron system is located at proximity, absorption of a UV photon by this species is followed by energy transfer to the lanthanide ion, which emits long-lived luminescence. Such a sensitized luminescence is well known in various lanthanide complexes. In the present case, the analyte bound to the receptor is excited by UV light and the excitation energy is transferred to the lanthanide ion, which acts as a signal transducer.
Addition of benzene to a solution of b-CD8 causes a two-fold enhancement of Eu3þ luminescence intensity. The stability constant of the complex between b-CD8 and benzene (@200) is comparable to that of the complex with the native b-CD. In contrast, benzoic and naphthoic acids show much stronger associations with b- CD8 than with native b-CD because association is assisted by the interaction between the carboxylic groups and the metal ion. Moreover, the enhancement factor of the luminescence is larger.
Surprisingly, no significant increase in luminescence intensity was observed
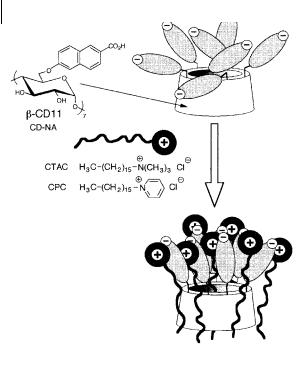
328 10 Fluorescent molecular sensors of ions and molecules
Fig. 10.41. Detection of cationic surfactants by a b-cyclodextrin with seven appended naphthoate fluorophores (Choppinet P. et al. (1996) J. Chem. Soc. Perkin Trans. 2, 249).
upon addition of benzene to b-CD9. This is because the association of benzene is defavored by the 3þ charge on the europium ion at the entrance of the cavity, which makes the interior of the latter less hydrophobic. This di culty is overcome by the three carboxylate groups of b-CD10 that neutralize the 3þ charge of the lanthanide ion. But Tb3þ must be used instead of Eu3þ in order to avoid low-lying carboxylate ligand-to-metal charge transfer excited states than can interfere with the energy transfer process when the metal ion is Eu3þ. Large enhancement factors have been observed, in particular with biphenyl and durene.
Modified cyclodextrins can also be used for the detection of cationic surfactants in the environment, which is of major interest; the high toxicity of these surfactants is linked to a slow biodegradation owing to their bactericidal nature. Very sensitive detection is possible by means of a b-cyclodextrin derivative CD-NA, bearing seven negatively charged naphthoate fluorophores (Figure 10.41). Interaction with a cationic surfactant leads to a drop in excimer emission. The ratio of the fluorescence intensities of the monomer and excimer bands is directly related to the concentration of the surfactant. In the case of electroactive surfactants such as cetylpyridinium chloride (CPC), the fluorescence quenching arising from photoinduced electron transfer can be additionally used for sensing. CD-NA permits detection of cetyltrimethylammonium chloride (CTAC) and cetylpyridinium chloride in an aqueous solution at concentrations as low as a few micromoles per liter and up to about 50 micromoles per liter. Interaction between CD-NA and cationic surfactants can be interpreted by a micellization process (Figure 10.41) induced by

10.5 Fluorescent molecular sensors of neutral molecules and surfactants 329
CD-NA rather than by the formation of 1:1 inclusion complexes. It should be noted that addition of the anionic surfactant sodium dodecylsulfate does not induce any photophysical e ect.
10.5.2
Boronic acid-based fluorescent sensors
Receptors containing diboronic acids can precisely recognize saccharide molecules. In fact, one boronic acid can reversibly form a boronate ester with two OH groups (one diol group), and one diboronic acid can immobilize two suitably positioned diol units to form a saccharide-containing macrocycle. Selectivity can be achieved by controlling the relative spatial position of the two boronic acids in relation to the cis-diol moieties on the saccharide. A given monosaccharide possesses at least two binding sites that di er from other monosaccharides.
Appropriate combinations of boronic acid and fluorophores lead to a remarkable class of fluorescent sensors of saccharides (Shinkai et al., 1997, 2000, 2001). The concept of PET (photoinduced electron transfer) sensors (see Section 10.2.2.5 and Figure 10.7) has been introduced successfully as follows: a boronic acid moiety is combined intramolecularly with an aminomethylfluorophore; consequently, PET from the amine to the fluorophore causes fluorescence quenching of the latter. In the presence of a bound saccharide, the interaction between boronic acid and amine is intensified, which inhibits the PET process (Figure 10.42). S-1 is an outstanding example of a selective sensor for glucose based on this concept (see Box 10.4).
S-2, in which the spacer between the two boronic acids is flexible, has the additional capability of forming excimers. The 1:1 binding of a saccharide leads to an increase in the monomer fluorescence intensity. This increase has two origins: the decrease in excimer formation, and the increase in fluorescence quantum yield resulting from suppression of the PET process. The 1:1 complex is formed at low saccharide concentrations, but increasing the concentration leads to the formation of the 1:2 complex, as revealed by the increase in the ratio of the intensities of the excimer band to the monomer band. The selectivity of S-2 was found to be similar to that of S-1.
Both steric and electronic factors are used for chiral recognition of saccharides by the R and S forms of S-3. A di erence in PET e ciency is created by the asymmetric immobilization of the amine groups relative to the binaphthyl moiety upon 1:1 complexation of saccharides by d- or l-isomers. For instance, d-fructose is recognized by the R form of S-2 with a large fluorescence enhancement.
10.5.3
Porphyrin-based fluorescent sensors
Porphyrins are very attractive as a ‘platform’ for the design of sensors for molecule recognition. The flat and relatively rigid structure of porphyrins o ers the possibility of constructing various types of recognition sites. Numerous functionalized
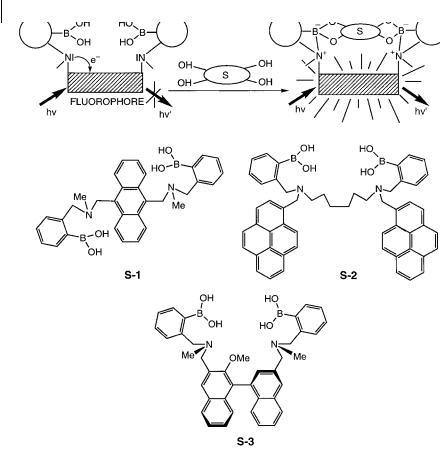
330 10 Fluorescent molecular sensors of ions and molecules
Fig. 10.42. Fluorescent sensors of saccharides based on boronic acids (adapted from James T. D. et al. (1996) Chem. Commun. 281).
porphyrins have been designed for the recognition of hydrophobic molecules, aminoacids, nucleobases, etc. Most of the investigations employ UV–vis spectrophotometry and/or NMR to demonstrate complex formation. This section will be limited to selected examples in which fluorescence is used for signaling the recognition event.
In line with the previous section, P-1 (Figure 10.43) associates a boronic acid moiety for recognition of diols (and in particular saccharides) and a tin-metallated porphyrin as a signaling moiety. This PET sensor changes its fluorescence intensity in an ‘on–o ’ manner instead of the usual ‘o –on’ manner. In the absence of analyte, the boronic acid moiety is positioned within a sterically crowded cleft of the porphyrin. The weak fluorescence emitted by P-1 can be explained by the residual boron–nitrogen interaction that prevents complete quenching by electron transfer from the intramolecular amino group to the porphyrin moiety. Upon binding of diols, the boronic moiety is pulled away from the cleft and the diol is suspended
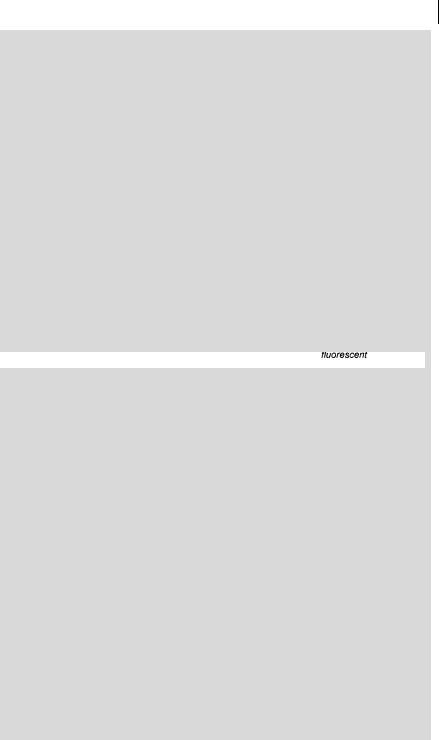
10.5 Fluorescent molecular sensors of neutral molecules and surfactants 331
Box 10.4 A fluorescent molecular sensor selective for glucose
Compound S-1, designed by Shinkai and coworkersa,b), can form di erent complexes with saccharides in methanol/waterc) bu er at pH 7.77, as shown in Figure B10.4.1: a non-cyclic 1:1 complex, a 1:1 cyclic complex and a 2:1 (saccharide:ligand) complex. The non-cyclic 1:1 complex could not be detected by fluorescence because both binding sites must be occupied in order to prevent fluorescence quenching by PET.
In the cyclic 1:1 complex, glucose is held close to the anthracene aromatic face, as represented in Scheme B10.4.1. In fact, the 1H-NMR spectrum exhibits a very large paramagnetic shift for the H3 proton ( 0.3 ppm), which thus points towards the p-electrons of the anthracene moietya,b).
Norrild and coworkersd) showed that this structure is only valid as an initial complex formed under completely non-aqueous conditions. In the presence of water, a rapid rearrangement from the a-d-glucopyranose form to the a-d- glucofuranose occurs. In the latter form, all five free hydroxy groups of glucose are covalently bound to the sensor molecule (Figure B.10.4.2).
It should be emphasized that binding of glucose by S-1 occurs at very low concentrations. Detection at physiological levels is possible, as demonstrated by
Fig. B10.4.1. Formation of non-cyclic and cyclic complexes of saccharides with the diboronic receptor S-1a,b).
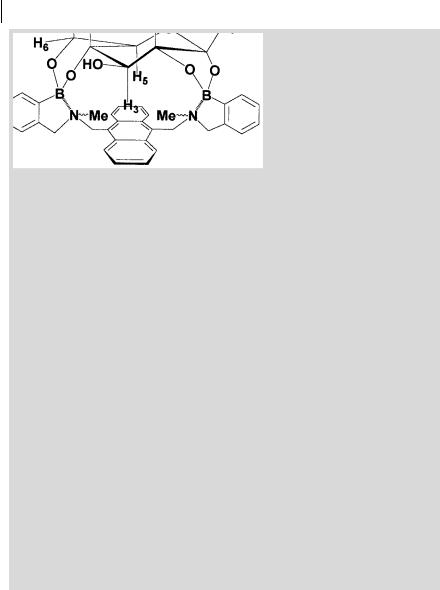
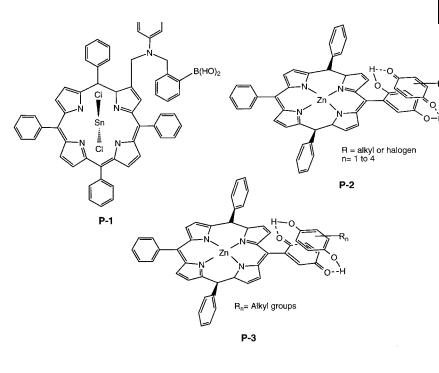
10.6 Towards fluorescence-based chemical sensing devices 333
Fig. 10.43. Porphyrin-based fluorescent sensors of neutral molecules (P-1: Kijima H. et al. (1999) Chem. Commun. 2011. P-2: D’Souza F. (1996) J. Am. Chem. Soc. 118, 923. P-3: D’Souza F. (1997) Chem. Commun. 533).
P-3, consisting of a free-base porphyrin with an appended quinone, is weakly fluorescent due to PET. Complexation with substituted hydroquinones induces fluorescence enhancement because of the unfavorable conditions for PET from the porphyrin to the quinone–hydroquinone entity.
10.6
Towards fluorescence-based chemical sensing devices
From a general point of view, a chemical sensor is a device capable of continuously monitoring the concentration of an analyte. The two main classes are electrochemical sensors and optical chemical sensors. The latter are based on the measurement of changes in an optical quantity: refractive index, light scattering, reflectance, absorbance, fluorescence, chemiluminescence, etc. For remote sensing, an optical fiber is used, and the optical sensor is then called an optode2) because of
2) This term comes from the Greek |
combination of the words ‘optical’ and |
(!pt!v ¼ visible, !d!v ¼ way) and is preferred |
‘electrode’. |
to the term optrode that results from the |
|
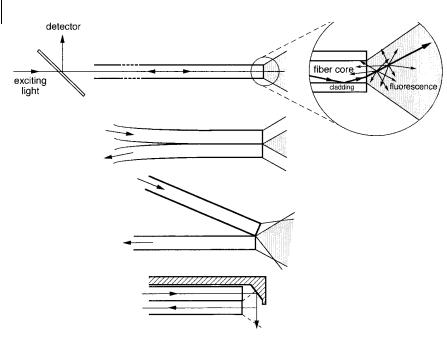
334 10 Fluorescent molecular sensors of ions and molecules
Fig. 10.44. Examples of optical configurations for passive optical sensors.
the analogy with the use of electrodes. However, the operating principles of optodes and electrodes are quite di erent.
Electrochemical sensors have several disadvantages with respect to optical sensors: (i) they are based on electrodes and require a reference electrode; (ii) the liquid–liquid junction is easily perturbed by external factors; (iii) they are sensitive to electrical interferences; (iv) miniaturization is not easy and their cost is relatively high. However, optical sensors also have some disadvantages: (i) ambient light can interfere; (ii) the range over which the concentration of an analyte can be accurately measured is often limited; (iii) they have generally limited long-term stability.
Among optical sensors, those based on fluorescence are of major interest because of their ability to use spectral and temporal information: multi-wavelength measurements allow simultaneous detection of two or more analytes, and discrimination between analytes is possible by time-resolved measurements. Multiplex capabilities represent the main advantage of such sensors compared to electrochemical devices.
Very di erent techniques are used in the design of fluorescence-based optical sensors. Passive and active modes of operation should be distinguished.
. In the passive mode, the optical device measures the variation in fluorescence characteristics (intensity, lifetime, polarization) of an intrinsically fluorescent analyte. The optical device can have di erent optical configurations involving in most cases an optical fiber (passive optode) (Figure 10.44).