
Molecular and Cellular Signaling - Martin Beckerman
.pdf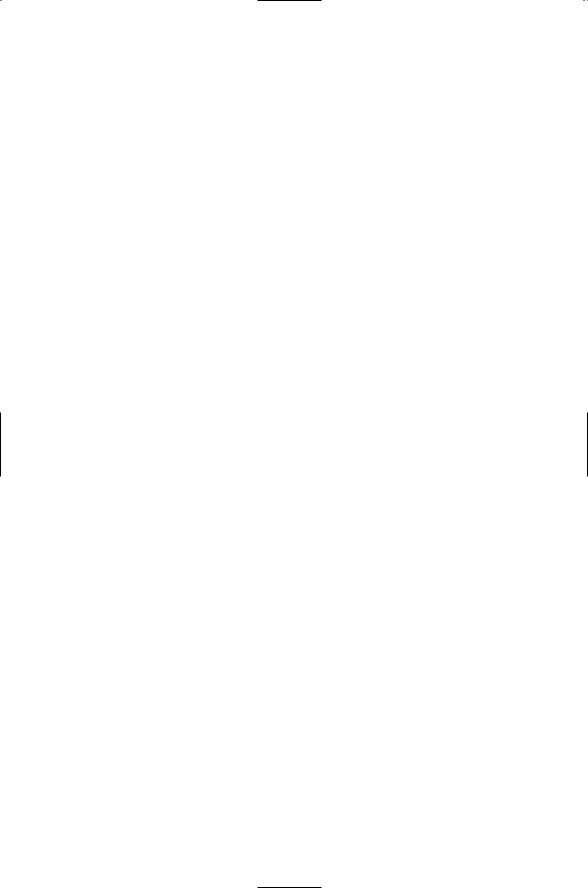
266 11. Signaling in the Endocrine System
gamma phosphate group to the GDP molecule. The result is an increase in the rate of hydrolysis from one GTP molecule every 35 minutes to 102 to 103 molecules per minute.
11.15Role of Rho, Rac, and Cdc42, and Their Isoforms
Rho, Rac, and Cdc42, and their isoforms, coordinate the reorganization of the actin cytoskeleton in response to extracellular signals. Members of the Rho family of GTPases—Rho, Rac, and Cdc42—regulate cell polarity, cell morphogenesis and shape, and cell motility. Each of these GTPases operates in a different pathway. In fibroblasts, Rho promotes the formation stress fiber bundles, Rac proteins regulate actin polymerization resulting in the formation of lamellipodia, and Cdc42 proteins control the formation of filopodia. All three regulate the formation of focal adhesion complexes. These observations are not limited to fibroblasts but rather are believed to take place in all eukaryotic cells. In addition to their role in remodeling the actin cytoskeleton, Rho family members regulate gene transcription. Signaling through receptor tyrosine kinases, G protein-coupled receptors and cytokine receptors activate Rho GTPases. In many instances integrins and Rho family members work together to regulate the actin cytoskeleton.
An examination of the steps leading to bud formation in yeasts provides some insight into how cell polarity develops and is regulated by the Rho family GTPases.The Cdc42 GTPase, its GEF called Cdc24, its GAP, of which there are several, and a scaffold protein named Bem1 contribute to the start of budding.As is the case for all GTPases, Cdc42 goes through a cycle of GDP release catalyzed by its GEF immediately followed by GTP binding. This state is followed some time later by hydrolysis catalyzed by its GAP in which
GTP is cleaved leaving GDP bound to the CDc42 protein. Unlike Ras, the
Cdc42 protein does not act on multiple substrate proteins in its GTP-bound state. Rather it goes through repeated cycles of GDP-GTP-GDP binding and hydrolysis to regulate the polymerization of actin fibers. The difference between the Ras switchlike behavior and Cdc42 assembly controller-like behavior is depicted in Figure 11.8. In the Ras mode of cycling, the GTPase is on and can influence many substrate proteins before being switched off by hydrolysis. In the Cdc42 mode of operation (Figure 11.8b), hydrolysis is required. Substrate interactions are again initiated by GTP binding but the action is not completed until the hydrolysis part of the cycle is executed. The EF-Tu GTPase uses this method of repeatedly cycling on and off during the translation elongation process. This second mode allows for some checking and quality control over the process; the assembly step can be aborted prior to hydrolysis if errors are detected.
Cell polarization (and bud formation) is typically driven by environmental cues such as gradients of chemoattractants or by signals from neighboring cells. These cues tell the cell which way is “up” and which way is
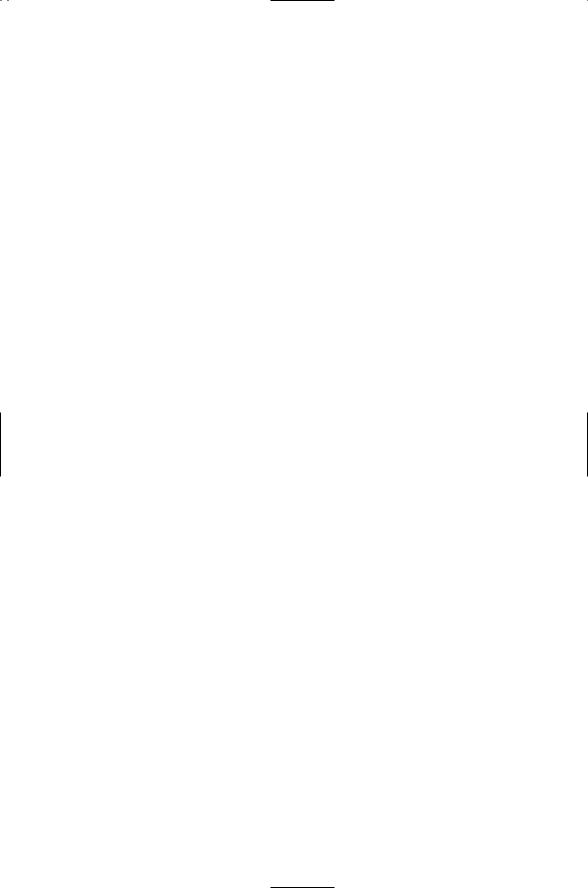
11.16 Ran Family Coordinates Traffic In and Out of the Nucleus |
267 |
“down.” In bud formation, the adapter protein Bem1 is recruited to the site of bud growth where it interacts with Ccd42 and its Cdc24 GEF. The GEF is recruited and stabilized at the plasma membrane by the Gbg subunit activated by GPCR signaling. Both Cdc42 and Cdc24 bind to the scaffold and a positive feedback loop is set up in which additional Bem1 proteins are recruited. A crucial element in the choice of bud site selection is the presence of position landmarks in the cell that identify the location of the poles. If these landmarks are absent the yeast cell is still able to form buds by a process in which a bud site is randomly selected.
11.16Ran Family Coordinates Traffic In and Out of the Nucleus
While small molecules can rapidly and freely diffuse in and out of the nucleus, macromolecules of size 40 to 50 kDa or greater cannot. Instead they are transported through selective and facilitated diffusion through nuclear pore complexes. Nuclear pore complexes (NPCs) are large units built up from more than 100 different proteins. The NPCs function as selective gates. Only the larger macromolecules containing the correct targeting or localization sequence are allowed through. Nuclear pore complexes have no motors to actively transport cargo. Instead, import and export occur by means of facilitated diffusion. This process does not consume energy and is nondirectional. Proteins, mRNAs, tRNA, ribosomal subunts, and other macromolecules are transported bound to transport molecules called importins and exportins that recognize the nuclear localization signals (NLSs) and nuclear export signals (NESs). These transport receptors together with adapter molecules, Ran GTPases, Ran GEFs, and Ran GAPs facilitate the diffusion of the macromolecules in and out of the nucleus.
Transport receptors belonging to the importin b family shuttle cargo in and out of the nucleus through the NPC. These receptors are encountered in two forms: as importins that shuttle cargo into the nucleus and as exportins that chaperone cargo the other way. The loading and release of cargo by the importin b receptors is regulated by the Ran GTPases. Ran, a 25-kDa protein, is an abundant gene product found in all eukaryotic cells. The key element in their ability to regulate cargo movement is the formation of a Ran concentration gradient across the nuclear envelope. This gradient in GTP-bound Ran is established and maintained by the Ran GEFs and Ran GAPs. The Ran GAPs are restricted to the cytoplasm and cannot enter the nucleus, while the Ran GEFs are localized exclusively in the nucleus. As a result the concentration of GTP-bound Ran is low in the cytoplasm and high in the nucleus.
The combined actions of importin b receptors and Ran GTPases are illustrated in Figure 11.9. As is the case for all GTPases its main actions occur during the GTP-bound part of the cycle, when it shuttles exportin + cargo and unbound importin molecules through the NPC from the nucleus to the
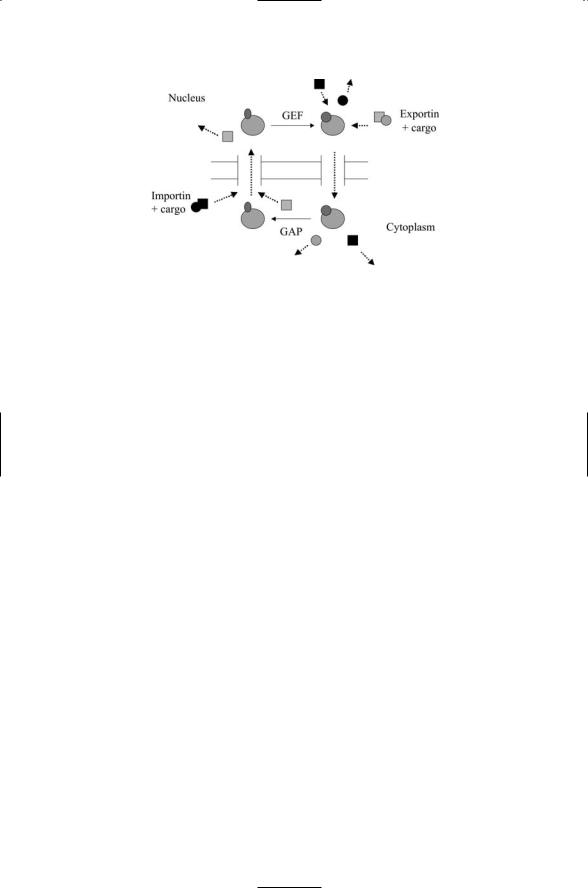
268 11. Signaling in the Endocrine System
FIGURE 11.9. Ran GTPase-mediated import and export through nuclear pore complexes (NPCs): Importin (dark square) shuttles its cargo (dark circles) through the NPC into the nucleus. In the nucleus, GTP-bound Ran GTPase triggers the release of the cargo from the importin and shuttles the importin back out to the cytoplasm. The GTP-bound Ran GTPase shuttles exportin (light squares) plus its cargo (light circles) through the NPC from the nucleus to the cytoplasm where other proteins (not shown) help dissociate the complex. The unbound exportin then diffuses back to the nucleus.
cytoplasm. It differs from the Ras switching and Cdc42 cytoskeleton assembly in so far as its GEF and GAP actions take place in different compartments. Hydrolysis is not required for movement through the pore and release of the cargo, but this aspect changes when Ran mediates assembly of the nuclear envelope. In those assembly operations hydrolysis is necessary.
11.17Rab and ARF Families Mediate the Transport of Cargo
Recall that in eukaryotes secreted soluble molecules and plasma membrane lipids and proteins are transported from compartment to compartment by transport vesicles. Newly synthesized proteins and lipids move from the ER to the Golgi apparatus and from there to the plasma membrane. The vesicles that transport these molecules are produced by budding from the membrane of the donor compartment, and upon arrival to the acceptor compartment fuse with the membrane of the acceptor compartment thereby transferring the cargo. The process of delivering cargo from the internal compartments to the plasma membrane is known as exocytosis. Cargo moves in two directions, outward and inward. Many membrane components are rapidly turned over. Vesicles transport macromolecules from
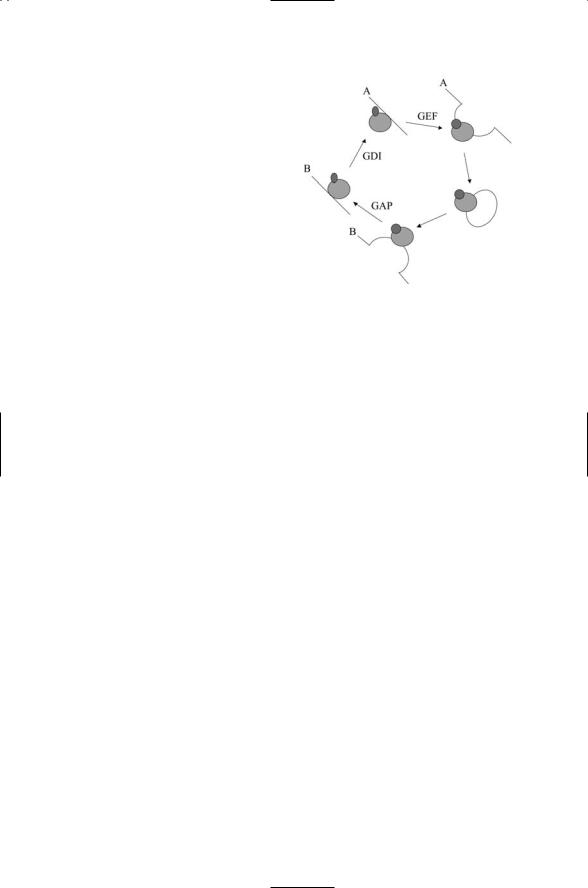
11.17 Rab and ARF Families Mediate the Transport of Cargo |
269 |
FIGURE 11.10. Rab GTPase cycle: The Rab GTPase cycle synchronizes vesicle transport and fusion between the membranes labeled A and B. In the cycle, a GDP-bound Ran protein is shuttled by its GDI to membrane A where GDP is released and GTP is bound in its place. While in its active form, a cargo vesicle pinches off from the membrane and is conveyed to membrane B where it docks and then fuses. Hydrolysis occurs and, assisted by the GDI, the GDPbound Ran protein is released from the membrane and returned to the cytosol.
the plasma membrane either to the lysosomes, where they are degraded, or to endosomes, where they are recycled back to the plasma membrane. The process of transporting plasma membrane molecules to lysosomes for degradation is known as endocytosis, and the outward and inward movement of plasma membrane molecules is said to take place in the secretory and endocytic pathways.
The Rab family with more than 30 known members is the largest group of Ras superfamily GTPases. These proteins coordinate the docking and fusion of cargo vesicles operating in the secretory and endocytic pathways. Reciprocal pairs of proteins called v-SNAREs and t-SNAREs mediate the fusion of cargo vesicles. The binding of the SNAREs is preceded by tethering/protection protein-binding steps that ensure that only the appropriate fusion events takes place. The Rab proteins are localized to the cytoplasmic face of organelles and vesicles and participate in the preliminary binding operations.
The Rab protein cycle of GDP and GTP binding and release is synchronized with the movement and fusion of cargo vesicles. The combined set of steps is illustrated in Figure 11.10. In this figure the GEFs and GAPs are joined by a third set of accessory regulators—GDP dissociation inhibitors, or GDIs. These molecules bind and maintain pools of inactive Rab proteins in the cytosol and serve as recycling chaperones. They first help to release the GDPases from the membranes and then shuttle them back to their site of origin in the cytosol in preparation for their next cycle of use.
ADP-robosylation factors (Arfs) make up the fifth family of Ras
GTPases. These 20-kDa proteins help regulate the formation of cargo vesicles through budding from donor membranes. In order for budding to take place, coat proteins must be assembled over the membrane surface. These binding agents, coat protein I (COPI), coat protein II (COPII), and clathrin, mediate the mechanical forces that pull a membrane into a bud, and they
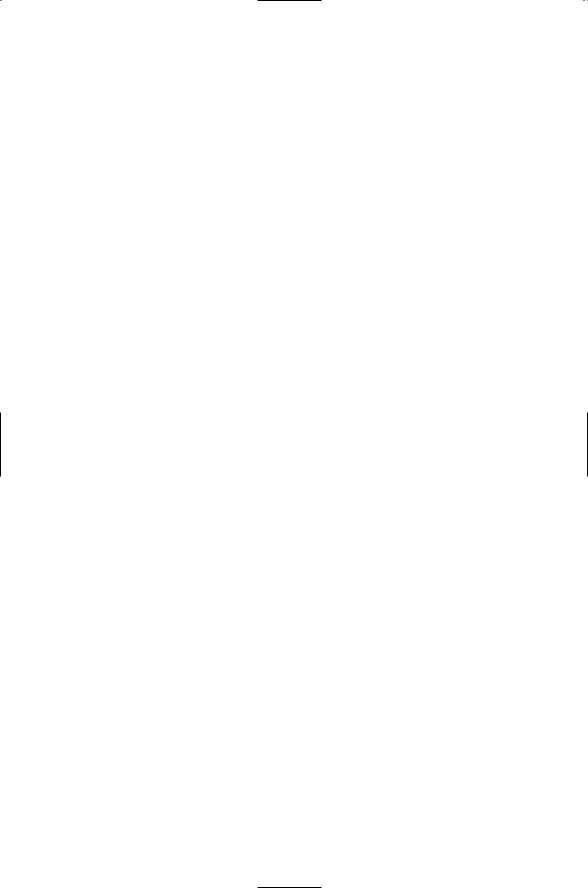
270 11. Signaling in the Endocrine System
help capture membrane receptors and cargo. The Arf proteins recruit the coat proteins to the donor membrane.
References and Further Reading
Angiogenesis
Ferrara N, Gerber HP, and LeCouter J [2001]. The biology of VEGF and its receptors. Nature Med., 9: 669–676.
Gale NW, and Yancopoulos GD [1999]. Growth factors acting via endothelial cellspecific receptor tyrosine kinases: VEGFs, angiopoietins and ephrins in vascular development. Genes Dev., 13: 1055–1066.
Jones N, et al. [2003]. Tie receptors: New modulators of angiogenic and lymphangiogenic responses. Nature Rev. Mol. Cell Biol. 2: 257–267.
Neufeld G, et al. [1999]. Vascular endothelial growth factor (VEGF) and its receptors. FASEB J., 13: 9–22.
Yancopoulos GD, et al. [2000]. Vascular-specific growth factors and blood vessel formation. Nature, 407: 242–248.
Neurotrophins
Chao MV [2003]. Neurotrophins and their receptors: A convergence point for many signaling pathways. Nature Rev. Neurosci., 4: 299–309.
Lee R, et al. [2001]. Regulation of cell survival by secreted proneurotrophins. Science, 294: 1945–1948.
Zheng YZ, et al. [2000]. Cell surface Trk receptors mediate NFG-induced survival while internalized receptors regulate NFG-induced differentiation. J. Neurosci., 20: 5671–5678.
Ligand-Induced, Receptor-Mediated Dimerization
Schlessinger J [2002]. Ligand-induced, receptor-mediated dimerization and activation of EGF receptor. Cell, 110: 669–672.
Phosphoprotein Recognition
Berg D, Holzmann C, and Reiss O [2003]. 14-3-3 proteins in the nervous system.
Nature Rev. Neurosci., 4: 752–762.
Durocher D, and Jackson SP [2002]. The FHA domain. FEBS Lett., 513: 58–66. Forman-Kay JD, and Pawson T [1999]. Diversity in protein recognition by PTB
domains. Curr. Opin. Struct. Biol., 9: 690–695.
Pawson T, Gish GD, and Nash P [2001]. SH2 domain, interaction modules and cellular wiring. Trends Cell Biol., 11: 504–511.
Tzivion G, and Avruch J [2002]. 14-3-3 proteins: Active cofactors in cellular regulation by serine/threonine phosphorylation. J. Biol. Chem., 277: 3061–3064.
Yaffe MB [2002]. Phosphotyrosine-binding domains in signal transduction. Nature Rev. Mol. Cell Biol., 3: 177–186.
Recognition of Proline-Rich Motifs
Ball LJ, et al. [2002]. EVH1 domains: Structure, function and interactions. FEBS Lett., 513: 45–52.
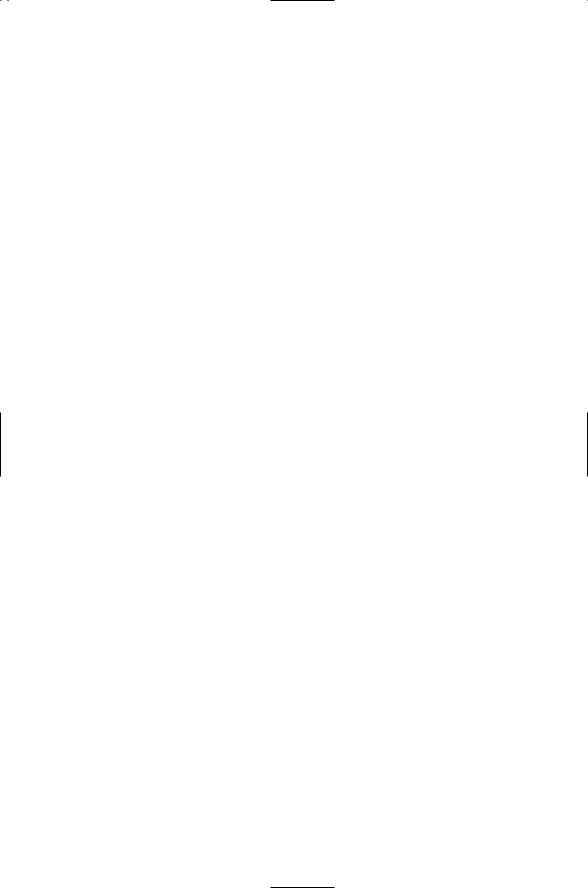
References and Further Reading |
271 |
Freund C, et al. [1999]. The GYF domain is a novel structural fold that is involved in lymphoid signaling through praline-rich sequences. Nature Struct. Biol., 6: 656–660.
Kay BK, Williamson MP, and Sudol M [2000]. The importance of being proline: The interaction of proline-rich motifs in signaling proteins with their cognate domains. FASEB J., 14: 231–241.
Macias MJ, Wiesner S, and Sudol M [2002]. WW and SH3 domains, two different scaffolds to recognize proline-rich ligands. FEBS Lett., 513: 30–37.
Mayer BJ [2001]. SH3 domains: Complexity in moderation. J. Cell Sci., 114: 1253–1263.
Zarrinpar A, and Lim WA [2000]. Converging on proline: The mechanism of WW domain peptide recognition. Nature Struct. Biol., 7: 611–613.
Protein–Protein Interaction Domains
Aravind L, Dixit MV, and Koonin EV [1999]. The domains of death: evolution of the apoptosis machinery. Trends Biochem. Sci., 24: 47–53.
Harris BZ, and Lim WA [2001]. Mechanism and role of PDZ domains in signaling complex assembly. J. Cell Sci., 114: 3219–3231.
Hepler JR [1999]. Emerging roles for RGS proteins in cell signaling. Trends Pharmacol. Sci., 20: 376–382.
Hofmann K [1999]. The modular nature of apoptotic signaling proteins. Cell. Mol. Life Sci., 55: 1113–1128.
Hung AY, and Sheng M [2002]. PDZ domains: Structural modules for protein complex assembly. J. Biol. Chem., 277: 5699–5702.
Schultz J, et al. [1997]. SAM as a protein interaction domain involved in developmental regulation. Protein Sci., 6: 249–253.
Zhong H, and Neubig RR [2001]. Regulator of G protein signaling proteins: Novel multifunctional drug targets. J. Pharmacol. Exp. Ther., 297: 837–845.
Src Nonreceptor Tyrosine Kinase
Brown MT, and Cooper JA [1996]. Regulation, substrates and function of Src.
Biochim. Biophys. Acta, 1287: 121–149.
Frame MC, et al. [2002]. v-Src’s hold over actin and cell adhesions. Nature Rev. Mol. Cell Biol., 3: 233–245.
Focal Adhesions
Ilic D, Damsky CH, and Yamamoto T [1997]. Focal adhesion kinase: At the crossroads of signal transduction. J. Cell Sci., 110: 401–407.
Schlaepfer DD, Hauck CR, and Sieg DJ [1999]. Signaling through focal adhesion kinase. Prog. Biophys. Mol. Biol., 71: 435–478.
Turner CE [2000]. Paxillin and focal adhesion signaling. Nature Cell Biol., 2: E231–E236.
Renshaw MW, Ren XD, and Schwartz MA [1997]. Growth factor activation of MAP kinase requires cell adhesion. EMBO J., 16: 5592–5599.
Ras Family of GTPases
Campbell SL, et al. [1998]. Increasing complexity of Ras signaling. Oncogene, 17: 1395–1413.
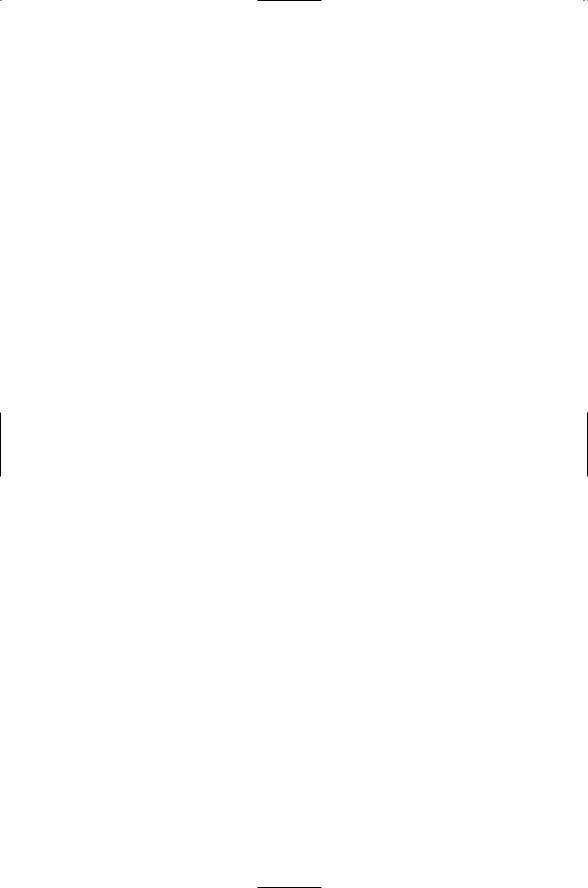
272 11. Signaling in the Endocrine System
Hancock JF [2003]. Ras proteins: Different signals from different locations. Nature Rev. Mol. Cell Biol., 4: 373–384.
Kolch W [2000]. Meaningful relationships: The regulation of the Ras/Raf/MEK/ ERK pathway by protein interactions. Biochem. J., 351: 289–305.
Rho Family of GTPases
Gladfelter AS, et al. [2002]. Septin ring assembly involves cycles of GTP loading and hydrolysis by Cdc42p. J. Cell Biol., 156: 315–326.
Hall A [1998]. Rho GTPases and the actin cytoskeleton. Science, 279: 509–514. Irazoqui JE, Gladfelter AS, and Lew DJ [2003]. Scaffold mediated symmetry break-
ing by Cdc42p. Nature Cell Biol., 5: 1062–1070.
Ren XD, Kiosses WB, and Schwartz MA [1999]. Regulation of the small GTPbinding protein Rho by cell adhesion and the cytoskeleton. EMBO J., 18: 578–585.
Schwartz MA, and Shattil SJ [2000]. Signaling networks linking integrins and Rho family GTPases. Trends Biochem. Sci., 25: 388–391.
Ran Family of GTPases
Görlich D, Seawald MJ, and Ribbeck K [2003]. Characterization of Ran-driven cargo transport and the RanGTPase systems by kinetic measurements and computer simulation. EMBO J., 22: 1088–1100.
Macara IG [2001]. Transport in and out of the nucleus. Microbiol. Mol. Biol. Rev., 65: 570–594.
Melchior F, and Gerace L [1998]. Two-way trafficking with Ran. Trends Cell Biol., 8: 175–179.
Weis K [1998]. Importins and exportins: How to get in and out of the nucleus. Trends Biochem. Sci., 23: 185–189.
Weis K [2003]. Regulating access to the genome: Nucleocytoplasmic transport throughout the cell cycle. Cell, 112: 441–451.
Rab and Arf Families of GTPases
Moss J, and Vaughn M [1995]. Structure and function of Arf proteins: Activators of cholera toxin and critical components of intracellular vesicle transport. J. Biol. Chem., 270: 12327–12330.
Zerial M, and McBride H [2001]. Rab proteins as membrane organizers. Nature Rev. Mol. Cell Biol., 2: 107–117.
Recycling, and Signaling in the Endocytic Pathway
González-Gaitán M [2003]. Signal dispersal and transduction through the endocytic pathway. Nature Rev. Mol. Cell Biol., 4: 213–224.
Sorkin A, and von Zastrow M [2002]. Signal transduction and endocytosis: Close encounters of many kinds. Nature Rev. Mol. Cell Biol., 3: 600–614.
Problems
11.1(a) Binding interactions. One of the standard questions addressed in investigations of signal transduction asks what interactions are possible in a particular molecular setting. A number of proteins
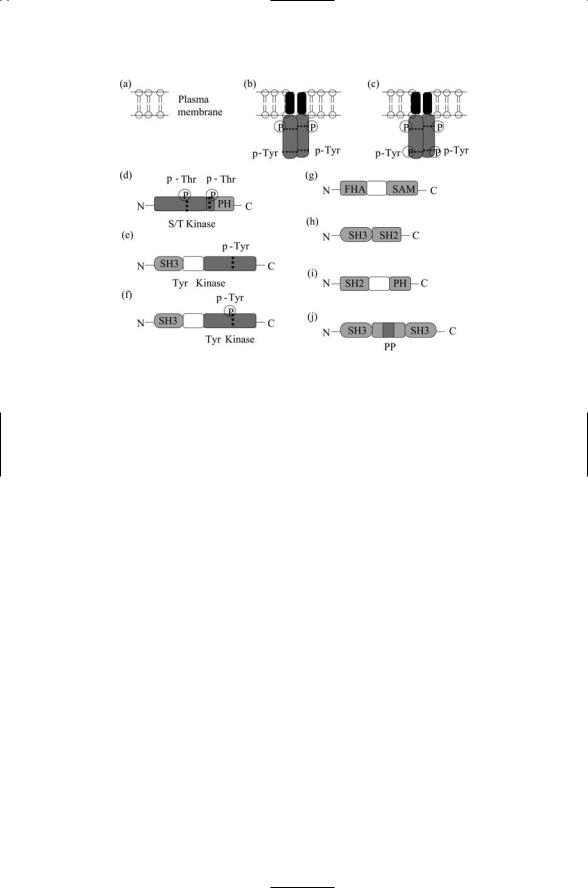
Problems 273
FIGURE FOR PROBLEM 11.1. Phosphorylated threonine and tyrosine residues are identified by a “P” enclosed in a circle. The symbol “PP” refers to proline-rich regions. Threonine and tyrosine phosphorylation sites with the correct flanking sequences for recognition by their kinases are indicated by the symbols p-Thr and p-Tyr. Note that phosphorylation at the sites located in the center of the catalytic domains of the NRTKs is required for activation.
and membranes are shown in the accompanying figure. Identify the possible interactions in a mixture containing multiple copies of each element (a) through (j).
(b)Build your own. Starting at the plasma membrane construct one or more signaling pathways using the elements (a) through (j).
11.2Nerve cells are highly polarized structures, and their terminals are located far from the cell body where the nucleus is located. Signals sent from axon terminals to the cell body must travel distances of up to a meter. These signals are referred to as retrograde signals since they move in a direction opposite to action potentials, which travel down the axon to the nerve terminal. How might a neuron generate a rapid (i.e., faster than one conveyed by passive diffusion) retrograde signal, observing that receptor tyrosone kinases such as the Trks (and also G protein-coupled receptors) are internalized following ligand binding?
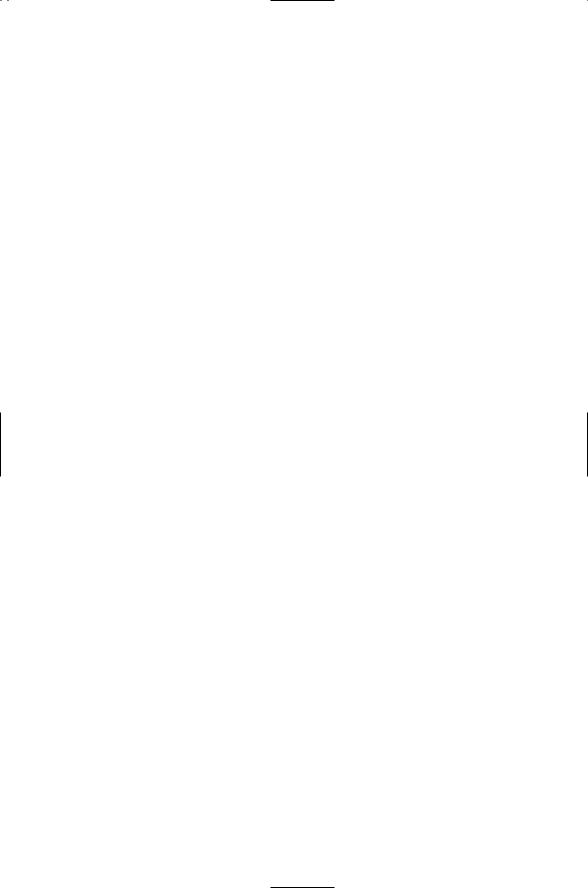
12
Signaling in the Endocrine and Nervous Systems Through GPCRs
The grouping formed by the G protein-coupled receptors (GPCRs) is the largest of its kind in the body. Recent estimates of the number of GPCRs in humans fall in the range of 800 or so. This number is divided roughly in half between receptors that bind sensory signals originating from outside the body (exogenous ligands) and signals produced internally by other cells (endogenous ligands). GPCRs transduce a remarkably diverse spectrum of messages into the cell. Among the signals transduced are light (phototransduction), extracellular calcium ions, tastants (gustatory), odorants (smell), pheromones (mating signals), warnings (pain), immunological (chemokines), endocrine (hormones), and neural (neuromodulators and neurotransmitters).
Members of the GPCR superfamily are sometimes referred to as the 7TM receptors because their chains pass back and forth through the plasma membrane seven times. The GPCRs transmit messages into the cell by activating heterotrimeric G proteins and by sending signals through growth pathways. In the absence of GPCR ligand binding, heterotrimeric G proteins remain in close association with the GPCRs. Ligand binding leads to G protein dissociation and signaling through second messengers that target protein kinases/phosphatases and ion channels. In the first part of the chapter, the general characteristics of GPCR signaling will be examined, and second messengers initially discussed in Chapter 8 will be looked at in more detail.
The GPCRs are the targets of many therapeutic drugs due to the receptors’ prominent involvement in the endocrine and nervous systems. It is estimated that some 40 to 60% of all therapeutic drugs target GPCRs. Some drugs act as agonists and others as antagonists. An agonist induces the same response in the receptor as that triggered by the natural ligand. An antagonist binds to the receptor but the receptor does not transmit a signal in response to the binding event. By binding the receptor the antagonist blocks access of the natural ligand to the receptor and thus prevents transmission of a signal. Drugs that bind in an antagonistic fashion are known as blockers. Prominent examples are beta blockers, which antagonize the beta-
275
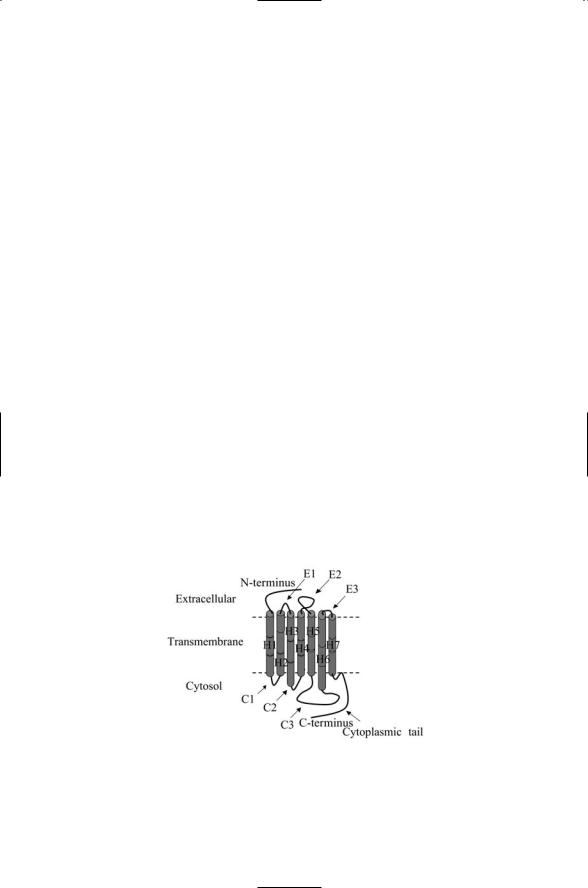
276 12. Signaling in the Endocrine and Nervous Systems Through GPCRs
adrenergic receptor, and antihistamines, which inhibit the histamine H1 receptor. The second part of the chapter will provide an overview of signaling using hormones, neurotransmitters, and neuromodulators (endogenous ligands) and how light and other exogenous ligands are transduced into cellular responses.
12.1 GPCRs Classification Criteria
G protein-coupled receptors share little sequence homology with one another yet all are organized in a remarkably similar fashion. All are single chain polypeptides with a topology as depicted in Figure 12.1. The transmembrane segments are composed of hydrophobic amino acid residues arranged into an alpha helix. The helices are connected to one another by three extracellular loops E1–E3 and three cytoplasmic loops C1–C3. Each GPCR has an extracellular N-terminal region and a cytoplasmic C- terminal region. The extracellular and cytoplasmic regions can be quite large. The extracellular loop E2 and the N-terminus segment are especially well situated for ligand binding. These structures vary in size from one class of GPCR to another. The intracellular loops plus the cytoplasmic ends of the transmembrane helices participate in protein binding and activation. The cytoplasmic tail and third intracellular loop (C3), in particular, provide multiple sites for protein docking and interactions.
GPCRs can be grouped into families according to the most prominent characteristics of the extracellular and intracellular domains, loop properties, and the presence of structurally important disulfide bridges. GPCRs possess a number of highly conserved sequences that influence the structure and binding properties of the GPCRs. These, too, enter into the classification process. There are three main families of GPCRs and a growing
FIGURE 12.1. Stereotypic representation of a rhodopsin family (Class A) G proteincoupled receptor: Shown are the seven membrane-spanning alpha helices H1–H7, three extracellular loops E1–E3, and three cytoplasmic loops C1–C3.