
Molecular and Cellular Signaling - Martin Beckerman
.pdf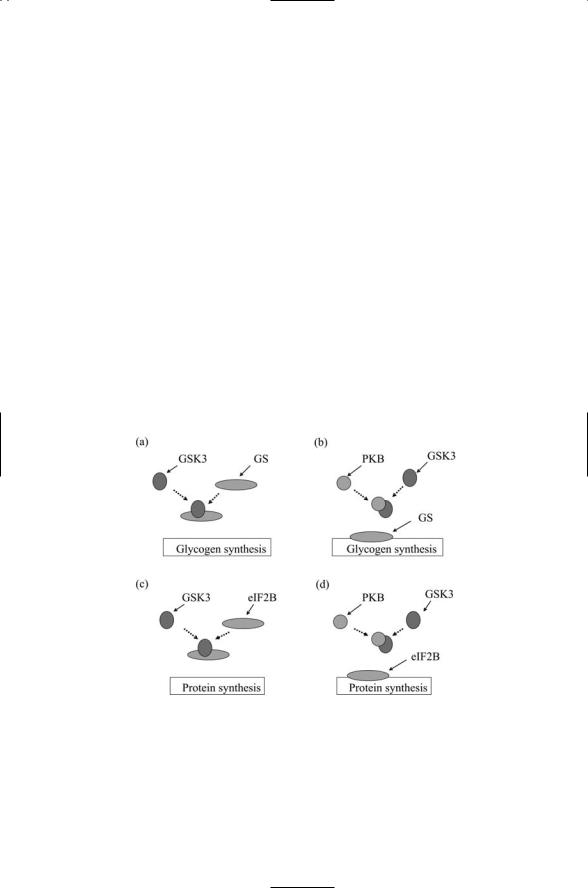
8.12 Lipids and Upstream Kinases Activate PKB |
175 |
PH domain. In response the kinase migrates to the plasma membrane where it is phosphorylated by 3-phosphoinositide-dependent kinase-1 (PDK-1) and then again at a second location either by another kinase or by itself. Once it is recruited to the plasma membrane and phosphorylated, the PKB enzyme is fully activated. As discussed earlier PIP3-mediated signaling is terminated by a protein phosphatase PTEN that converts PIP3 to PIP2.
Protein kinase B is centrally involved in insulin signaling. One of its immediate downstream targets is glycogen synthase kinase 3 (GSK3). In the absence of insulin signaling, GSK3 is active, and when it phosphorylates glycogen synthase it inhibits its enzymatic stimulation of glycogen synthesis. Signaling is fairly rapid. Within a few minutes of insulin binding, PKB is activated and phosphorylates GSK3, thereby inactivating it so that it cannot phosphorylate glycogen synthase. The latter becomes dephosphorylated and consequently is better able to stimulate glycogen production. Another effect of insulin binding leading to GSK3 inactivation is that of an increase in protein translation. This, too, is slowed down by GSK3, but insulin signaling frees the protein translation initiation regulator eIF2B from its inhibition by GSK3 (Figure 8.11).
FIGURE 8.11. Insulin signaling through protein kinase B: (a) In the absence of PKB activity GSK3 inhibits the stimulation of glycogen synthesis by glycogen synthase (GS). (b) When PKB is activated it binds to and prevents GSK3 from inhibiting GS.
(c) In the absence of PKB activity GSK3 inhibits the initiation of protein synthesis by eIF2B. (d) When PKB is activated it binds to and prevents GSK3 from inhibiting eIF2B.
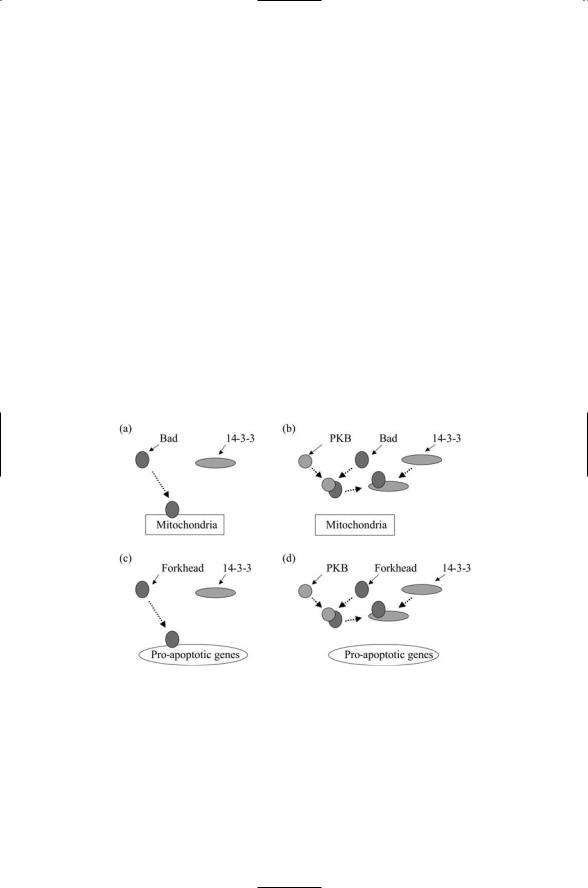
176 8. Organization of Signal Complexes by Lipids, Calcium, & Cyclic AMP
8.13 PKB Supplies a Signal Necessary for Cell Survival
Protein kinase B is activated by a number of signals, not just insulin. It is activated through PI3K when growth factors bind to members of the receptor tyrosine family to which the insulin receptor belongs. The effect on the cell of protein kinase B signaling is to enhance cell survival. It mainly does so by inhibiting proteins that promote cell suicide, or apoptosis. Two examples of this kind of action are presented in Figure 8.12. The first example is inhibition of Bad signaling. Bad is a member of a group of apoptosis regulators known as the Bcl-2 family that will be explored in Chapter 15. Some Bcl-2 family members promote apoptosis while others inhibit it. These proteins are regulated by phosphorylation. The Bad protein, in particular, promotes apoptosis, but this activity is turned off by phosphorylation. When PKB is fully activated, it diffuses over to and phosphorylates Bad, thereby preventing its proapoptosis actions.
The second example presented in Figure 8.12 is inhibition of Forkheadmediated transcription. The Forkhead protein is a transcription factor. Protein kinase B catalyzes the phosphorylation of substrates at sites characterized by the consensus sequence RXRXXS/T. Among the PKB
FIGURE 8.12. Survival signaling through protein kinase B: (a) In the absence of PKB signaling Bad translocates from the cytoplasm to the mitochondria where it promotes apoptotic responses. (b) When PKB is activated it phosphorylates Bad, which then binds to a 14-3-3 protein and becomes immobilized in the cytoplasm. (c) In the absence of PKB-signaling, the Forkhead (FH) transcription factor translocates from the cytoplasm to the nucleus where it promotes the expression of proapoptotic genes. (d) When PKB is activated it phosphorylates FH, which then binds to a 14- 3-3 protein and becomes immobilized in the cytoplasm.
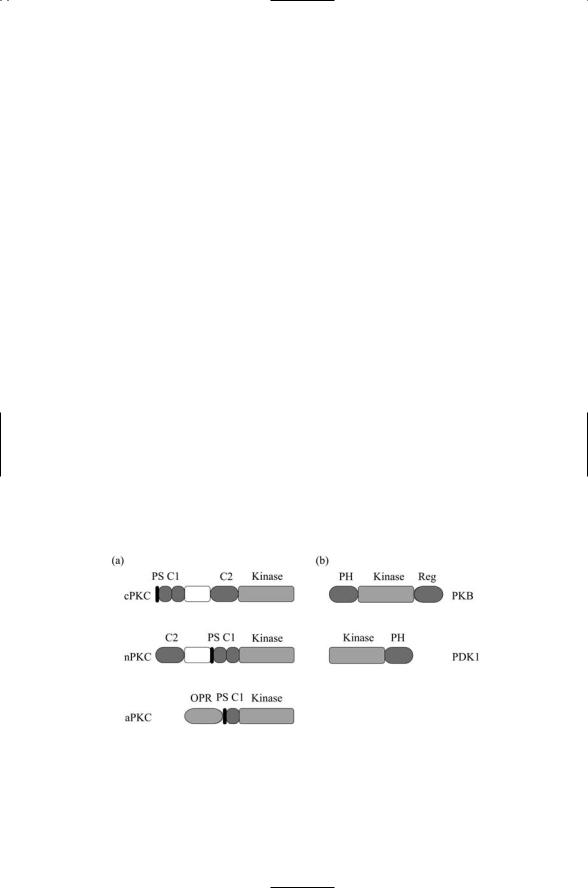
8.14 Phospholipids and Ca2+ Activate Protein Kinase C |
177 |
substrates possessing this consensus sequence are the transcription factors belonging to the Forkhead (FH) family. As was the case for Bad, phosphorylating these proteins inactivates them. In the absence of PKB signaling, the FH proteins translocate from the cytoplasm to the nucleus where they stimulate transcription of apoptosis-promoting genes. When protein kinase B is actived it can phosphorylate FH resulting in its binding to, and immobilization by, cytoplasmic 14-3-3 proteins. Binding to the 14-3-3 proteins prevents FH from carrying out its anticell-cycle progression actions, and thus again promotes cell survival. In both of these examples, the cellular response to lipid-mediated protein kinase B signaling is survival—the suppression of the cell suicide program, and continued progression through the cell cycle.
8.14 Phospholipids and Ca2+ Activate Protein Kinase C
There are several subfamilies of protein kinase C (PKC), each characterized by a slightly different domain structure. As depicted in Figure 8.13, the different isozymes of PKC belong to either the classical, novel, or atypical subfamilies. The pseudosubstrate (PS) is a portion of the chain that interacts with and blocks the activity of the catalytic domain, but activation relieves the block. There are three requirements for full activation of protein kinase C. The first is phosphorylation. Protein kinase C, like protein kinase B, is activated by phosphorylation. PKC is phosphorylated by upstream kinases such as PDK1. The second requirement is presence of subfamily-specific second messengers acting as coactivators. The various isozymes of protein kinase C are listed in Table 8.4 along with the associated coactivators.
FIGURE 8.13. Structure of protein kinase C and protein kinase B: (a) Protein kinase C families—Conventional (c), novel (n), and atypical (a) protein kinase C proteins.
(b) Protein kinase B and PDK1 proteins. Abreviations: pseudosubstrate (PS); Pleckstrin homology (PH); octicopeptide repeat (OPR).
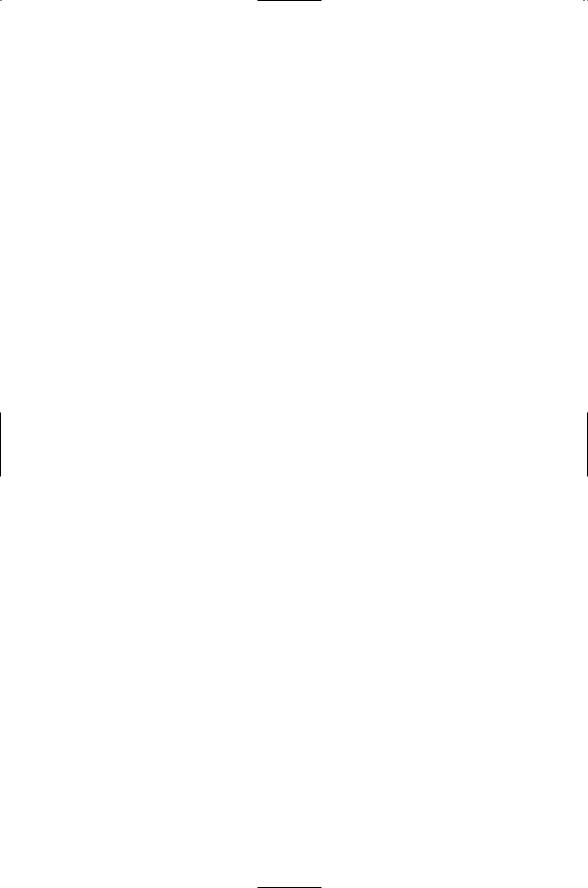
178 8. Organization of Signal Complexes by Lipids, Calcium, & Cyclic AMP
The best-characterized PKC proteins are the classical (conventional) isozymes. As indicated in Table 8.4, these enzymes are activated by calcium along with DAG. Their C1 domain binds DAG while their C2 domain binds Ca2+. Two other parts of the protein, C3 and C4, form the ATP and substrate-binding lobes of the catalytic core. The steps leading to second messenger signaling have been discussed. Binding of a ligand to a receptor activates PLC, which then splits PIP2 to create DAG and IP3. The latter stimulates the release of Ca2+ from intracellular stores and together the
Ca2+ and DAG activate protein kinase C. Binding of DAG to the C1 domain and Ca2+ to the C2 domain facilitates the recruitment of the protein to the plasma membrane, where it binds to the negatively charged phosphatidylserine molecules concentrated in the cytoplasmic leaflet. In this process the C1 domain is mostly responsible for the recognition of phosphatidylserine while the C2 domain confers a more general specificity for lipid membranes.
8.15Anchoring Proteins Help Localize PKA and PKC Near Substrates
Anchoring proteins were introduced in Chapter 6. These proteins do not function as enzymes but instead help localize the kinases near their substrates. They provide sites for the tethering of protein kinase A and protein kinase C, and protein phosphatases PP1 and PP2B, to the cytoplasmic face of the plasma membrane and similarly to organelle membranes. There are several prominent families of kinase-anchoring proteins, among which are the A-kinase anchoring proteins (AKAPs) and receptors for activated C kinase (RACKs).
The AKAPs localize protein kinase A close to its substrates. The AKAPs contain an amphipathic helix that binds to specific regulatory subunits of protein kinase A, and the AKAPs have a targeting sequence that directs the anchor protein to a specific subcellular location. Sites of AKAP attachment include cell membranes, cytoskeleton, nuclear matrix, and endoplasmic reticulum. The AKAPs serve as control points where the primary protein kinase-signaling elements, and their regulators and modulators, are brought together near or at their fixed infrastructure targets. The AKAPs serve as platforms for assembly and integration of several signaling proteins, thereby serving as points of control of their substrates.
The RACKs bind protein kinase C proteins once they have been partially activated by their upstream kinases and coactivators. Different isozymes of the PKCs localize to different subcellular locations. In response to production of cofactors such as Ca2+ and DAG, different PKC isozymes translocate from the plasma membrane to distinct subcellular locations, aided by the RACKs. Some PKCs are localized by their RACKs to the plasma membrane, where they phosphorylate L-type calcium channels,
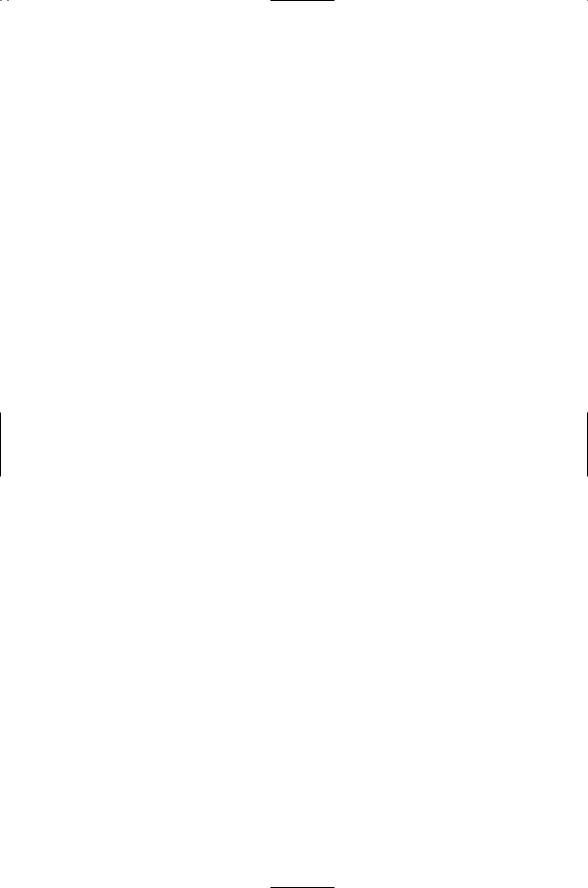
8.16 PKC Regulates Response of Cardiac Cells to Oxygen Deprivation |
179 |
ligand-gated ion channels (discussed in Chapter 18), and other membranebound signaling proteins. Other PKCs are localized to the nucleus or to the mitochondria or to other cellular compartments where they phosphorylate their substrates.
8.16PKC Regulates Response of Cardiac Cells to Oxygen Deprivation
Cells in any tissue of the body will not survive prolonged periods of oxygen deprivation. In oxygen deprived heart muscle (myocardium) cells shift from oxidative phosphorylation to anaerobic glycolysis. The amount of available ATP drops, and cellular pH rises. Contractile forces are reduced, and energy dependent ion pumps are impaired. Myocardial cells (and others, too) respond to brief periods of oxygen deprivation by adjusting their cellular processes, and as a result are be able to tolerate longer periods of poor oxygen conditions. This kind of response is known as ischemic preconditioning (IP). The key signaling events responsible for IP are sketched in Figure 8.14. As can be seen in the figure, signaling starts with release of adenosine by stressed cells resulting in their binding to adenosine receptors, members of the G-protein coupled receptor (GPCR) family. The heterotrimeric G proteins activated by these receptors stimulate the hydrolysis of PtdIns(4,5)P2 to DAG and IP3; the DAG acting as a cofactor for e and d members of the nPKC subfamily, which are anchored nearby the receptors along the plasma membrane, stimulates their activation.
Once activated the PKC e and d proteins change their cellular location, translocating from the plasma membrane to the mitochondria. They become anchored there, and associate with and activate members of the Src family of protein tyrosine kinases such as Lck leading to activation of a
MAP kinase cascade. The downstream PKC e-signaling targets are the mitochondrial ATP-dependent potassium channel proteins, which are phosphorylated by the last MAP kinase in the cascade. The PKC e and d have opposing effects. While PKC e promotes preconditioning responses, PKC d stimulates proapoptotic responses. The cellular response to oxygen deprivation will depend at least in part on the balance between these two signals.
Anchoring proteins such as the RACKs help localize the PKC isozymes in their proper locations. This aspect is illustrated in Figure 8.14 where several RACKs are depicted localizing the PKCs to the plasma membrane, mitochondria, and nucleus. At the plasma membrane the RACKs position the kinase near its substrate—cardiac L-type calcium channels. In the nucleus, the RACKs serve a similar role, placing the kinase near transcription factors that it regulates by means of phosphorylation. The RACKs not only help localize the proteins near their substrates, but also help organize signaling complexes. This aspect is represented in the figure by the binding of Src/Lck along with PKC e to the mitochondrial RACK.
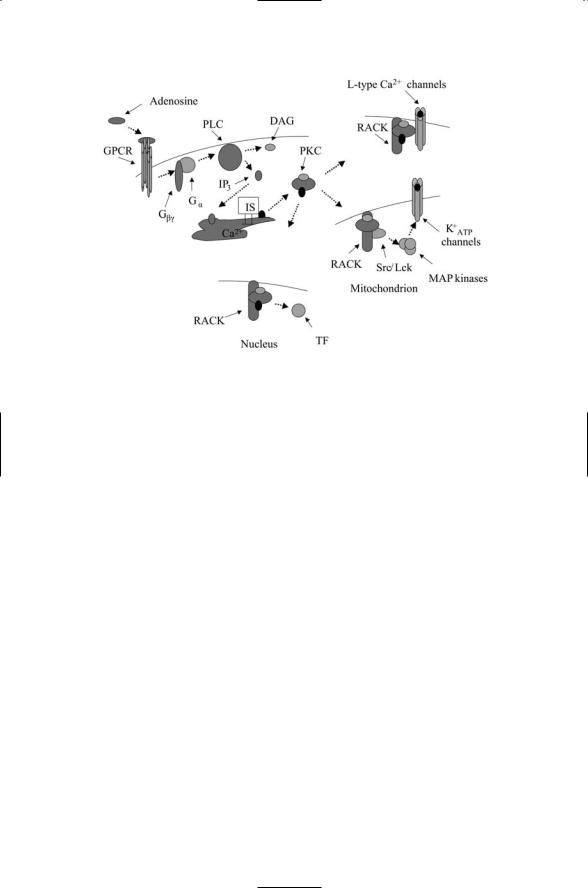
180 8. Organization of Signal Complexes by Lipids, Calcium, & Cyclic AMP
FIGURE 8.14. Protein kinase C signaling in cardiac cells: Signaling is initiated by binding of adenosine to a G protein-coupled receptor (GPCR) resulting in dissociation of the G protein tethered nearby into its Ga and Gbg subunits. The Ga subunit activates phospholipase C, leading to formation of IP3 and DAG second messengers. IP3 triggers the release of calcium from intracellular stores (IS). Calcium and DAG activate cPKCs and DAG activates nPKCs. The RACKs position these protein kinases near their substrates along the plasma membrane and in the mitochondria and nucleus. (To keep the figure from getting too cluttered, phosphorylation of the PKCs by upstream kinases is not shown.)
8.17cAMP Activates PKA, Which Regulates Ion Channel Activities
Cyclic AMP is a second messenger that acts through cAMP-dependent protein kinase A (PKA) to form the cAMP signaling pathway. This pathway is used to relay a variety of hormonal and neuromodulatory signals to the transcription machinery in the nucleus, to ion channels embedded in the plasma membrane, and to other targets such as the actin cytoskeleton. The cAMP signaling pathway consists of a receptor such as a GPCR, an intermediary such as a G protein, adenylyl cyclases and phosphodiesterases that form and regulate cAMP production, and protein kinase A. In more detail, ligand binding to a GPCR activates a G protein (the intermediary) tethered to the cytoplasmic region of the plasma membrane close enough to the receptor to be activated by its cytoplasmic region. Once activated, the G protein translocates to and stimulates the production of cAMP by adenylyl cyclases. The cAMP molecule, in turn, binds to and activates protein kinase A.
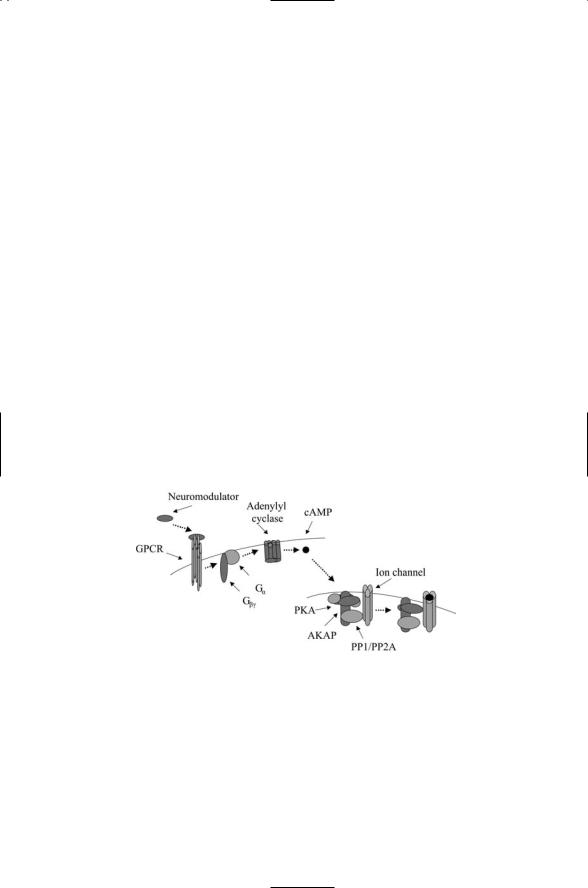
8.17 cAMP Activates PKA, Which Regulates Ion Channel Activities |
181 |
Protein kinase A contains a pair of catalytic (C) subuints and a pair of regulatory (R) subunits. In its inactive form, the regulatory units bind to the catalytic site and inhibit its activity. Binding of cAMP to the regulatory subunits results in a conformational change that permits the dissociation of the regulatory units form the complex. Once freed of its regulatory subunits, the catalytic subunits are able to catalyze the transfer of phosphoryl groups to their targets using ATP as the phosphoryl group donor. Protein kinase A is able to phosphorylate a number of different proteins. The choice of target is dictated by the choice of regulatory units and by its subcellular location. The specific location in the cell is determined by an AKAP.
The AKAP complexes incorporate not only protein kinases but also protein phosphatases that after a time turn off what the kinases turn on. In Figure 8.15, PKA together with protein phosphatases PP1 or PP2A regulate the opening of an ion channel embedded in the plasma membrane. An AKAP called Yotiao helps regulate the opening and closing of ion channels called NMDA receptors in neurons in the brain. The manner of this is depicted in the figure.
AKAP79 is a well-studied member of the A-kinase anchoring protein family. This protein binds two AGC kinases, protein kinase A and protein kinase C along with a prominent serine/threonine protein phosphatase called calcineurin (CaN), or as it is sometimes known, protein phosphatase 2B (PP2B). These enzymes are capable of binding many different substrates
FIGURE 8.15. PKA signaling through AKAPs regulating ion channel openings: Binding of a neuromodulator to a G protein-coupled receptor initiates signaling in the neuron. The activated Ga subunit stimulates production of cAMP by adenylyl cyclase. The cAMP molecules bind to the regulatory subunits of protein kinase A. In response, the regulatory subunits dissociate from the catalytic subunits, which then phosphorylate the ion channel, overcoming its inhibition (closed state) by the protein phosphatases. After a time, the protein kinases are no longer able to maintain the channel in its phosphorylated state and the ion channel closes again (not shown).
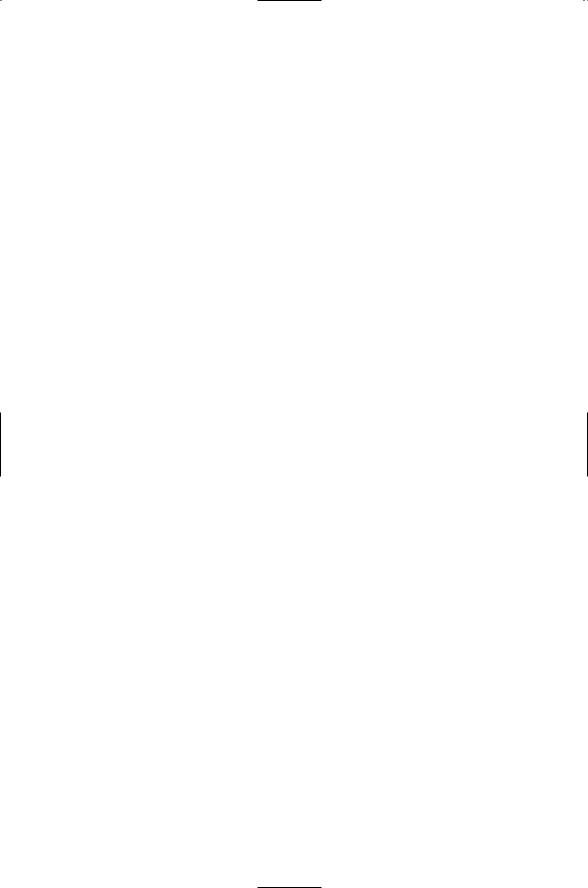
182 8. Organization of Signal Complexes by Lipids, Calcium, & Cyclic AMP
with high affinity. The desirable property of high affinity is combined with another important property, substrate specificity, through use of an AKAP. The AKAP79 situates the three signaling proteins near their targets: plasma membrane receptors and ion channels. The enzymatic functions of these proteins are disabled by their association with the anchoring protein. Ca2+/CaM supplies the “on” signal for these enzymes. When this complex binds protein kinase C and calcineurin, the enzymes detach, and diffuse to and phosphorylate/dephosphorylate their nearby targets. Similarly, cAMP binding will enable protein kinase A to detach from the AKAP79 and phosphorylate its targets.
In the brain, protein kinase A participates in the “learning pathway” along with several other protein kinases. The main endpoint of the learning pathway is the nucleus where kinases functioning as transcription factors regulate gene expression. Signaling interactions of a short term nature involving the regulation of ion channels, and long term natureproducing changes in gene expression, are central to brain function. They will be examined in Chapter 21.
8.18PKs Facilitate the Transfer of Phosphoryl Groups from ATPs to Substrates
The core unit of protein kinase A is representative of the core units of all serine, threonine, and tyrosine kinases. As shown in Figure 8.16, it consists of a small lobe, a linker, and a large lobe. A cleft formed by elements of the small and large lobes operates as the catalytic site. The large lobe binds the substrate peptide or protein, while the small lobe supplies the main site for attachment of the ATP molecule. The ATP molecule sits at the base of the cleft and provides structural support, helping to fix and maintain the orientations of the large and small lobes with respect to one another. The cleft is bordered by the glycine-rich loop and the C helix from the small lobe and by a beta sheet from the large lobe. As shown in Figure 8.16, the phosphoryl groups attached to the adenosine are oriented towards the cleft with the gamma phosphoryl group at the end. The loops and beta sheet position this group for transfer to the substrate.
A common feature in protein kinases is the presence of metal ions. As was the case for the histidine kinases discussed in the last chapter, these positively charged ions help position and stabilize the cell’s assembly. The Mg ion-positioning loop highlighted in the figure assists in positioning the magnesium ions.
There are two phosphorylation sites on the core unit—Thr197 and Ser338. In the active state conformation, the cleft is opened up and the core unit is catalytically competent. Phosphorylation at Thr197 and Ser338 helps stabilize the open conformation.
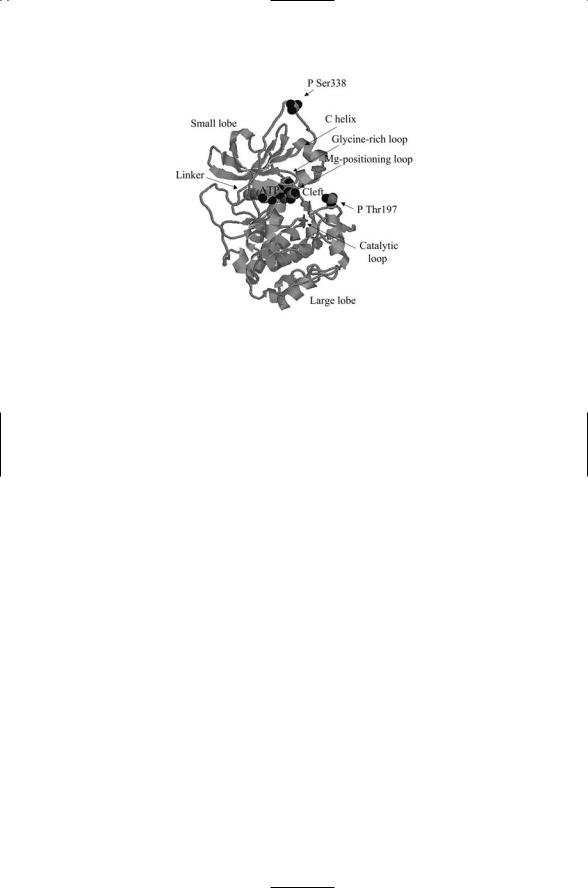
References and Further Reading |
183 |
FIGURE 8.16. Crystal structure of the conserved core of the catalytic subunit of protein kinase A: The core unit consists of a small lobe, a linker and a large lobe. The small lobe contains a five-stranded antiparallel beta sheet and a conserved helix (the C helix). The large lobe consists mainly of helices plus a grouping of four beta strands at the bottom of the active site cleft. The phosphoryl groups covalently attached at Thr197 and Ser338 are depicted as space-filled model. The ATP molecule is also shown with a space-filled representation. The figure was prepared using Protein Explorer with atomic coordinates deposited in the Protein Data Bank (PDB) under accession code 1ATP.
References and Further Reading
Plasma Membrane Organization
Brown DA, and London E [1998]. Structure and origin of ordered lipid domains in biological membranes. J. Mem. Biol., 164: 103–114.
Sheets ED, Holowka D, and Baird B [1999]. Critical role for cholesterol in Lynmediated tyrosine phosphorylation of FceRI and their association with detergentresistant membranes. J. Cell Biol., 145: 877–887.
Simons K, and Ikonen E [2000]. How cells handle cholesterol. Science, 290: 1721– 1726.
Simons K, and Toomre D [2000]. Lipid rafts and signal transduction. Nature Rev. Mol. Cell Biol., 1: 31–41.
Smart EJ, et al. [1999]. Caveolins, liquid-ordered domains, and signal transduction. Mol. Cell Biol., 19: 7289–7304.
Vereb G, et al. [2003]. Dynamic, yet structured: The cell membrane three decades after the Singer–Nicolson model. Proc. Natl. Acad. Sci. USA 100: 80553–80558.
Lipid Signaling, Phosphoinositide-3-OH Kinase, and PIP2 Signaling
Honda A, et al. [1999]. Phosphatidylinositol 4-biphosphate kinase a is a downstream effector of the small G protein ARF6 in membrane ruffle formation. Cell, 99: 521–532.
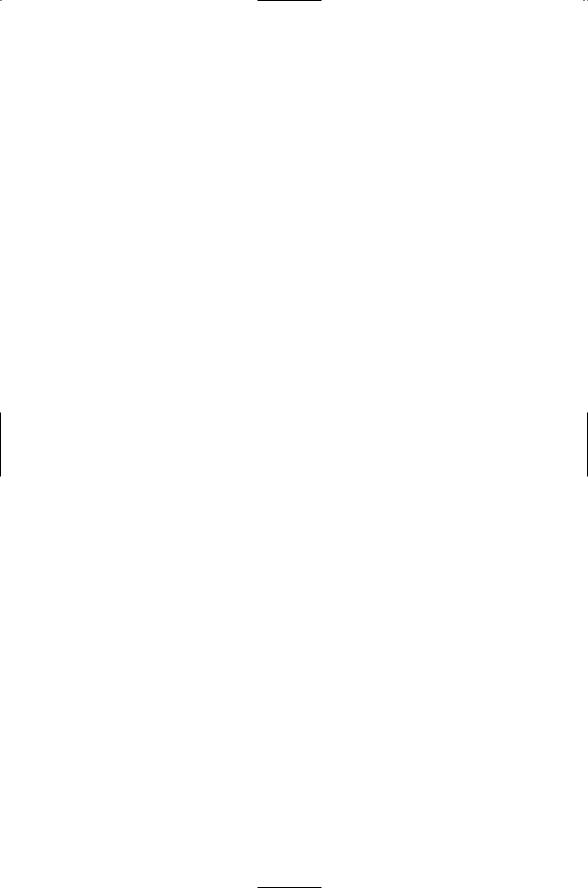
184 8. Organization of Signal Complexes by Lipids, Calcium, & Cyclic AMP
Raucher D, et al. [2000]. Phosphatidylinositol 4,5-biphosphate functions as a second messenger that regulates cytoskeleton-plasma membrane adhesion. Cell, 100: 221–228.
Toker A, and Cantley LC [1997]. Signaling through the lipid products of phosphoinositide-3-OH-kinase. Nature, 387: 673–676.
Vanhaesebroeck B, and Waterfield MD [1999]. Signaling by distinct classes of phosphoinositide 3-kinases. Exp. Cell Res., 253: 239–254.
Lipids and Lipid-Binding Domains
Czech MP [2000]. PIP2 and PIP3: Complex roles at the cell surface. Cell, 100: 603– 606.
Hurley JH, and Meyer T [2001]. Subcellular targeting by membrane lipids. Curr. Opin. Cell Biol., 13: 146–152.
Lemmon MA, and Ferguson KM [2000]. Signal-dependent membrane targeting by Pleckstrin homology domains. Biochem. J., 350: 1–18.
Calcium/Calmodulin
Berridge MJ, Lipp P, and Bootman MD [2000]. The versatility and universality of calcium signaling. Nature Revs. Mol. Cell Biol., 1: 11–21.
Carafoli E [2002]. Calcium signaling: A tale for all seasons. Proc. Natl. Acad. Sci. USA, 99: 1115–1122.
Chin D, and Means AR [2000]. Calmodulin: A prototypic calcium sensor. Trends Cell Biol., 10: 322–328.
Adenylyl Cyclases
Cooper DMF, Mons N, and Karpen JW [1995]. Adenylyl cyclases and the interaction between calcium and cAMP signaling. Nature, 374: 421–424.
Hurley JH [1999]. Structure, mechanism, and regulation of mammalian adenylyl cyclase. J. Biol. Chem., 274: 7599–7602.
Taussig R, and Gilman AG [1995]. Mammalian membrane-bound adenylyl cyclases,
J. Biol. Chem., 270: 1–4.
Cyclic AMP
Houslay MD, and Milligan G [1997]. Tailoring cAMP-signaling responses through isoform multiplicity. Trends Biochem. Sci., 22: 217–224.
Rich TC, et al. [2001]. A uniform extracellular stimulus triggers distinct cAMP signals in different compartments of a simple cell. Proc. Natl. Acad. Sci. USA, 98: 13049–13054.
Schwartz JH [2001]. The many dimensions of cAMP signaling. Proc. Natl. Acad. Sci. USA, 98: 13482–13484.
Cyclic Nucleotide Phosphodiesterases
Beavo JA [1995]. Cyclic nucleotide phosphodiesterases: Functional implications of multiple isoforms. Physiol. Rev., 75: 725–748.
Francis SH, Turko IV, and Corbin JD [2001]. Cyclic nucleotide phosphodiesterases: Relating structure and function. Prog. Nucl. Acid Res. Mol. Biol., 65: 1–52.