
Molecular and Cellular Signaling - Martin Beckerman
.pdf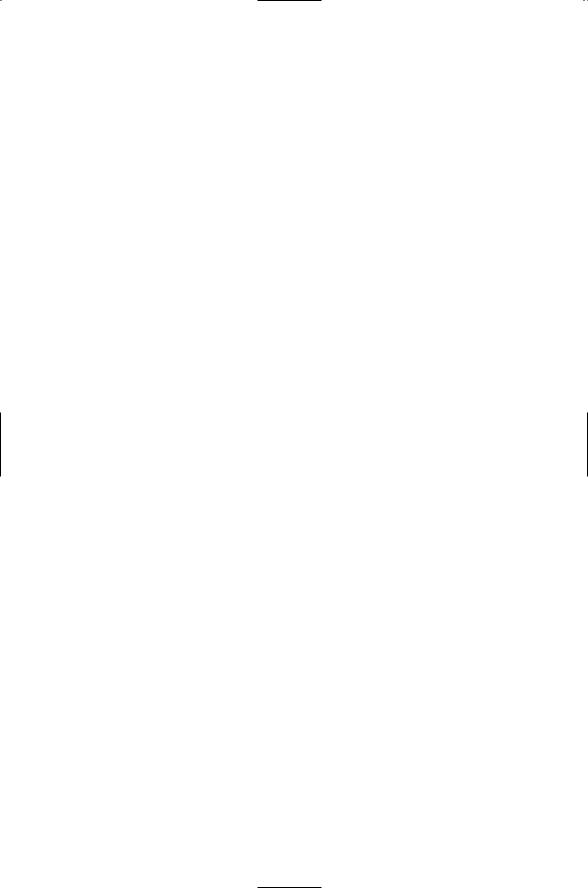
124 6. Stress and Pheromone Responses in Yeast
ATP as a donor. Protein phosphatases do the opposite. They catalyze the removal of phosphoryl groups using ADP as an acceptor.
Like the protein kinases, the protein phosphatases fall into two main groups—those that catalze the removal of phosphoryl groups from serine/threonine residues, and those that do the same on tyrosine residues. Eukaryotic genomes encode a smaller number of protein phosphatases than protein kinases. The serine/threonine phosphatases can be placed into two families—PPP and PPM—and the tyrosine phosphatases are placed into one large familty designated as PTP. The best studied of the serine/threonine phosphatases are the members of the PP1 and PP2 families. These proteins possess nearly identical catalytic subunits, and it is the regulatory units that provide substrate specificity. Each phosphatase family member can associate with any of a number of different regulatory units.
6.12Scaffold and Anchor Protein Role in Signaling and Specificity
Scaffold and anchor proteins help organize the signaling pathway and confer specificity. Spatial localization is a powerful way of ensuring that protein kinases only phosphorylate the correct substrates. While cytosolic proteins are, in principle, free to diffuse about in the cytosol, they generally do not do so. Instead, proteins are restricted to specific locations in the cell, either in particular organelles or in specific subcellular compartments, that is, in restricted regions of space in the cytosol. The restriction of signaling molecules to specific locations in the cell is known as spatial localization or compartmentalization.
Scaffold and anchor proteins promote spatial localization and substrate specificity. These proteins do not have any enzymatic activity but rather serve as platforms that help organize the signaling pathways. The scaffold and anchor proteins (Figure 6.2) provide binding sites for attachment of the kinases and their substrates at specific locales in the cell. Scaffolding proteins were first discovered in the MAP kinase pathways of yeast cells, and then in the mammalian MAP kinase pathways as well. Since the initial discoveries, several different kinds of scaffold proteins have been identified in nerve cells, where they play a prominent role in organizing the signaling machinery.
Anchor proteins are similar to scaffold proteins in that they too help organize signaling routes, but unlike scaffolds are attached, or anchored, to the cytoplasmic face of the plasma membrane. They sequester signaling proteins in either active or inactive states, ready for activation or deactivation, positioned close to their substrates that are usually other plasma mem- brane-associated signaling proteins. These too are prominent components of the signaling machinery in nerve cells.
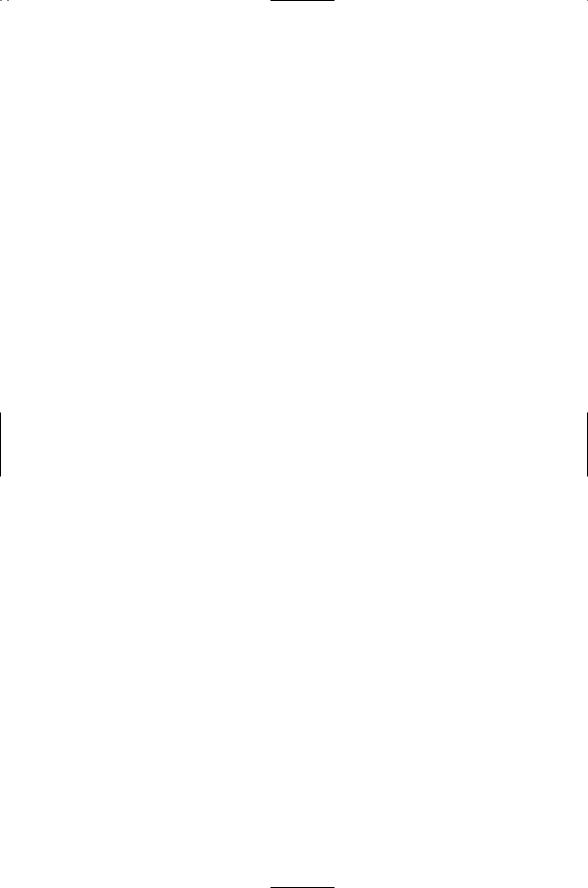
6.14 Pheromone Response Pathway Is Activated by Pheromones |
125 |
6.13 GTPases Regulate Protein Trafficking in the Cell
The transcription machinery resides in the cell nucleus while the translation machinery is located in the cytosol. Among the major consequences of this compartmentalization is the addition of control points that regulate nucleocytoplasmic transport. In order for a transcription factor to influence transcription it must be located in the nucleus. If it is kept out of the nucleus then it cannot act. Similarly, a signaling molecule whose target lies in the cytosol cannot act on that molecule if its location is restricted to the nucleus. Spatial localization and sequestering is another way of regulating kinase actions so that they only act on the appropriate targets at the right time. A hallmark of this sort of regulation is its dynamic character. In response to regulatory signals, some kinases may migrate from the nucleus into the cytosol while others translocate in the opposite direction from the cytosol into the nucleus.
GTPases play a crucial role in regulating protein traffic in a cell. These are a large family of GTP-binding proteins that function as molecular switches, operating at key control points in the cell. Members of this family of control elements are involved in shuttling proteins in and out of the nucleus. Other family members regulate the movement of cargo throughout the cell, and especially from organelles in the interior to the surface and back, and still others regulate the organization of the actin cytoskeleton. GTPases along with a variety of small adapter molecules are often concentrated at and just below the plasma membrane, where they help organize the intracellular signal-transducing pathways leading to the various control points that serve as interfaces to the fixed infrastructure and mediate the cellular responses to the signals.
6.14Pheromone Response Pathway Is Activated by Pheromones
Yeasts respond to mating pheromones and to stresses by altering their patterns of gene expression. Environmental and pheromone signals are sent from the plasma membrane to the nucleus through a set of parallel pathways named for the last in a series of protein kinases that serve as the central signal transducers. The kinases that lend their name to the pathways are mitogen-activated protein (MAP) kinases, members of the CMGC group of serine/threonine kinases. Six of these pathways have been found in yeasts and three in mammals.
One of the best-characterized pathways, the pheromone response pathway, is diagrammed in Figure 6.7. As depicted in the figure, this pathway contains a kinase core that sits between the receptor and associated signal transduction machinery positioned at the plasma membrane and down-
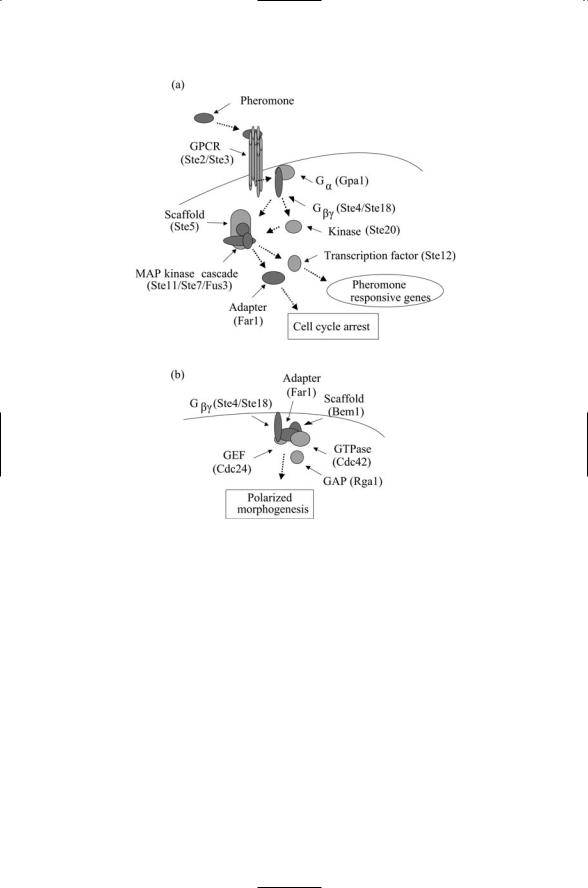
126 6. Stress and Pheromone Responses in Yeast
FIGURE 6.7. Pheromone response pathway: (a) Pheromones initiate signaling when they attach to the extracellular ligand-binding portion of the G protein-coupled receptor (GPCR). Receptor-binding triggers dissociation and activation of the Ga and Gbg subunits of the G-protein. The Gbg subunit activates the Ste20 kinase and interacts with the Ste5 scaffold protein resulting in activation of signaling through a MAP kinase cascade, leading to activation of Ste12 and the subsequent transcription of genes responsive to pheromone signals, and of Far1, a mediator of cell cycle arrest (in G1). (b) Far1 is an adapter protein and serves as an adapter between Gbg and the Cdc24 GEF at the plasma membrane.
stream elements that make contact with the transcription machinery to influence the program of gene transcription. The transmission of a signal from the extracellular side of the plasma membrane into the cell interior and from there to the cell nucleus involves the execution of several activities, or processes, each designed to enhance the specificity, or fidelity, of the signaling. These are: transmembrane signal transduction; nucleocytoplasmic
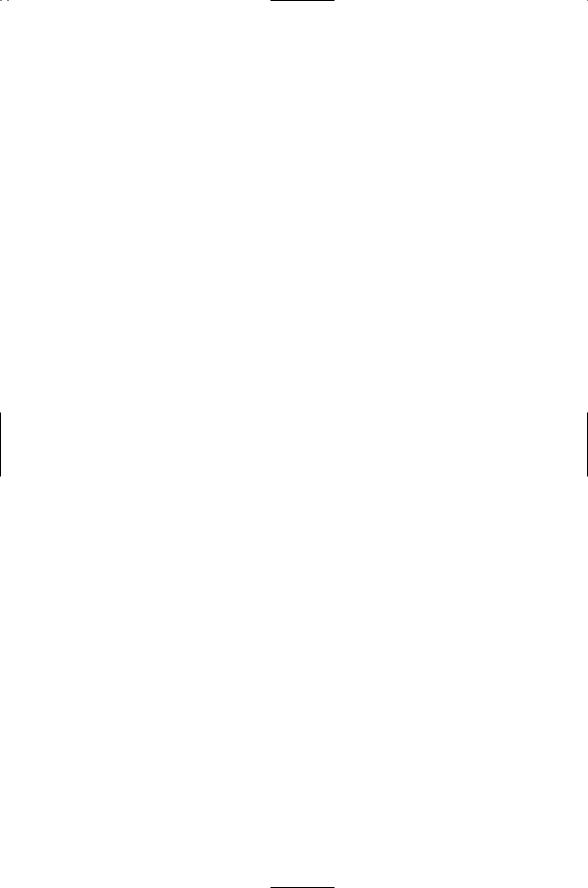
6.14 Pheromone Response Pathway Is Activated by Pheromones |
127 |
shuttling; and recruitment to the cytosolic face, leading to assembly and activation of the kinase core unit.
Membrane-spanning receptors bind ligands and send signals indicative of the binding event across the plasma membrane to the cytoplasmic surface. In the pheromone pathway, members of the G protein-coupled receptor (GPCR) family perform this task. The GPCR family is a prominent component of the human genome with roughly 600 members identified so far. They serve as sensors for physical and chemical signals such as light and odors, and for a variety of cell-to-cell, hormonal and neural signals. These receptors and their methods of action will be explored in detail in Chapter 12. In brief, when a ligand binds a G protein-coupled receptor, it produces changes in the electrostatic environment of the cytoplasmic portions of the receptor. As its name indicates, a GPCR acts through a G protein to which it is coupled, or tethered. The G proteins are built from three distinct subunits, called alpha, beta, and gamma, and so are referred to as being heterotrimeric. The changes in the cytoplasmic portions of the GPCR cause the dissociation of the G protein alpha subunit from the beta and gamma subunits, allowing the subunits to move about and activate other signaling molecules.
The set of actions that follow signal reception and conveyance across the plasma membrane may be collectively termed recruitment and pathway formation. These activities occur at and just below the plasma membrane. In the yeast pheromone pathway, the recruitment of two proteins, Ste20 and Ste5, is promoted primarily by the activated G protein beta subunit. Ste5 continually shuttles between the cytoplasm and nucleus, and in response to activation of G protein beta subunits, Ste5 starts to accumulate just below the cell surface. The binding by the G protein beta subunit to Ste5 and Ste20, the latter a protein concentrated just below the cell surface, is the crucial step in forming the kinase core. (“Ste” is an abbreviation for the term “sterile,” assigned to proteins whose mutated forms produce nonmating, i.e., sterile phenotypes in the yeast.)
In the pheromone response pathway the kinase core consists of three kinases, Ste11, Ste7, and Fus3, organized in a linear fashion with one signaling to the next in the chain. The Ste5 protein functions as a molecule scaffold for the formation of the kinase module. The protein organizes the kinases into a signaling pathway leading to the activation of the last member of the module. This assembly step takes place subsequent to binding to the G protein beta subunit of Ste20, which phosphorylates and activates Ste11, and to activation of Ste5, which interacts with all members of the MAP kinase module.
The Fus3 MAP kinase functions as the output unit from the kinase module. In the absence of pheromone signals, this protein, like the Ste5 scaffold, continually shuttles between nucleus and cytoplasm. (The other members of the module, Ste7 and Ste11, are cytoplasmic proteins.) In the presence of pheromone signals, the Fus3 protein assembles into the MAP
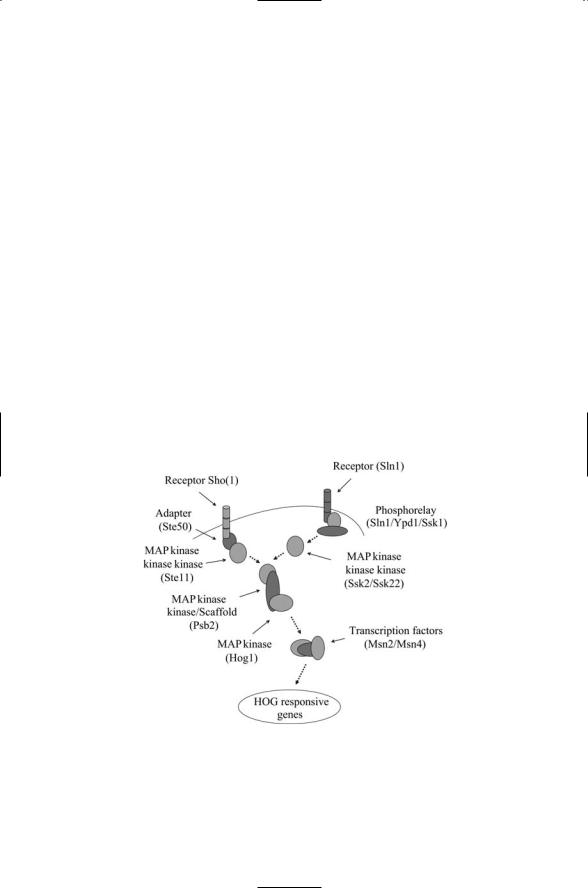
128 6. Stress and Pheromone Responses in Yeast
kinase module, along with Ste11 and Ste7, and is activated. It then translocates back to the nucleus. There it activates the transcription factors Ste12.
The Fus3 MAP kinase also phosphorylates and thus activates the Far1 adapter protein. This protein has several functions. It is a mediator of cell cycle arrest (in G1) as indicated in Figure 5.7. Like Ste5, it shuttles between different subcellular locations. It shuttles the Cdc24 GEF to the plasma membrane and mediates formation of a complex containing several proteins at the Gbg location where the pheromone signals are strongest. This action makes the place wherein the cytoskeleton reorients itself in preparation for bud formation.
6.15Osmotic Stresses Activate Glycerol Response Pathway
The high osmolarity glycerol (HOG) response pathway, activated by osmotic stresses, is shown in Figure 6.8. This is another of the yeast stress pathways. It enables the yeast cells to adapt to changes in external osmolarity by altering their patterns of gene expression. In response to elevated
FIGURE 6.8. The high osmolarity glycerol (HOG) response pathway: Two receptors, Sln1 and Sho1, signal through a common MAP kinase cascade and downstream Hog1 and Msn2/Msn4 transcription factors to influence the expression of HOGresponsive genes.
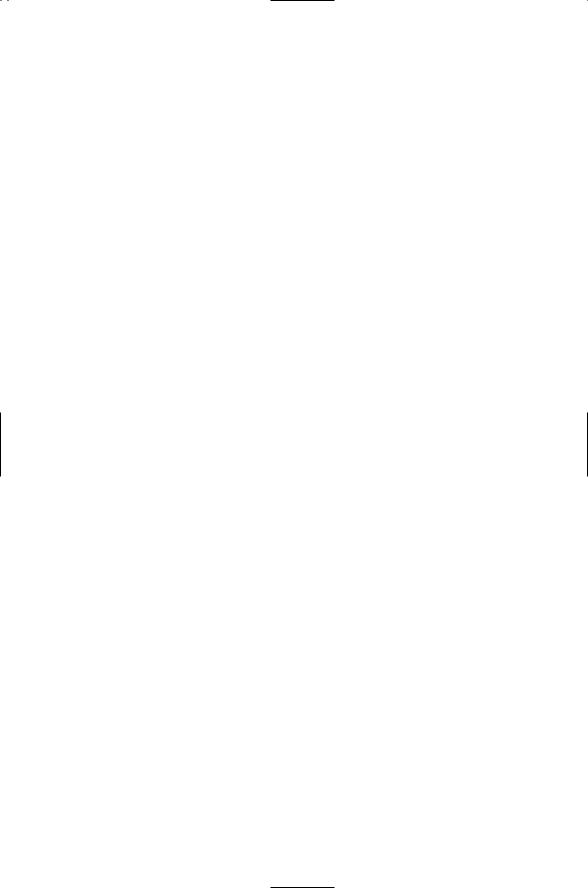
6.16 Yeasts Have a General Stress Response |
129 |
external osmolarity, the yeast cells increase glycerol synthesis and decrease glycerol permeability, thereby increasing their internal osmolarity and restoring a correct osmotic gradient for water uptake.
The HOG pathway has two distinct osmosensors, Sho1 and Sln1. Sho1 and Sln1 (along with Ypd1 and Ssk1) operate in place of the GPCR and associated G proteins that sense and transduce pheromone signals. Both Sho and Sln1 signal through components of the HOG MAP kinase core module resulting in the activation of the Hog1 MAP kinase. The central element in the kinase core, Psb2, doubles both as the kinase kinase and as the scaffold protein for the module. Signals conveyed into the cell through the Sln1 receptor utilize Ssk2 or Ssk22 as the kinase kinase kinase, while Sho1 signals are routed via the Ste11 kinase kinase kinase. The last element in the MAP kinase core, Hog1, like Fus3, shuttles back and forth between the cytoplasm and nucleus. In response to elevated osmotic stress, the kinase is phosphorylated by Psb2 and becomes concentrated in the nucleus along with the Msn2 and Msn4 transcription factors.
The second osmosensor, Sln1, is unusual for a eukaryotic sensor. It is a histidine kinase, a kind of signal protein that is common in bacteria, but is not only uncommon in eukaryotes, but also seems to be absent all together in animals. These systems are often called histidine-aspartate (His-Asp) phosphorelays because they involve the transfer, or relay, of phosphoryl groups between histidine and aspartate residues. The mechanistic details of how His-Asp systems operate in bacterial cells will be a main focus of the next chapter.
6.16 Yeasts Have a General Stress Response
The term general stress response was coined to describe the expression of a common set of genes by a number of different stress conditions. Roughly speaking, the same set of genes was observed to be upregulated in response to unfavorable temperature shifts, osmotic shock, starvation conditions, and DNA damage. Among the proteins upregulated in response to these conditions are those such as heat shock proteins that confer general protection against the stresses. The stimulation of gene transcription of a specific set of genes is associated with the presence in the promoters of each of these genes of a stress responsive element, or STRE.
The pathways leading to the transcription initiation sites located in the nucleus start with a sensing activity taking place in the outer reaches of the yeast cell. These activities are most often performed by receptors located in the plasma membrane, but may also be initiated by proteins functioning as sensors located near or at the cytoplasmic face of the plasma membrane. As indicated in Figure 6.9 two signaling routes are involved in signaling to the STREs. One involves glucose sensing by a transmembrane receptor, and the other involves sensing of internal stresses such as low pH. The crucial
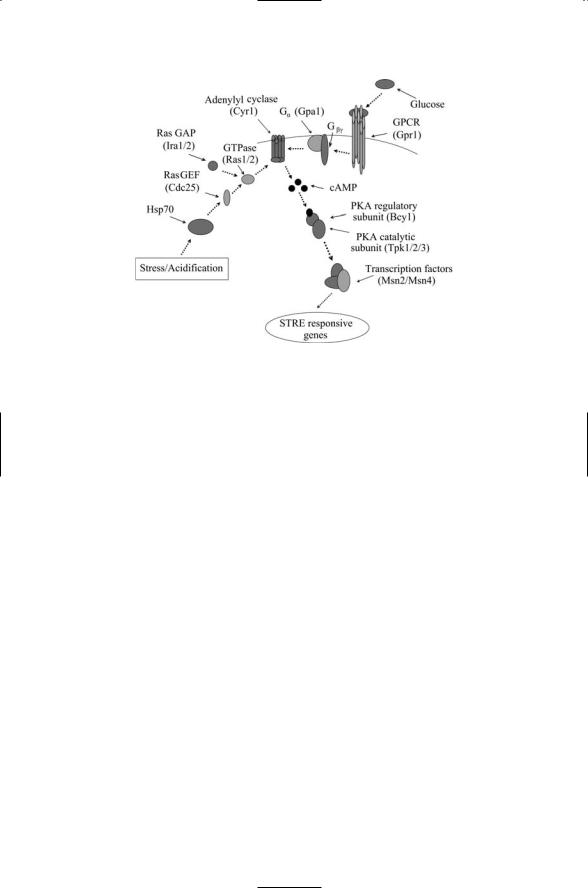
130 6. Stress and Pheromone Responses in Yeast
FIGURE 6.9. General stress response pathway: Protein kinase A, the core signaling element, is stimulated by Ras-mediated internal stress signals and by GPCR/G protein transduced glucose signals, both acting through adenylyl cyclase/cAMP intermediaries.
element in the internal pathway is the heat shock protein Hsp70 introduced in the last chapter. Under low pH conditions the number of denatured proteins increases. The Hsp70 proteins must deal with this situation and are not available to signal to the Ras GEF, Cdc25.
The G protein-coupled receptor (GPCR) functions as a sensor of glucose, the preferred sugar for yeast cells. In response to the presence of glucose it stimulates the production of cAMP by adenylate cyclase. These molecules function as second messengers and have as their cellular role the activation of protein kinase A (PKA). In the yeast this protein has a pair of regulatory subunits and a pair of catalytic subunits. It is activated when two cAMP molecules bind to each regulatory subunit. In response, the catalytic subunits dissociate from the regulatory subunits and become activated. The glucose-activated pathway leading from a GPCR is not the only path to activation of PKA. Alternatively, Ras may stimulate cAMP production in response to stress conditions, again leading to activation of PKA.
Activated PKA is a negative regulator of Msn2p and Msn4p, transcription factors (TFs) that bind to the STRE. Under good (logarithmic) growth conditions these TFs are localized in the cytoplasm, but migrate to the nucleus when glucose conditions are degraded. The import and export of Msn2p and Msn4p from the nucleus is regulated by PKA activity and also independently by signals from the TOR pathway (to be discussed next). The
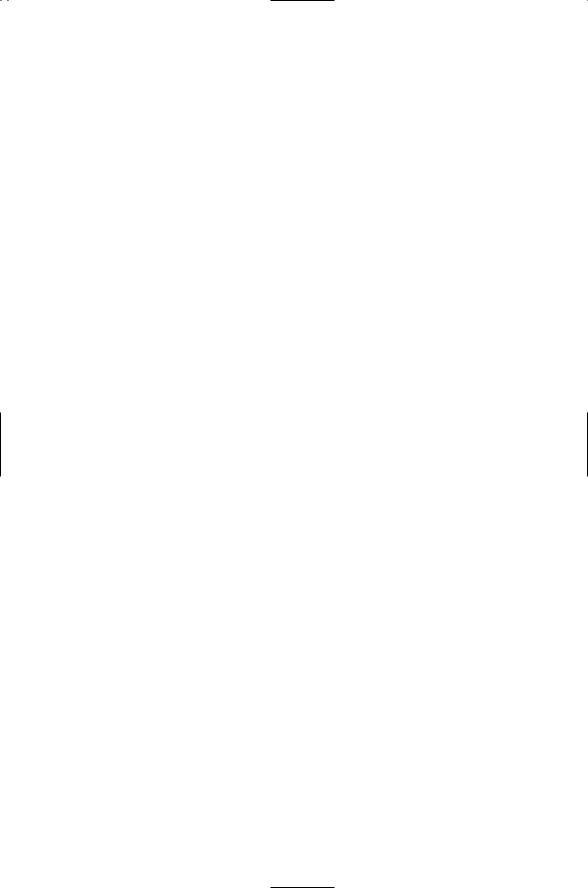
6.17 Target of Rapamycin (TOR) Adjusts Protein Synthesis |
131 |
overall mechanism is that when growth conditions are poor and the cell is subjected to stressful conditions the Msn2p and Msn4p proteins accumulate in the nucleus, bind to the STREs, and stimulate transcription of genes whose protein products protect the cell.
6.17Target of Rapamycin (TOR) Adjusts Protein Synthesis
TOR adjusts protein synthesis in accordance with growth conditions. The process of growth is distinct from that of proliferation, although the latter often follows from the former. Cell growth refers to the increase in cell size and mass and is driven by rapid protein synthesis. Cell proliferation is the result of the progression through the cell cycle resulting in cell division. Under good growth conditions yeast cells will grow rapidly. The average lifetime of a ribosome is about 100 minutes, and to maintain an optimal rate of growth, yeast cells produce 2000 ribosomes per minute. The rRNA genes account for more than 60% of the total cellular transcription activity. The yeast genome contains 137 ribosomal protein (RP) encoding genes; there are some gene duplications and these genes encode 78 different RPs. Their transcription yields 25% of the total number of mRNA molecules in the cell.
As a large expenditure of resources is needed to maintain this growth machinery, when conditions are no longer favorable to growth, cellular resources must be directed elsewhere. Targets of rapamycin (TOR) proteins are central controllers of cellular resources. They regulate the balance between protein synthesis and protein degradation, throttling back the program of cellular growth in response to reductions in the quality of nitrogen and carbon nutrients. In S. cerevisiae this means shutting down budding, the growth at a particular location on the cell surface that lends the name
“budding yeast” to the organism. TOR proteins carry out their resource management functions by sending out regulatory signals to the translation apparatus and the transcription machinery.
TOR is a serine/threonine kinase belonging to the PIKK family (Table 6.1). It remains in an active state as long as intracellular amino acid concentrations, most notably, that of leucine, are adequate. Under plentiful conditions it phosphorylates and thus activates a protein called Tap42 (Figure 6.10). This protein is a regulator of phosphatase PP2A activity, functioning as a subunit of PP2A and PP2A-like phosphatases. The PP2A phosphatase is composed of three subunits that must come together for the phosphatase to carry out its dephosphorylation actions on its cognate target proteins.
When Tap42 is activated it binds the catalytic subunit of the phosphatase PP2A. This binding event prevents the catalytic subunit from associating with the two other subunits of the phosphatase PP2A and by this means immobilizes the phosphatase in an inactive form. Whenever the quality of
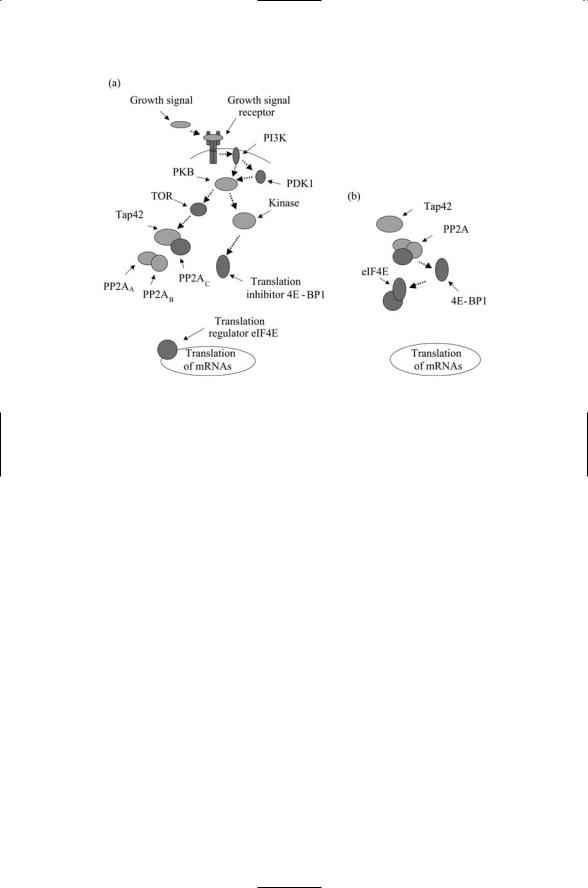
132 6. Stress and Pheromone Responses in Yeast
FIGURE 6.10. Regulation of translation initiation through the TOR signaling pathway: (a) When growth signals are present (good growth conditions) TOR is phosphorylated by upstream kinases such as PKB, and TOR then phosphorylates Tap42. In response Tap42 binds the catalytic PP2A subunit, keeping it away from the other two subunits. The translation inhibitor 4E-BP1 remains in a phosphorylated state and cannot prevent the translation regulator eIF4E from stimulating translation of mRNAs. (b) In the absence of growth signals, the PP2A phosphatase is active and dephosphorylates 4E-BP1, which in turn inhibits eIF4E translation initiation.
carbon and nitrogen nutrients degrades,TOR becomes inactive. It no longer activates Tap42, which, in turn, no longer prevents activation of PP2A. The result of turning off TOR and turning on PP2A is that downstream targets involved in translation initiation and elongation are dephosphorylated and, by these actions, turns down translation of resident mRNAs.
In yeast, TOR proteins match ribosome biogenesis to nutrient avalilabity. Not only do the TOR proteins regulate genes that encode metabolic proteins, but also they regulate genes that encode components of the ribosomes such as the ribosomal proteins mentioned at the beginning of this section.
TOR signaling regulates the transcription of RPs by poly II and also regulates the machinery responsible for transcribing rRNA subunits and tRNAs. End points of TOR signaling include sites at poly I responsible for transcribing the 35S rRNA subunit, and poly III that transcribes the 5S rRNA precursor and tRNAs.
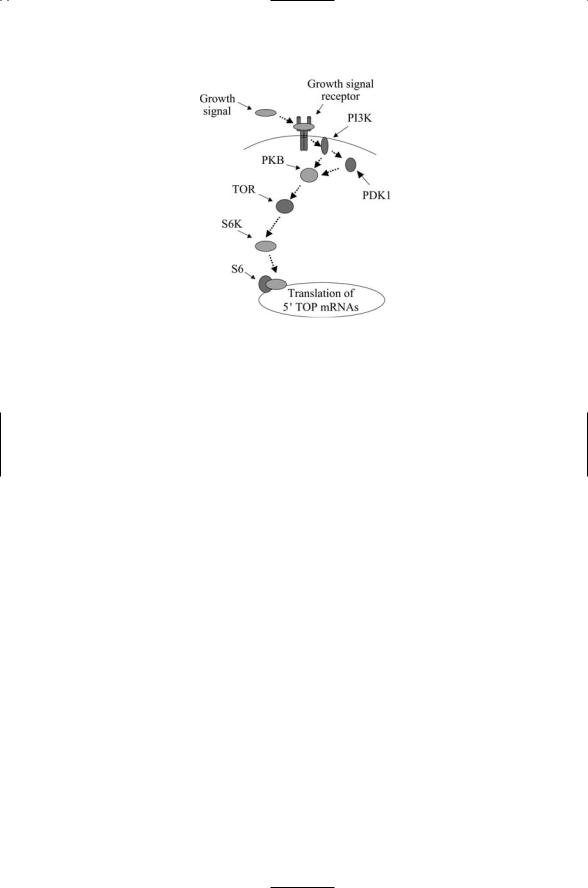
6.18 TOR Adjusts Gene Transcription |
133 |
FIGURE 6.11. Regulation of translation of 5¢TOP genes through the TOR signaling pathway: When growth conditions are favorable TOR is active and phosphorylates the S6K kinase, which in turn phosphorylates the ribosomal factor S6 thereby stimulating transcription of mRNAs bearing a 5¢TOP sequence in their upstream untranslated (regulatory) region (UTR).
When growth conditions are favorable, TOR phosphorylates a serine/threonine kinase called S6K (Figure 6.11). This kinase, a member of the AGC family (Table 6.1), is a key regulator of ribosomal biogenesis. When activated it phosphorylates S6 ribosomal protein resulting in increased translation of mRNAs that encode components of the translation apparatus. The genes encoding these ribosomal proteins are referred to as
5¢TOP genes because they contain a characteristic 5¢TOP (terminal oligopyrimidine tract) sequence in their mRNA regulatory region.
6.18 TOR Adjusts Gene Transcription
TOR adjusts gene transcription in accordance with nutrient conditions. Under poor nutrient conditions, transcription factors are activated that regulate genes encoding proteins involved in metabolism. The specific transcription factors involved in adjustments to poor quality nitrogen supply are the Gln3p and Gat1p, and for poor carbon conditions the transcription factors are Msn2p and Msn4p. The other set of TOR end points, besides beings elements of the translation initiation and elongation regulatory apparatus, are regulators of these transcription factors. In a manner analogous to the immobilization of the catalytic subunit of PP2A, these transcription factors are kept out of the nucleus when growth conditions are