
Molecular and Cellular Signaling - Martin Beckerman
.pdf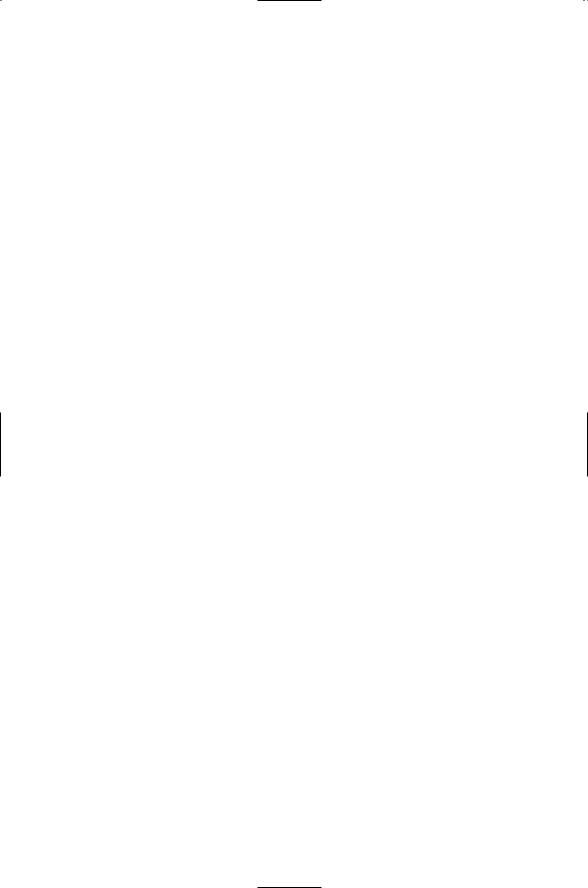
256 11. Signaling in the Endocrine System
phosphothreonines. They are found in kinases, phosphatases, RNA-binding proteins, and especially in nuclear proteins such as transcription factors and proteins involved in detecting and repairing DNA damage.
11.7Modules that Recognize Proline-Rich Sequences Utilized Widely in Signaling Pathways
Proline has several properties that favor its utilization in signaling pathways. First it exhibits a rather limited range of conformations since it alone of the amino acids has a side chain that closes back onto the backbone amino nitrogen atom. This restriction in conformation extends to the residue immediately preceding it and the result is a preference for a particular secondary structure called a polyproline (PP II) helix. This helix has an extended structure with three residues per turn, and the prolines form a continuous hydrophobic strip about the helix surface and also expose several hydrogen binding sites. All in all, the result is an amphipathic structure sometimes referred to as a “sticky arm.” At the same time the binding is relatively weak and complexes so formed are easy to disassemble. Small changes in binding sequence or by phosphorylation produce large changes in the dissociation constant.
SH3,WW, EVH1, and GYF domains all recognize proline-rich sequences, but each recognizes a slightly different sequence or group of sequences. All recognize some variant of the sequence PxxP that forms a left handed PP II helix. The WW domain contains a pair of tryptophans (W) spaced some 20 to 22 amino acids apart and a block of two to four aromatic amino acids situated in between the two tryptophans, hence the name WW domain. There are several kinds of WW domains, each specific for a certain kind of proline-rich sequence. Group IV WW domains recognize sequences containing phosphorylated serine/threonine residues.
11.8Protein–Protein Interaction Domains Utilized Widely in Signaling Pathways
Regulator-of-G protein-signaling (RGS) proteins contain a domain approximately 120 amino acids in length that acts as a G recognition module. It functions as a GAP to accelerate the GTPase activities of Ga subunits of heterotrimeric G proteins coupled to GPCRs. This module has been found in proteins containing other signaling modules such as PDZ domains. The next entry in Table 11.1 following RGS domain is the sterile alpha motif (SAM) domain. This domain forms homoand hetero-oligomers with other domains and is encountered in a variety of signaling proteins. For example, it is found in the C-terminus of all Eph receptors.
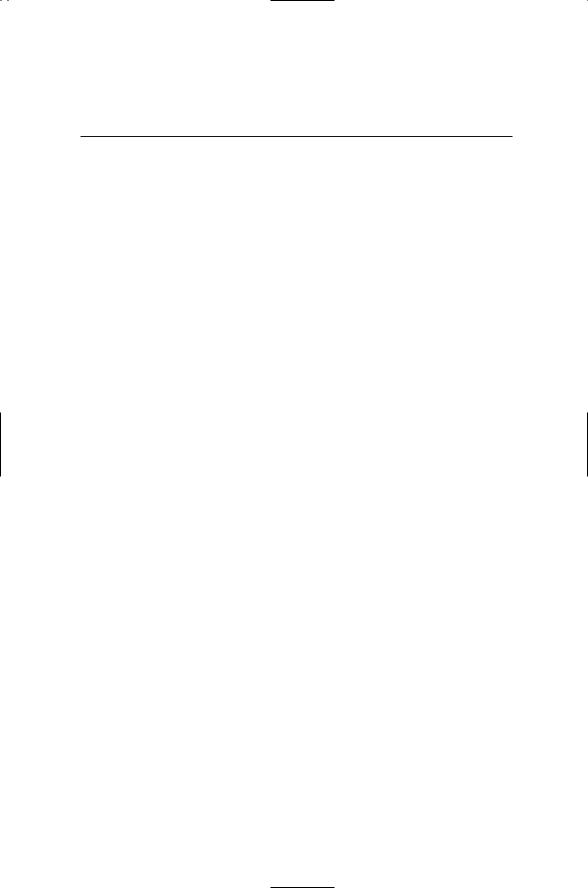
11.8 Protein–Protein Interaction Domains |
257 |
TABLE 11.1. Protein interaction and phosphoprotein recognition modules: Phosphoprotein recognition motifs are denoted using p-Tyr, p-Ser, and p-Thr notation. Proline (P)-based protein interaction motifs are described using single letter codes.
Name of module |
Abbreviation |
Recognition motif(s) |
Src homology-2 |
SH2 |
Phos-Tyr |
Phosphotyrosine binding |
PTB |
Phos-Tyr, NPxY |
Src homology-3 |
SH3 |
PxxP |
WW |
WW |
PPxY; PPLP; P-R; Phos-Ser |
Enabled/vasodilator-stimulated |
EVH1 |
FPPPP |
phosphoprotein homology-1 |
|
|
Gly-Tyr-Phe |
GYF |
PPPPGHR |
14-3-3 |
14-3-3 |
Phos-Ser |
Forkhead-associated |
FHA |
Phos-Thr |
Regulator-of-G protein signaling |
RGS |
Ga |
Sterile a motif |
SAM |
SAM |
Death domain |
DD |
DD |
PSD-95, DLG, ZO-1 |
PDZ |
PDZ, C-terminal motifs |
|
|
|
The next-to-last entry in the table is the death domain (DD). Proteins containing this domain convey death (apoptotis) instructions. The p75NTR receptor shown in Figure 11.1 contains a cytoplasmic death domain (DD). This domain enables cytoplasmic proteins bearing similar death domains to attach via a DD-to-DD linkage. The DD is not the only module of this sort. Instead there is an entire family of six (or seven)-helix bundle death domains that includes the DDs, death effector domains (DEDs), caspase recruitment domains (CARDs), and Pyrin domains (PYDs). Death domains will be discussed further in the chapter on apoptosis.
The final entry in the table is for the PDZ domain, named for the first three proteins found with this domain—the postsynaptic density protein of 95 kDa (PSD-95) found in postsynaptic terminals of neurons, the Discs Large (DLG) protein of Drosophila, and the zona occludens 1 (ZO-1) protein found in epithelial cells. These domains promote protein-protein interactions through PDZ-PDZ binding and also recognize specific sequences in the C-terminals of target proteins. Proteins containing PDZ domains are especially prominent in synaptic terminals where they combine with other domains to form scaffolding proteins. Members of the Shank family of proteins serve as examples of this theme. As shown in Figure 11.4, Shank proteins contain five domains. Their SH3, PDZ, and proline-rich regions either directly or indirectly bind to the three main kinds of glutamate receptors found in postsynaptic terminals, providing an anchorage for their assembly and clustering. This assembly is anchored to the actin cytoskeleton by intermediates that bind the ankrin repeats in the N- terminus and the proline-rich region in the middle. Finally, the SAM
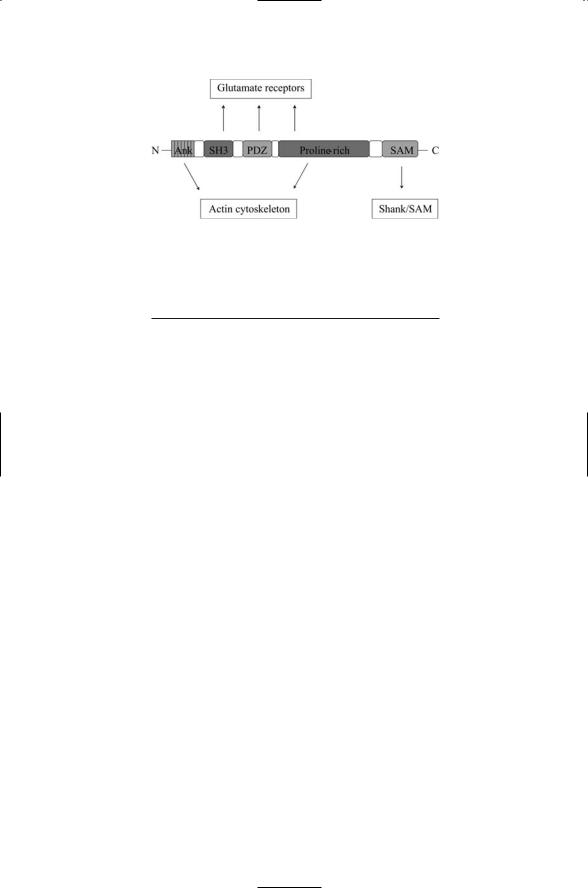
258 11. Signaling in the Endocrine System
FIGURE 11.4. Domain organization of a Shank protein: From the N-terminus to the C-terminus the Shank protein has a set of ankrin repeats (Ank), an SH3 domain, a PDZ domain, proline-rich region, and a SAM domain.
TABLE 11.2. Families of nonreceptor tyrosine kinases.
Family |
Family members |
Abl |
Abl, Ars |
Csk |
Csk, Ctk |
FAK |
CAKb, FAK |
Fes |
Fps (Fes), Fer |
Jak |
JAK1-3, Tyk2 |
Src |
Blk, Fgr, Fyn, Hck, Lck, Lyn, Src, Yes, Yrk |
Syk |
Syk, ZAP70 |
Tec |
Bmx, Btk, Itk/Tsk, Tec, Txk/Rlk |
|
|
domains near the C-terminus promote the linking of one Shank protein to another through SAM-SAM binding.
11.9Non-RTKs Central in Metazoan Signaling Processes and Appear in Many Pathways
Metazoans make extensive use of tyrosine phosphorylation in their signal transduction pathways and in addition to the receptor tyrosine kinases there are several families of nonreceptor tyrosine kinases (NRTKs). These signaling protein tyrosines range in size from 50 to 150 kDa. They are the archtypical examples of modular proteins. In addition to possessing catalytic domains, the NRTKs possess in various combinations phospholipid, phosphotyrosine, and proline-rich sequence recognition domains. Like an RTK, the signaling (catalytic) activity of an NRTK is triggered by tyrosine phosphorylation in the activation loop of the kinase domain. This can happen through autophosphorylation or through phosphorylation by another kinase.
The NRTKs can be grouped into eight families (Table 11.2) according to their catalytic domain sequences, domain composition, and posttransla-
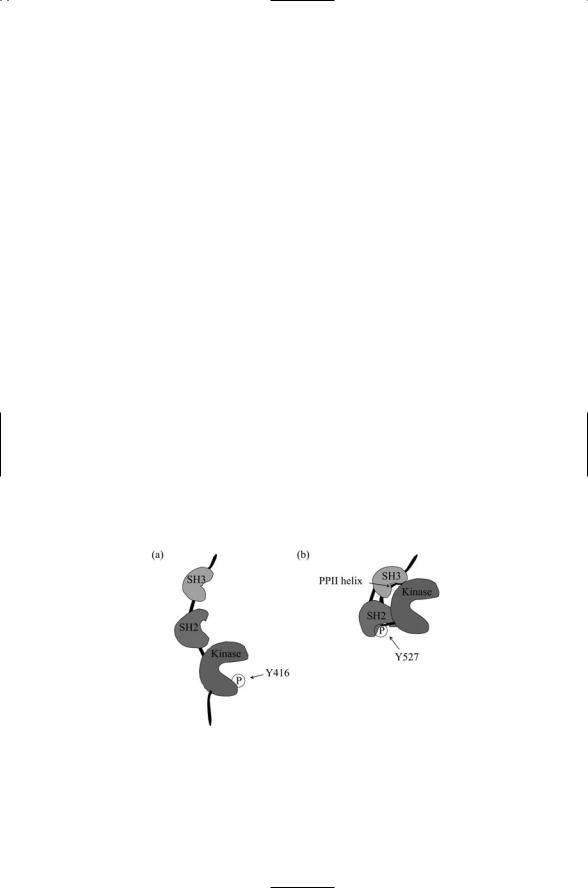
11.10 Src Is a Representative NRTK 259
tional modifications. The Src family of NRTKs is the largest with nine members while several other families have as few as two members. Some NRTKs tether to the cytoplasmic face of the plasma membrane and are covalently modified to permit attachment. Most are cytoplasmic proteins, but Abl family members possess a nuclear localization signal (NLS) and are found both in the cytoplasm and in the nucleus.
11.10 Src Is a Representative NRTK
The Src family is representative of the NRTKs and the discussion will mostly focus on this family and on focal adhesion kinase (next section). The organization of the catalytic domain of Src follows the general pattern discussed above for the RTKs. The catalytic domain is bilobed with an active site cleft and an activation loop containing a critical tyrosine residue. Phosphorylation of this tyrosine activates the kinase domain. The overall structure of Src is as follows. The N-terminal region contains a myristylation site and sometimes a palmitoylation site. An SH3 domain, an SH2 domain, the catalytic domain, and finally the COOH terminal region containing a second critical tyrosine residue follow initial segment. Phosphorylation of the tyrosine (Tyr527) in the COOH tail by regulatory proteins such as Csk deactivates the protein kinase.
The SH2 and SH3 domains are located on the backside of the catalytic domain of Src and do not impede activation and catalysis (Figure 11.5). The SH2 domain of Src, as well as the SH2 domains of Fyn, Lck, and Fgr, select peptide sequences of the form pYEEI; but they also bind the sequence pYAEI of FAK and other similar sequences in other proteins. The SH3
FIGURE 11.5. The Src protein in open and closed conformations: (a) The Src protein is in an open conformation in which a crucial tyrosine residue in the kinase domain is exposed and phosphporylated. (b) The site of tyrosine phosphorylation in the kinase domain is blocked and the binding surfaces of the SH2 and SH3 domains are sequestered.
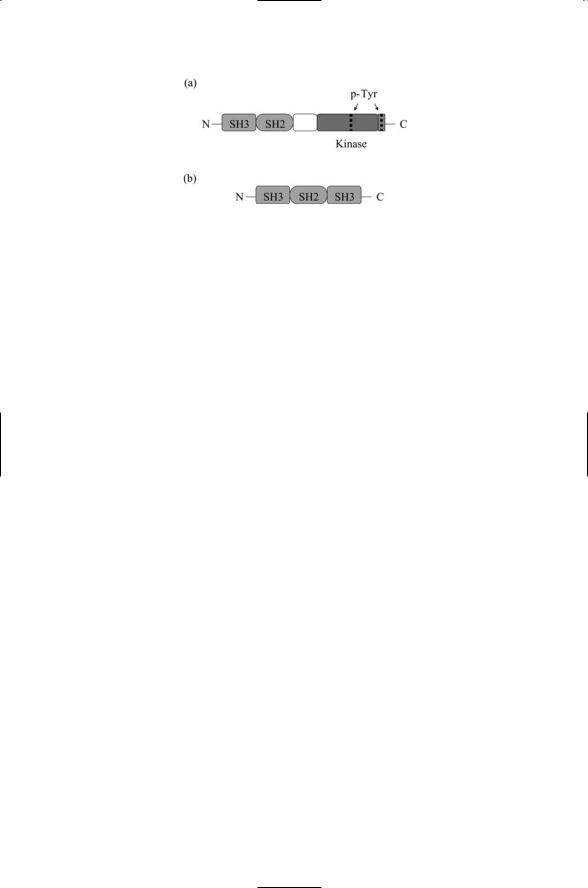
260 11. Signaling in the Endocrine System
FIGURE 11.6. SH2and SH3-bearing proteins: (a) Organization of c-Src nonreceptor tyrosine kinase. (b) Organization of the Grb2 adapter protein. Proteins such as Grb2 that lack domains that carry out enzymatic activities are referred to as adapters. If they are very large and can bind multiple proteins they are called scaffolds. Sites where the protein can be phosphorylated on tyrosine residues are labeled by the abbreviation p-Tyr.
domain of Src binds a number of PXXP-like sequences forming left handed PP II helices. Although these two domains are located on the backside of the catalytic domain they cooperate with one another to inhibit Src catalytic activity. In the simplest model of how this occurs Src has two states, an inactive closed conformation and an active open conformation (Figure 11.6). In its closed conformation the SH2 domain binds to pTyr527 in the COOH tail and the SH3 domain binds to a PP II helix in a portion of the linker between SH2 domain and the catalytic domain. These binding events are sufficient to shift the catalytic domain into a conformation where it cannot bind ATP and release ADP, and cannot bind its protein substrate. Thus phosphorylation at Tyr527 by Csk turns off the kinase activity of Src through cooperative autoinhibitory actions of Src’s own SH2 and SH3 domains. The inhibitory interactions are relatively weak, and Src is activated when phosphotyrosineand polyproline-containing sequences in other signal proteins favorably compete for Src SH2 and SH3 binding. Primary activators of Src include PDGF and other RTKs such as EGF, FGF, and NGF. These are not the only transmembrane receptors that signal through Src family members. Other signal pathways linked to these NRTKs include integrins, G protein-coupled receptors, and immune system receptors.
Proliferation is a highly regulated process. For proliferation to occur the appropriate adhesive and growth factor signals must be sent and received. Integrin receptors and receptor tyrosine kinases are localized in the plasma membrane close to one another and together work in signaling growth. The adhesive signals confirm that the cell remains in adhesive contact with the extracellular matrix, and thus growth is permitted. Paxillin, a 68-kDa protein associated with focal adhesions, contains multiple binding sites and serves as a platform for gathering adhesive signals relayed through integrin receptors and growth factor signals sent by receptor tyrosine kinases. Several NRTKs participate in the relay of messages from the transmem-
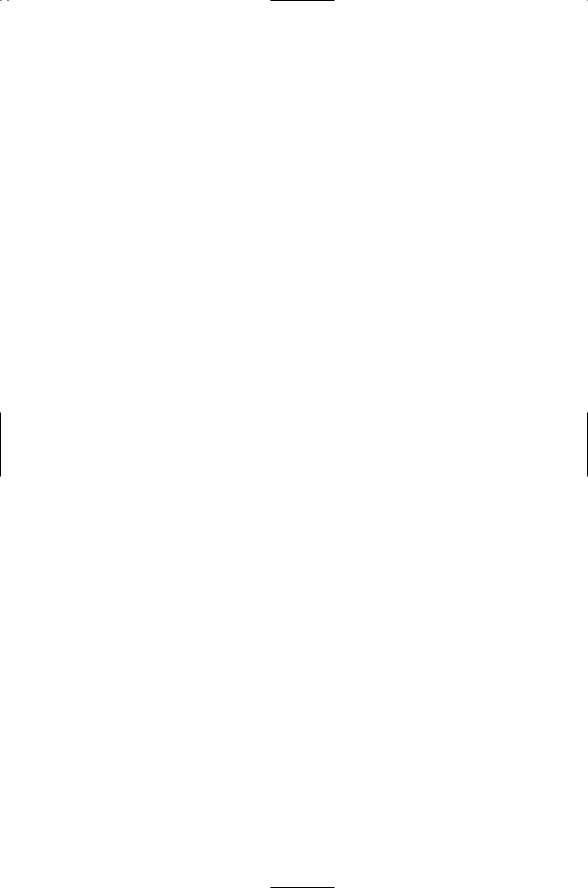
11.11 Roles of Focal Adhesion Kinase Family of NRTKs 261
brane receptors to paxillin; prominent among these is the focal adhesion kinase (FAK).
11.11Roles of Focal Adhesion Kinase Family of NRTKs
The FAK family of NRTKs promotes the assembly of signaling complexes at focal adhesions, and regulates motility and growth factor signaling. Focal adhesions are points of contact and adhesion between the cell and its supporting membranes. Focal adhesions are control points where growth and adhesion signals are integrated together and coordinated across multiple points of ECM-to-cell surface contact to govern the overall growth and movement of the cell. These control points not only regulate the assembly and disassembly of the focal adhesions but also convey signals that control cellular growth, proliferation, differentiation, and survival.
Extracellular matrix proteins, transmembrane proteins, and the actin cytoskeleton proteins participate in the adhesive contacts. Integrins and growth factor receptor co-localize at focal adhesions. The integrins bind to ECM proteins such as laminin and the growth factor receptors bind to growth factor ligands. In response to ligand binding by these receptors, a number of nonreceptor tyrosine kinases and adaptor/scaffold proteins are recruited to the plasma membrane. Among the nonreceptor tyrosine kinases recruited are Src, its negative regulator Csk, and focal adhesion kinase. These kinases along with paxillin, a key adapter, link the integrin and growth factor receptor-signaling to the actin cytoskeleton.
Paxillin is a fairly small protein; as mentioned previously it is only 68 kDa in mass, but it contains a large number of binding sites. Its structure is shown in Figure 11.7a. It possesses two tyrosine phosphorylation sites that are targeted by the nonreceptor tyrosine kinases such as Src, Csk and FAK, and bound by SH2 domain-bearing proteins subsequent to phosphorylation. A proline-rich region serving as an attachment site for SH3 domains is located in the same vicinity. These N-terminal sites provide a linkage to upstream integrins and growth factor receptors, and also downstream to proteins associated with the actin cytoskeleton through the five LD repeats. The C- terminal LIM domains anchor the paxillin protein at the plasma membrane.
The domain composition of FAK is presented in Figure 11.7b. It does not have any SH2 or SH3 domains but instead provides phosphorylation and anchoring sites for proteins with these domains. In place of the SH2 and SH3 domains FAK has two large domains of about 400 amino acids each, one on either side of the catalytic domain. FAK possesses six tyrosine phosphorylation sites. Two of these, Tyr397 and 407, lie just N-terminal to the kinase domain; two other, Tyr576 and 577, lie inside the kinase domain, and the last two, Tyr861 and 925, lie in the COOH terminal region N-terminal to the FAT. Autophosphorylation at Tyr397 exposes an SH2 docking site for Src.
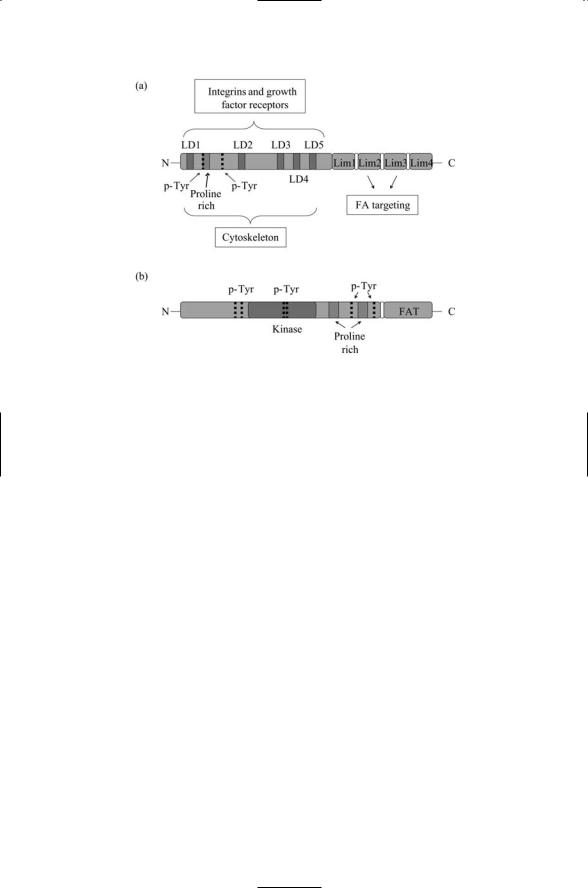
262 11. Signaling in the Endocrine System
FIGURE 11.7. Focal adhesion proteins paxillin and FAK: (a) Paxillin—The four Lin-11, Isl-1, Mec-3 (Lim) protein-protein interaction domains in the C-terminus mediate targeting to focal adhesions (FAs). The leucine-rich sequences, or LD repeats, sequences of the form LDXLLXXL, where X is any amino acid residue, found in the N-terminus domain bind FAK and proteins such as vincullin associated with the cytoskeleton. The N-terminal domain contains a number of motifs (tyrosine phosphorylation sites and proline-rich regions) that bind to Sh2and SH3bearing proteins. (b) Focal adhesion kinase (FAK)—This protein has a focal adhesion targeting (FAT) domain in its C-terminus, and several tyrosine phosphorylation sites and proline-rich regions in its N-terminal domain.
Phosphorylation by Src at Tyr407, 576, and 577 maximally activates the kinase domain, and phosphorylation at the sixth site,Tyr925, provides a Grb2 docking site. The domain structure of Grb2 was presented in Figure 11.6b. It is an adapte protein that links FAK to the MAP kinase pathway. There are also two proline-rich regions in the COOH terminal domain that provide docking sites for adapte proteins bearing SH3 domains. Thus, like paxillin, FAK serves as integrator of adhesive and growth signals. The COOHterminal region of FAK contains a focal adhesion targeting (FAT) sequence of about 160 amino acids that provides binding sites for paxillin and talin.
11.12GTPases Are Essential Regulators of Cellular Functions
Since 1982, an ever-increasing number of GTPases have been found in eukaryotes. Most of these belong to the Ras superfamily. The Ras GTPases are small, 20–40 kDa monomeric proteins that bind guanine nucleotides,
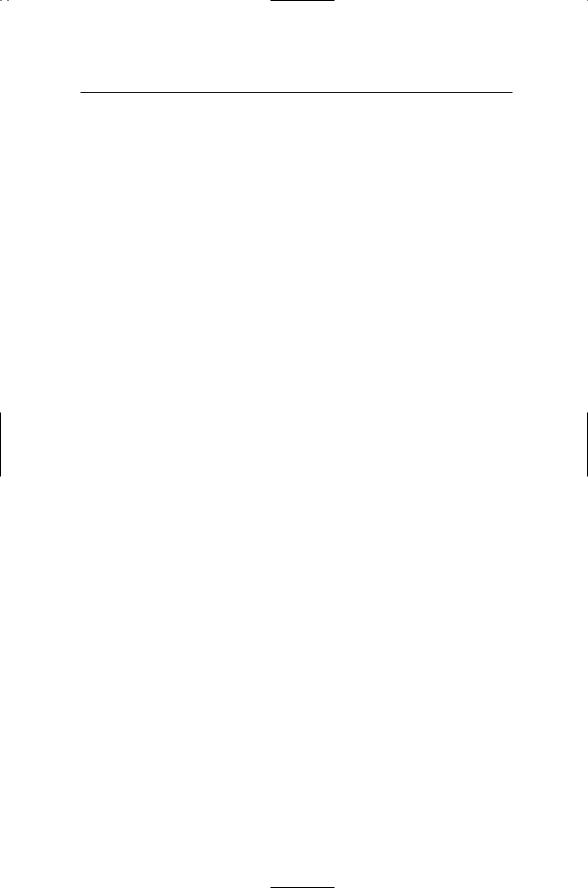
11.13 Signaling by Ras GTPases from Plasma Membrane and Golgi |
263 |
TABLE 11.3. The Ras superfamily of GTPases.
Family |
Function |
Ras |
Operates in the pathway that relays growth, proliferation, and differentiation |
|
signals to the nucleus |
Rho |
Relays coordinating signals to the actin cytoskeleton |
Ran |
Shuttles mRNAs and proteins in and out of the nucleus |
Rab |
Regulates the targeting and docking of cargo vesicles to membranes |
Arf |
Regulates the formation of cargo vesicles |
|
|
either GDP or GTP. More than 100 Ras superfamily GTPases have been identified to date in eukaryotes. Each of these can be placed into one of five families—Ras, Rho, Ran, Rab, or Arf (Table 11.3). There are several other groups of proteins that operate as GTPases. Chief among these are the Ga subunits of the heterotrimeric G proteins that associate with G protein-coupled receptors and the EF-Tu elongation factors that help regulate protein synthesis.
Members of the Ras superfamily of GTPases carry out a variety of essential regulatory tasks. They regulate transcription, migration and focal adhesion, transport through the nuclear pore complex, assembly of the nuclear envelope and mitotic spindle, and vesicle budding and trafficking. Ras family members act as upstream switches in the pathway that conveys growth and differentiation signals to the nucleus. Rho family members Rho, Rac and Cdc42 coordinate growth and adhesion signaling. They regulate the reorganization of the actin cytoskeleton, and coordinate its structure with gene expression in response to extracellular signals. Ran GTPases regulate the inport and export of molecules from the cytoplasm to the nucleus. They regulate the assembly of the nuclear envelope and also that of the mitotic spindle. Rab proteins with over 30 family members are the largest family of the Ras superfamily of GTPases. They are regulators of vesicle trafficking, while Arf GTPases control vesicle budding.
11.13Signaling by Ras GTPases from Plasma Membrane and Golgi
Ras is a major regulator of cell growth. Ras GTPases convey growth, proliferation, and differentiation signals to the nucleus from the plasma membrane and from the Golgi apparatus. Ras is implicated in a large number of human cancers, and in this context it will be examined further in Chapter 15. As shown in Figure 11.3 Ras operates as a master switch in the pathway connecting upstream receptors for growth factors with downstream MAP kinases that relay these signals to the transcription machinery in the nucleus. Once Ras becomes GTP-bound it activates the serine/threonine kinase Raf, which belongs to the Raf/MEK/ERK MAP kinase module.
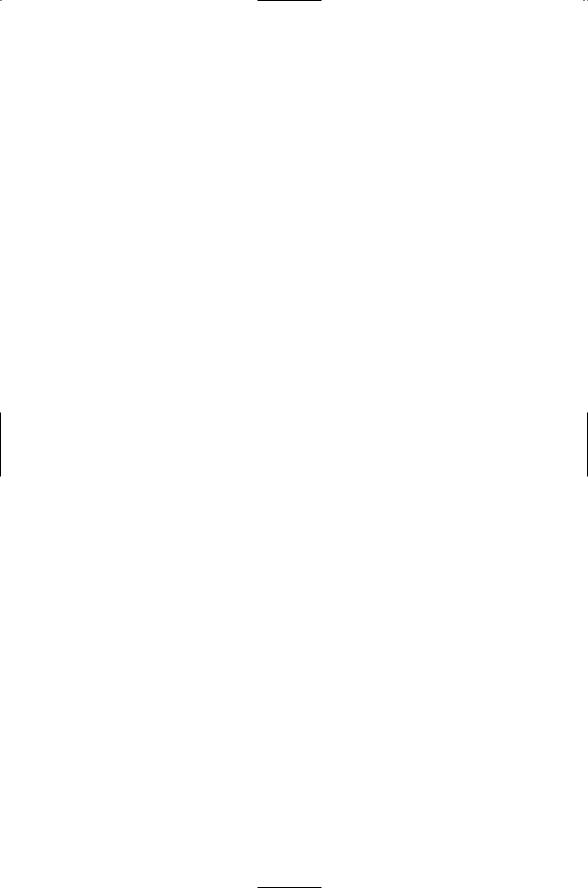
264 11. Signaling in the Endocrine System
Signaling through this module activates downstream transcription factors that stimulate growth and differentiation.
These downstream steps are preceded by upstream activation of receptor tyrosine kinases through ligand binding that creates binding sites for the Grb2 adapter protein. This adapter enables the RasGEF Son-of-sevenless (Sos) to bind and then accelerate the formation of GTP-bound Ras proteins. In the absence of GTP-bound Ras, the Raf kinase resides in the cytosol. GTP-bound Ras brings Raf to the plasma membrane. The Raf N- terminal domain binds Ras, thereby enabling the Raf C-terminal domain to bind and phosphorylate MEK.
Ras signaling is not restricted entirely to the plasma membrane. It can be activated at the Golgi through a calcium-dependent pathway involving a second pair of RasGEFs and RasGAPs. In place of the plasma membrane sequence of steps where the adapter Grb2 links receptor kinases to the RasGEF Sos, there is a pathway leading through Src. This nonreceptor tyrosine kinase activates phospholipase Cg (PLCg), which acts on PIP2 to produce DAG and IP3. The latter stimulates the release of Ca2+ from the intracellular stores. In response to release of DAG and Ca2+, the RasGEF RasGRP1 translocates to the Golgi where it activates Ras, while at the same time the RasGAP calcium-promoted Ras inactivator (CAPRI) relocates to the plasma membrane where it deactivates any plasma membraneassociated Ras.
11.14GTPases Cycle Between GTPand GDP-Bound States
All GTPases function in a similar manner, passing through an assisted cycle of GDP/GTP-binding and release. Recall from Chapter 6 and Figure 6.4 that GTPases bind either GTP or GDP. They are converted from GDPbound forms to GTP-bound forms by the catalytic actions of guanine nucleotide exchange factors (GEFs). GTPase-activating proteins (GAPs) catalyze their subsequent hydrolysis. In more detail, the GEFs “kick out” the GDP molecule. In most situations GTP is far more abundant than GDP and it readily binds the vacated pocket. In catalyzing the hydrolysis of the GTP, a molecule of a phosphoryl group is removed leaving a GDP molecule bound to the GTPase.
GTPases such as Ras function as molecular switches. These switches are turned “on” when GTP is bound and turned “off” when GDP is bound. In the “on” state the GTPases are continually active and become inactive only when turned off by a GAP. Ras is inactive when GDP-bound and it remains so until upstream signals conveyed by RasGEFs trigger the dissociation of GDP from Ras. Since the cellular concentration of GTP is far greater than that of GDP, the binding of GTP to Ras follows almost immediately. At this point Ras is activated and can bind to a downstream target, or effector,
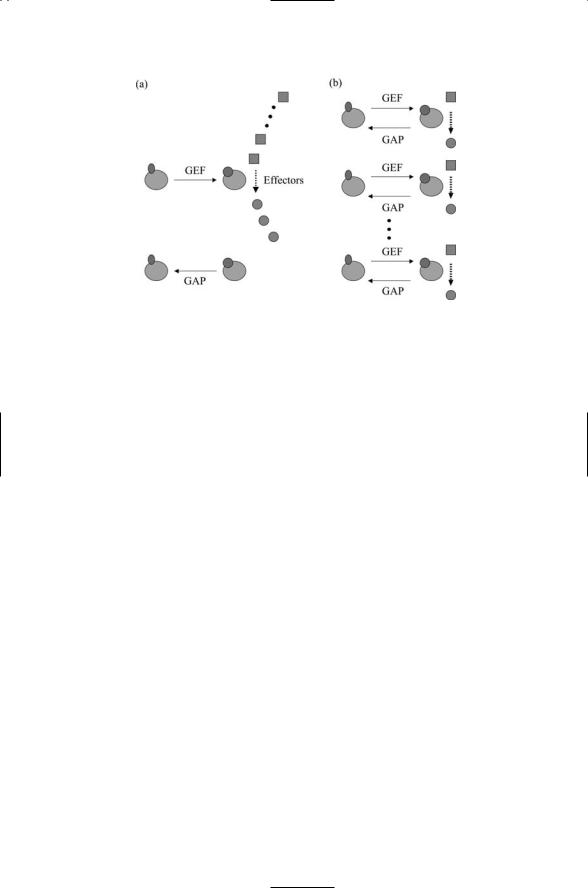
11.14 GTPases Cycle Between GTPand GDP-Bound States 265
FIGURE 11.8. GTPase cycles: (a) Switch cycle in which GTP-binding turns on the GTPase, which can then interact with multiple effector molecules. The GTPase will stay on until turned off by hydrolysis. (b) Assembly-controller cycle in which GTPbinding initiates interactions with an effector molecule. If the assembly is not correct, the assembly step is aborted prior to hydrolysis, a process that is necessary for disengagement from the substrate.
molecule. It remains active (on) until it undergoes hydrolysis. During the time it remains on it can activate many Raf proteins, as illustrated in Figure 11.8a.
The GEF for plasma membrane associated Ras is Sos (Son of sevenless). It works in the following way to speed up the dissociation of GDP from Ras: The Ras molecule, consisting of 188 amino acids residues, contains two flexible regions on its surface. These surface regions can alternate between several conformational substates, and they are referred to as Switch 1 and
Switch 2. In its inactive state the Ras molecule binds GDP tightly with a dissociation rate of 10-5/s. Sos-binding produces a tertiary Sos-Ras GDP complex that stabilizes Ras in a conformation in which Switch 1 has swung out to open the binding pocket. A portion of Sos stabilizes Switch 2 in an alternative conformation, and this change plus an altered electrostatic environment further weakens the binding of the phosphate group of GDP and its associated magnesium ion to Ras. The changes in the switch regions increase the dissociation rate by several orders of magnitude and allow GDP to exit the binding pocket in less than a second.
The Ras GAPs work in the following manner. Arginine and lysine residues have long side chains and are positively charged under physiological conditions. When bound to the Ras-GTP complex the Ras GAPs extend an “arginine finger” into the active site that neutralizes negative charges in its vicinity. A network of hydrogen bonds form, stabilizing the transition state and promoting the cleavage of the phosphodiester bond-linking