
Engineering and Manufacturing for Biotechnology - Marcel Hofman & Philippe Thonart
.pdf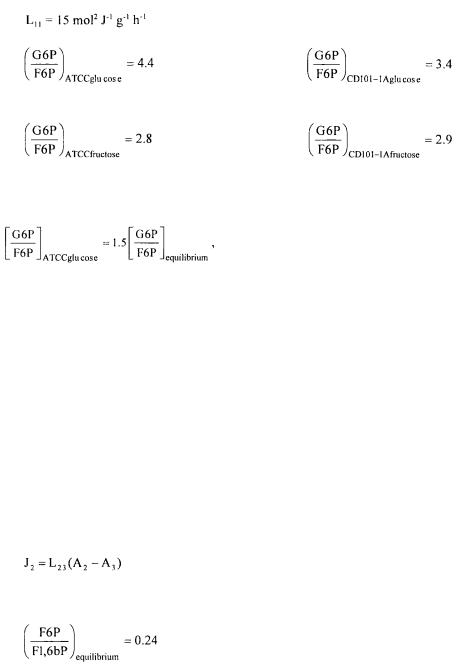
Metabolic flux modelling of a genetically modified strain of saccharomyces cerevisiae
The previous results show that the G6P/F6P ratio is slightly modified for the different culture conditions although the reactions rates are very different. Even if the
concentrations |
ratios |
are |
calculated |
from |
an |
arbitrary |
value |
the main result is that such an approach leads to
consider that a small change in intracellular concentrations may considerably modify the rates. Conversely, it may be concluded that the intracellular concentrations are finely tuned and are characteristic of a given physiological state.
The two other reactions catalysed by Phosphofructokinase and F1,6 biphosphatase do not proceed in the vicinity of thermodynamic equilibrium, knowing that F6P and
F1,6bP concentrations are of the same order of magnitude which cannot compensate for the offset values of and
(22.4 and
respectively). Moreover, this two enzymes system results in a futile cycle or substrate cycle (Fell, 1990) which globally hydrolyses ATP (reaction 4).
As previously indicated, the metabolic flux calculation (Table 2) leads to compute an overall resultant flux and a global maintenance flux which accounts for all futile cycles involved in the metabolism and other nondescript processes in the metabolic network such as membrane transport.
Starting from the hypothesis that for a purely futile cycle behaviour of this two enzymes system, the affinities of the two reactions might be equal, we calculated that the overall flux on glucose,
on fructose) could be correlated as previously in a linear expression of the difference of the affinities such as :
From the above, the purely futile cycle behaviour corresponds to so that :
153
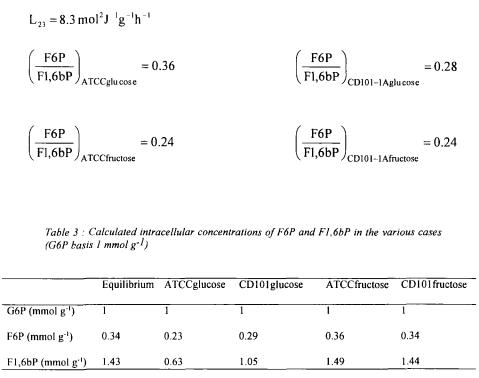
Urrieta-Saltijeral J.M., Dussap C.G., Pons A., Creuly C. and Gros J.B
Considering as previously that for the reference situation (ATCC strain cultivated on glucose) that the concentration ratio F6P/Fl,6bP is 1.5 times the equilibrium ratio, one obtains :
Starting from a reference value of 1 mmol for G6P intracellular concentration, the intracellular concentration of F6P and F1,6bP are calculated in table 3.
The results show that the Fl,6bP concentrations are always greater on fructose than on glucose and that the intracellular concentrations vary in narrow ranges for the different situations investigated.
4. Conclusion and perspectives
The study of a strain lacking the glucose-6P dehydrogenase enzyme was interesting to a thorough understanding of energetic metabolism of the yeast Saccharomyces cerevisiae.
Such a genetic mutation is not lethal which suggests that one or more points are disposable to produce NADPH,H+ species, generally obtained at the level of the pentose phosphate pathway. The statistical analysis of a complete set of experimental results for anaerobic growth of the reference (ATCC 77554) and the mutant (CD101-1A) strains enable to draw a map of specific intracellular rates, including the estimation of the reliability of the predictions. This analysis conducts to the selection of 3 enzymes as possible points of NADPH,H+ production. The selected network allows a satisfactory qualitative interpretation of the possible development of the mutant strain which seems to undergo NADPH,H+ production through acetate synthesis with the operation of the
154
Metabolic flux modelling of a genetically modified strain of saccharomyces cerevisiae
NADP dependent acetaldehyde dehydrogenase. The flux computation confirms that, in yeast, the pentose phosphate pathway is mainly devoted to anabolic functions. These results allow to conclude that pentose phosphate pathway as well as NADP dependent acetaldehyde dehydrogenase stand as limiting rates providing control among all other reactions in the network.
The analysis of the upper part of glycolysis in terms of linear thermodynamics of irreversible processes has enabled to calculate the intracellular concentrations on the basis of an assumed reference situation of exponential growth of the ATCC strain on glucose. Obviously the computed values need to be compared to experimental measurements of intracellular metabolites concentrations. However, the obtained calculated results indicate that slight variations of intracellular concentrations correspond to completely different distributions of intracellular flux. Such a result is consistent with the general assumption that the intracellular concentrations of key metabolites are highly regulated variables. This leads to conclude that such a phenomenological approach, via thermodynamics of irreversible processes, is an interesting tool of the investigation of cellular metabolism.
References
Andreasen A.A. and Stier T.J.B. (1953). Anaerobic nutrition of Saccharomyces cerevisiae I. Ergosterol requirement for growth in a defined medium J. Cell. Comp. Physiology, 41, 23-26.
Andreasen A.A. and Stier T.J.B. (1954). Anaerobic nutrition of Saccharomyces cerevisiae II. Unsaturated fatty acid requirement for growth in a defined medium. J. Cell. Comp. Physiology, 43, 271-281.
Çalik P., Çalik G., Takaç S., Özdamar T.H. (1999). Metabolic flux analysis for serine alkaline protease fermentation by Bacillus licheniformis in a defined medium : Effects of the oxygen transfer rate.
Biotech. Bioeng., 64, 151-167.
Dussap C.G. (1988). Etude thermodynamique et cinétique de la production de polysaccharides microbiens par fermentation en limitation par le transfert d'oxygène. Thèse de Docteur ès Sciences Physiques de 1' Université Blaise Pascal - Clermont-Ferrand, France.
Dussap C.G., Pons A., Péquignot C., Gros J.B. (1997). Application d’une méthode de réconciliation de données à des cultures menées en fermenteur en discontinu. Récents Progrés en Génie des Procédés. Lavoisier Technique et Documentation, vol. 11(57), 7-12.
Fell D.A. (1990). Substrate cycles : theoretical aspects of their role in metabolism. Comments Theoretical
Biology, 1, 341-357.
Goel A., Lee J., Domach M.M., Ataai M.M. (1999). Metabolic fluxes, pools, and enzyme measurements suggest a tighter coupling of energetics and biosynthetic reactions associated with reduced pyruvate kinase flux. Biotech. Bioeng., 64, 129-134.
Heinrich R. and Schuster S. (1996). The regulation of cellular systems. Chapman & Hall, New York. Himmelblau D.M., (1968). Process analysis by statistical methods. John Wiley & Sons, New York. Kristiansen B. (1994). Integrated design of a fermentation plant, VCH - Weinheim.
Lagunas R. and Gancedo C. (1973). Reduced pyridine-nucleotides balance in glucose-growing
Saccharomyces cerevisiae. Eur. J. Biochem., 37, 90-94.
Lee S.Y. and Papoutsakis E.T. (1999). Metabolic Engineering. Marcel Dekker Inc, New York.
Nielsen J. and Villadsen J. (1994). Bioreaction Engineering Principles. Plenum Press, New York.
Ould-Moulaye C.B., Dussap C.G., Gros J.B. (1999). Estimation of Gibbs energy changes of central metabolism reactions. Biotechnol. Techniques, 13, 187193.
Oura E. (1983). Formation of biomass from carbohydrates. In Biotechnology, H.J. Rehm and G. Reed (eds.), Verlag Chemie, vol 3, pp. 3-16.
Pons A., Dussap C.G., Péquignot C., Gros J.B. (1996). Metabolic flux distribution in Corynebacterium melassecola ATCC 17965 for various carbon sources. Biotech. Bioeng., 51, 177-189.
155
Urrieta-Saltijeral J.M., Dussap C.G., Pons A., Creuly C. and Gros J.B
Pramanik J. and Keasling J. D. (1997). Stoichiometric model of Escherichia coli metabolism : incorporation of growth-rate dependent biomass composition and mechanistic energy requirements. Biotech. Bioeng., 56, 398-421.
Thomas D, Cherest H., Surdin-Kerjan Y. (1991). Identification of the structural gene for glucose-6- phosphate dehydrogenase in yeast. Inactivation leads to a nutritional requirement for organic sulphur.
The EMBO Journal, 10, 547-553.
Vallino J.J. and Stephanopoulos G. (1990). Flux distribution in cellular bioreaction networks : application to lysine fermentations. in Frontiers in Bioprocessing, S.K. Sikdar (ed.), CRC Press, pp. 205-219.
Vallino, J.J. and Stephanopoulos G. (1993). Metabolic flux distributions in Corynebacterium glutamicum during growth and lysine overproduction. Biotech. Bioeng., 41, 633-646.
Vanrolleghem P.A., de Jong-Gubbels P., van Gulik M.W., Pronk J.T., van Dijken P.J., Heijnen S. (1996). Validation of a metabolic network for Saccharomyces cerevisiae using mixed substrate studies.
Biotechnol. Prog., 12, 434-448.
Veverka V.V. and Madron F. (1997). Material and energy balancing in the process industries. Elsevier, Amsterdam.
156
METABOLIC INVESTIGATION OF AN ANAEROBIC CELLULOLYTIC BACTERIUM : FIBROBACTER SUCCINOGENES
C. CREULY, A. PONS, AND C.G. DUSSAP
Laboratoire de Génie Chimique et Biochimique. Université Blaise Pascal. Clermont-ferrand II. 24 avenue des Landais. F63177 Aubière cedex.
Abstract
Fibrobacter succinogenes, an anaerobic bacteria is cultivated in a batch reactor. A detailed stoichiometric model of metabolism is developed. It includes all reactions of
catabolic pathways that have been proved to exist and anabolic pathways. The measured conversion yields are correlated in terms of metabolic flux distribution using a mathematical technique of yields calculation associated with a methodology of data reconciliation. This approach validates the metabolic network built on Fibrobacter succinogenes.
1. Introduction
Microbial cellulases and hemicellulases are widely used in different industrial activities, such as in textile, detergent, brewery or wood-processing, and also in the treatment of domestic wastes and in biological treatment of fibrous feeds in the non-ruminant livestock industry. However, these enzymes are not very efficient for the degradation of highly lignified plant cell walls because cellulose and hemicelluloses are cross-linked to lignin which is very difficult to degrade, and protects cellulose and hemicelluloses against enzymatic hydrolysis (Selinger et al., 1996).
Ruminant animals possesses rumen bacteria which developed a symbiotic relationship to digest lignocellulosic substrates (Hungate, 1950). Fibrobacter succinogenes is a major fibrolytic bacterium found in the rumens of cattle and sheep.
With the aim of developing a biotechnological process for the degradation of lignocellulosic residues, we propose to take advantage of the high potentiality of Fibrobacter succinogenes. Its enzymatic equipment explains these specific performances ; it includes a very efficient cellulolytic system, ferulic acid and acetylxylane esterases, arabinofuranosidases, xylanases and glucuronidases (Chesson and Forsberg, 1997). This strictly anaerobic bacterium uses cellulose, glucose and
157
M. Hofman and P. Thonart (eds.), Engineering and Manufacturing for Biotechnology, 157–167.
© 2001 Kluwer Academic Publishers. Printed in the Netherlands.

Creuly C. Pons A. and J.M., Dussap
cellobiose as carbon and energy sources, and produces succinate, acetate and few formate (Stewart and Flint, 1989).
The development of a high-performance bioreactor is based on a general concept of metabolic engineering : the idea is to estimate metabolic fluxes in F. succinogenes to direct bacterial metabolism towards the production of biomass and enzymes of interest.
The aim of this paper is to show how, using the data concerning the biochemistry of F. succinogenes and the measured conversion yields, the special metabolic pathways specific of those anaerobic bacteria could be validated. The supposed metabolic network involves 96 stoichiometric reactions. Validation of the model is obtained by comparison between the theoretical yields of carbon elements calculated by a data reconciliation technique and the experimental yields measured during anaerobic cultures of F. succinogenes in a bench scale bioreactor.
2. Material and method
2.1. STRAIN AND CULTIVATION
Fibrobacter succinogenes S85 (ATCC 19169) was originally isolated from the bovine rumen (Bryant and Doetsch, 1954) and has been maintained as pure culture in laboratory ever since. It was grown anaerobically under in a medium containing (per litre):
Biotin, 0.005 mg para-aminobenzoic acid, 500 mg Cysteine, 4 g
Glucose and a volatile fatty acid mixture (Gaudet et al, 1992). 1.2 litre of the above medium was sterilised (120°C, 20 min) in the reactor (2 litres total volume).
2.2. EXPERIMENTAL DESIGN
After redox potential reduction at -350 mV and temperature equilibration at 37°C, the thermostated, stirred (set at 40 rpm) fermentor (1.5 1) was inoculated with 200 ml of an overnight culture. pH and redox were measured on line. Growth was monitored by the increase of optical density (600nm). Growth was correlated with the decrease of the pH since the initial value (6.9) until value 5.5. At this value, pH was readjusted at neutral value by addition. In the same order, a substrate feed was performed when glucose concentration became limiting (fed-batch technique)
2.3. METABOLITES ASSAYS
Cell mass was determined by dry weight at 100°C after a centrifugation step. Extracellular metabolites (glucose, succinate, acetate, volatile fatty acids) were quantified in cell free samples by high pressure-liquid chromatography (1100 series Hewlett Packard, 1047A refractometer analyser) fitted with two ionic exclusion columns (Phenomenex rezex organic acid - 300*7.8 mm) maintained at 80 °C and isocratic eluted with solvent 5 mM
158
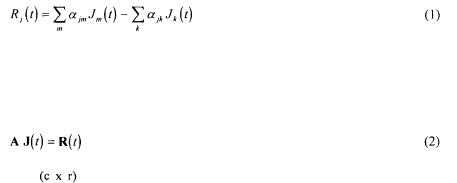
Metabolic investigation of an anaerobic cellulolytic bacterium
2.4. FLUX ESTIMATION AND STATISTICAL ANALYSIS
Metabolic fluxes were calculated from a mass balance technique. This method takes into account the stoichiometric reactions obtained by an analysis of the internal behaviour of the microorganism. The metabolic reactions network is depicted in terms of matrices and vectors algebra for representing the metabolites balances. The bioreaction network (Vallino and Stephanopoulos, 1990) is used to determine the rates of production and consumption of each metabolite in the network as a function of all the unknown fluxes
for the ith reaction). The accumulation rate of a metabolite in a metabolic network is given by the summation of all reactions producing that metabolite minus the reactions consuming it.
where are the stoichiometric coefficients,
is the flux through reaction m and the
is the accumulation rate of metabolite j.
The set of equations formed from such balances for each metabolite in the network is represented in matrix notation by :
A is the |
matrix of stoichiometric coefficients (c metabolites and r metabolic |
reactions) of all the reactions involved in the metabolism. Each column in the matrix represents a metabolic reaction.
The c metabolic constituents are divided into two categories :
•the n exchangeable compounds, which are exchanged with the growth medium, from a macroscopic point of view, these compounds are the products and the substrates involved during the cellular growth phase ; their accumulation rates are linked to growth yields ;
•the m non-exchangeable compounds, which are only involved inside the cell ; for
these the accumulation rate is assumed to be equal to zero.
This is a pseudo-steady state hypothesis that expresses that the rate of accumulation of intracellular compounds is negligible compared to the rates of production / consumption of exchangeable compounds (Vallino and Stephanopoulos, 1990). Assuming that during a sufficiently long period of growth the yields remain constant (some of them being measured), and that non-exchangeable compounds are not accumulated inside the cell, the previous Equation (2) is split in two parts: the first part corresponds to the known accumulation rates (non-exchangeable compounds and measured yields), the second part being the remaining rows corresponding to the unknown yields (Pons et al, 1996).
The number of supplied information must at least be greater than the number of columns i.e. of unknown fluxes J. In case of equality, provided matrix A is of full rank, fluxes are calculated by inversion of the matrix.
In case of redundant information (more independent rows than columns) a data reconciliation technique is worked using the conservation laws of non-exchangeable
159
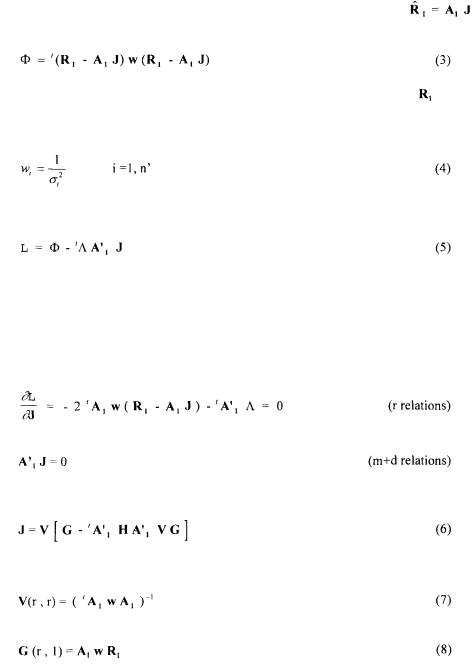
Creuly C. Pons A. and J.M., Dussap
compounds as constraints. To solve this problem of optimisation under constraints, we use a Lagrange method in minimising a quadratic criterion calculated between the n’
measured values of the yields contained in and the computed values (Dussap et al., 1997).
where w is the diagonal weighted matrix of the n’ experimental values in |
which |
account for the accuracy of the determination. In the case under study, variances of the measured yields are known and elements of w are given by:
The Lagrange function to be considered is given by :
where is a matrix
and
is a vector
contains all the rows corresponding to the m non-exchangeable compounds plus the d relationships between some of the fluxes necessary to eliminate to metabolic cycles included in the network (Dussap et al., 1997). This makes (m+d) rows for
r flux of J and values of A vector are calculated by solving the system:
The solution is given by:
where V, H and G matrices are defined as:
160
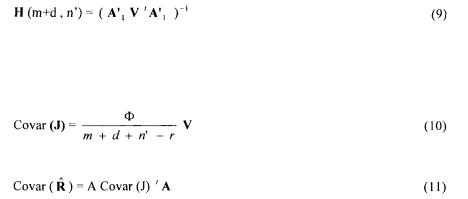
Metabolic investigation of an anaerobic cellulolytic bacterium
The re-calculated conversion rates values are given by where, the (m+d) drawn values are found by construction. The standard deviations of the calculated flux and rates are estimated by diagonal elements of matrices of covariance:
This set of Equations (10,11) leads to compute the best estimates of all the yields and all of the fluxes. Statistical analysis also provides the standard deviations of the estimation.
3.Results and discussion
3.1.METABOLIC NETWORK
The purpose of this work is to present a novel approach on this bacterium by developing a detailed stoichiometric model of anaerobic metabolism that includes a more complete database of known reactions involved in the central catabolism of glucose and previously established anabolic reactions based on the general knowledge of bacterial metabolism (Gottschalk, 1986) (Figure 1).
The aim is to check if the present reported biochemical pathways could be consistently integrated in the overall growth metabolism without involving thermodynamically impossible reactions. The major features of catabolic pathways, which have been described, are as follows:
•Glucose is transported across the cytoplasmic membrane through independent constitutive transporters that are sodium dependent. In the cytoplasm, glucose is phosphorylated by a GTP-dependent glucokinase (Glass and Sherwood, 1994). Cultures that were provided with glucose produced cellobiose, and cellobiose gave rise to cellotriose. Gaudet et al (1992) showed that Fibrobacter succinogenes continuously synthesised and degraded glycogen during all phases of growth, but its dependency on glycogen catabolism was not defined.
•As it possesses fructose 1,6-biphosphate aldolase and glyceraldehyde 3-phosphate dehydrogenase, Fibrobacter succinogenes is assumed to ferment hexoses by the
Embden-Meyerhof-Parnas pathway (Joyner and Baldwin, 1966; Miller, 1978) until pyruvate. Phosphoenolpyruvate is carboxylated to oxaloacetate by a GDPspecific PEP carboxykinase.
•Oxaloacetate is converted to malate by a pyridine nucleotide-dependent malate dehydrogenase (Table 1; J20).
161
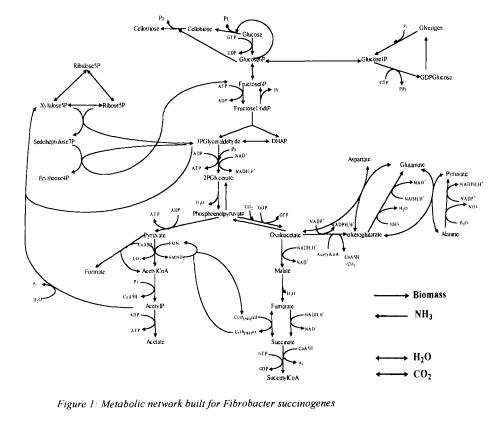
Creuly C. Pons A. and J.M., Dussap
•Fumarase activity (Table 1; J14) was not demonstrated but it is probably present to produce fumarate from malate. Fumarate is reduced by a flavin-dependent, membrane bound fumarate reductase to produce the major fermentation product, succinate. The reduction of fumarate with reduced flavins is likely to involve cytochrome b (Miller, 1978). Flavin nucleotides mediate electron transport between pyruvate and fumarate (Table 1; three coupled reactions J9, J79, J77).
In addition, Fibrobacter succinogenes possesses the essential enzymes of the nonoxidative branch of the pentose phosphate pathway (Matte et al, 1992), though known to be unable to metabolise pentoses. Enzymatic researches on the oxidative branch give negative results. This specificity imposes to set glutamate dehydrogenase (Table 1; J22) on NAD/NADH dependence and isocitrate dehydrogenase NADP / NADPH specific (Table 1; J82). Matheron et al (1999) have isolated a specific enzyme that produce alanine from pyruvate and (Table 1; J80).
162