
Kluwer - Handbook of Biomedical Image Analysis Vol
.2.pdf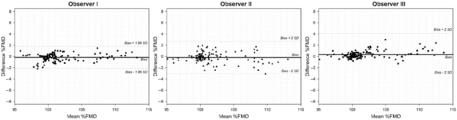
242 |
Frangi, Laclaustra, and Yang |
5.4.2.1 Manual Measurements
Manual measurements of arterial diameter were performed in 117 frames corresponding to four sequences of different image quality. Three experts assessed each frame twice in independent sessions. In each sequence several frames were measured: 1 out of 10 in phase B1 (frame number 1, 11 . . . 61) and 1 out of 50 during the rest of the test (frame number 101, 151 . . . ). Depending on the duration of the sequence the total number of measured frames was between 28 and 30 per sequence.
Each diameter measurement was obtained by manually fitting a spline to the inner contour of each arterial wall. The diameter was defined as the average distance between both spline curves (see Appendix 5.8). The vasodilation measurements were obtained by dividing the manually obtained diameters by the average diameter over phase B1; the dilation was finally expressed as a percentage, getting %FMD values as defined before.
Gold-standard measurements were derived from these 117 frames. The grand-average of the six diameter measurements done by the three observers is considered the gold-standard arterial diameter estimate for each frame. The gold-standard dilation measurements are obtained by dividing these estimated diameters by the grand-average diameter over phase B1 for each sequence, to get %FMD values. Accuracy, reproducibility, and intraand interobserver variability of manual measurements were analyzed:
(i)Accuracy. Figure 5.7 shows the Bland–Altman plots comparing the intersession average measurement for each observer and the gold-standard measurements. The biases and standard deviation of the differences for
Figure 5.7: Bland–Altman plots comparing the intersession measurement average versus the gold-standard measurements. The horizontal and vertical axes indicate the average %FMD and the difference %FMD, respectively.

Computerized Analysis and Vasodilation Parameterization of FMD |
243 |
||||
|
Table 5.3: Accuracy of manual measurements |
|
|||
|
|
|
|
|
|
|
|
Obs I |
Obs II |
Obs III |
|
|
|
|
|
|
|
|
Bias (%FMD) |
−0.16 |
−0.18 |
0.34 |
|
|
SDa (±%FMD) |
0.68 |
0.94 |
0.68 |
|
|
SDw (±%FMD) |
0.74 |
1.14 |
1.41 |
|
|
SDc (±%FMD) |
0.86 |
1.24 |
1.20 |
|
Bias and standard deviation of the differences (SDc ), corrected for repeated measurements, between manual and gold-standard %FMD measurements. SDa and SDw stand for the SD of the differences of the intersession average and the within-observer variability.
the three observers are given in Table 5.3. Standard deviations are corrected to take into account repeated measurements according to the method proposed by Bland and Altman [26].
(ii)Reproducibility. The CV of each group of six measurements is calculated for each one of the 117 manually measured frames. This CV is averaged for all the frames of each one of the four sequences, being considered the CV of the manual measurement for each sequence. These four values are averaged finally, obtaining an overall reproducibility value for manual measurements in our study. The results are shown in Table 5.4.
(iii)Interand intraobserver variability. Figure 5.8 shows Bland–Altman plots comparing both sessions of each observer. In order to estimate the overall interand intraobserver variability of manual measurements (with correction for repeated measurements) we carried out the procedure proposed by Bland and Altman in [27]. To this end, a two-way Analysis of Variance (ANOVA) with repeated measurements was performed
Table 5.4: Reproducibility of manual and automated measurements
|
|
|
|
CV (m ± SD) |
|
|
|
|
Seq A |
Seq B |
Seq C |
Seq D |
Overall |
|
|
|
|
|
|
|
Manual (%) |
0.95 ± 0.5 |
1.20 ± 0.4 |
0.71 ± 0.6 |
1.35 ± 0.6 |
1.04 ± 0.6 |
|
Computerized (%) |
0.23 ± 0.1 |
0.26 ± 0.1 |
0.32 ± 0.3 |
0.84 ± 0.4 |
0.40 ± 0.3 |
Mean and SD of CV (%) measured with respect to %FMD value.

244 |
Frangi, Laclaustra, and Yang |
Figure 5.8: Bland–Altman plots comparing the two manual sessions of dilation measurements of each observer. The horizontal and vertical axes indicate the average %FMD and the difference %FMD of the two sessions, respectively.
using Analyse-it v 1.68 (Analyse-it Software Ltd, Leeds, UK). The two-way ANOVA was controlled by observer and measurement frame as fixed factors and by the session number as random factor (Table 5.5). From this analysis, the interand intraobserver within-frame %FMD standard deviations were 1.20% and 1.13%, respectively.
5.4.2.2 Computerized Measurements
The scaling factor in the direction normal to the vessel axis that relates each frame to the reference frame constitutes the vasodilation parameter output by the automatic method. As a consequence, the measurements are normalized to the arterial diameter of the reference frame. This normalization is different from that of the gold-standard dilation measurements, which, as described before, were normalized for each sequence to the grand-average diameter over phase B1. To make the computerized measurements comparable to the gold standard,
Table 5.5: A two-way ANOVA of manual measurements of %FMD
Source of variation |
SSq |
DOF |
MSq |
F |
p |
|
|
|
|
|
|
Frame |
9329.1 |
116 |
80.423 |
62.82 |
<0.0001 |
Observer |
19.4 |
2 |
9.708 |
7.58 |
0.0006 |
Observer × Frame |
359.7 |
232 |
1.551 |
1.21 |
0.0529 |
Session |
449.4 |
351 |
1.280 |
|
|
Total |
10157.6 |
701 |
|
|
|
|
|
|
|
|
|
SSq: Sum of squares; DOF: degrees of freedom; MSq: mean squares; F: F of Snedecor;
p: Snedecor test significance.
Computerized Analysis and Vasodilation Parameterization of FMD |
|
245 |
||||||
|
Table 5.6: Comparison between different similarity metrics |
|
|
|
||||
|
|
|
|
|
|
|
|
|
|
|
NMI |
MI |
GCC |
JE |
CC |
SSD |
|
|
|
|
|
|
|
|
|
|
|
Bias (%FMD) |
+0.05 |
+0.11 |
+0.25 |
−1.00 |
+1.03 |
+1.68 |
|
|
SD (± %FMD) |
1.05 |
1.08 |
2.02 |
2.49 |
2.55 |
3.92 |
|
Bias and difference SD in the comparison between the gold standard measures and the automatic dilation obtained with different similarity measures. Values reported correspond to %FMD values. NMI: Normalized mutual information; MI: mutual information; GCC: gradient image cross correlation; JE: joint entropy; CC: cross correlation; SSD: sum of squared differences.
a new normalization of the former measurements is necessary. To this end, the values measured at each frame are divided by the average values over all measurements of phase B1, and are multiplied by a factor 100 to obtain %FMD values.
(i)Choosing a similarity measure. Several similarity measures traditionally used in image registration were compared to select the most appropriate one. Thus the four sequences where gold-standard measurements were available were processed using the six similarity measures introduced in Table 5.1. Finally gold-standard vasodilations were compared to the automated vasodilations computed using each registration measure. Table 5.6 indicates that NMI yields the most accurate estimates although the results are only marginally better than using MI. NMI is therefore the similarity measure selected.
(ii)Accuracy. Figure 5.9 shows a Bland–Altman plot comparing the automated versus the gold-standard measurements. The SD of the differences is 1.05%. The dilation curves obtained by the proposed method are superimposed to the gold-standard measurements in Fig. 5.10 where we also include the 95% confidence interval of the gold-standard measurements for comparison [26].
(iii)Robustness. The whole set of 195 sequences were processed with the proposed method (more than 280,000 frames). The overall result was ranked according to the ability to recover the clinically relevant information from the corresponding vasodilation curve. The results were classified as good, useful, and bad, depending on the amount and severity of the artifacts present in the curve. When, in the opinion of an expert
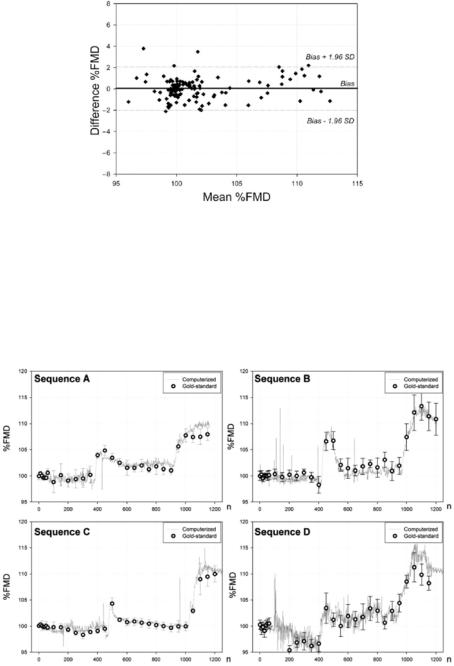
246 |
Frangi, Laclaustra, and Yang |
Figure 5.9: Bland–Altman plot comparing the automatic measurements (using normalized mutual information as similarity measure) versus the gold standard. The horizontal and vertical axes indicate the average %FMD and the difference %FMD of the automatic measurements and the gold-standard measurements, respectively.
Figure 5.10: FMD curves obtained by the proposed automated method (–) and by the gold-standard measurements (•). Error bars show the 95% confidence interval of the gold-standard measurements for comparison.
Computerized Analysis and Vasodilation Parameterization of FMD |
247 |
sonographer, there were no evident artifacts in the vasodilation curve, the result was scored as good (77.3% of sequences). Artifacts considered were, for instance, lack of convergence or unusual vasodilation evolution. A vasodilation curve was ranked as useful (5.2% of sequences) when artifacts appear only in the DI phase (Fig. 5.2), where no medical information is to be extracted, and therefore, it would still be possible to get clinical information from the other phases. When artifacts appeared in any of the other phases, from where clinical information should be derived, the result was ranked as bad (17.5% of sequences). The vasodilation curves were extracted in a fully automatic fashion with the preprocessing of the reference frame as the only manual intervention from the operator.
(iv)Reproducibility. The four sequences with gold-standard measurements were analyzed with the automatic method in six independent runs. Each time a different reference frame was randomly chosen from within phase B1 and it was manually preprocessed (horizontal repositioning of the vessel and removal of extra luminal structures). The CV was computed using as a basis the six dilation measurements for each frame of each sequence. Subsequently, the mean CV in each sequence was obtained by averaging the CV values of the frames where manual measurements were also carried out. These four values are presented in Table 5.4.
5.5Parameterizing the Vasodilation Response
In the last few years there has been a growing interest in understanding the link between endothelial function and several aspects of cardiovascular diseases (CVD). It is known that impaired endothelial function is associated with a number of disease states, including CVD and its major risk factors [28–31]. Also, endothelial dysfunction seems to precede by many years other more manifest symptoms and may itself be a potentially modifiable CVD risk factor. Therefore, it promises to have not only diagnostic value but also use as an instrument for patient monitoring during treatment.
Once that ongoing research establishes the role and value of FMD in clinical practice, and that computerized tools like the one presented in this chapter
248 |
Frangi, Laclaustra, and Yang |
become extensively validated and accepted in clinical protocols, it will be possible to move one step further and use the information contained in FMD curves to derive new clinical indexes of endothelial function. In this section we provide our initial experience in this direction and suggest a possible approach to parameterize FMD curves.
In most clinical papers, FMD is classically measured according to the expression
FMDc = |
max − basal |
× 100% |
(5.13) |
|
|||
|
basal |
|
where max and basal are the maximal and basal arterial diameters, respectively. One of the problems generally not discussed in the clinical literature is that manually measuring these diameters can be cumbersome without access to the whole dilation curve. Estimation of max by visual inspection of the ultrasound image sequence is time-consuming and subjective, given the small magnitude of the measured dilations. However, using a computerized technique like the one presented here can not only simplify this issue but also provide richer information about the dynamics of the dilation process.
There is still a problem on how to summarize the information present in FMD curves with only a few informative and intuitive parameters. To this end we propose the use of principal component analysis.
5.5.1 Robust EigenFMD and EigenD Modes
Principal component analysis (PCA) [32] is a classical statistical tool to analyze multidimensional datasets by determining the dominant axes according to a maximum variance subspace principle. As the underlying mathematical machinery can be shown to be equivalent to an eigenanalysis of the covariance matrix, PCA axes are usually also named eigenmodes. In cases where outliers can be present in the dataset, there exist robust extensions to PCA, which are less sensitive to wrong or imperfect data samples.
In our application, we performed a PCA-based statistical decomposition of FMD curves and explored the use of the projections on the corresponding eigenmodes (mode coefficients) as potential surrogate indexes of classical FMDc. To our purpose we will use a robust version of PCA known as ROBPCA [33] to avoid problems derived from potentially imprecise dilation curves in our dataset.
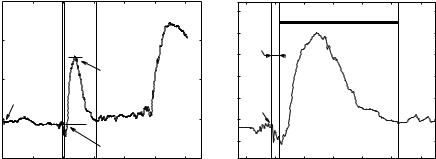
Computerized Analysis and Vasodilation Parameterization of FMD |
249 |
115 |
|
|
|
|
|
|
|
|
|
|
|
|
110 |
|
|
|
|
|
|
108 |
110 |
|
|
|
|
|
|
|
|
|
|
|
|
106 |
105 |
|
|
|
max |
|
104 |
|
|
|
|
|
FMD[%] |
|
FMD[%] |
r |
|
|
|
|
|
|
|
|
|
|
102 |
|
|
|
|
|
|
|
|
100 |
|
|
|
|
|
100 |
|
|
|
|
basal |
|
98 |
|
|
|
|
|
|
|
95 |
200 |
400 |
600 |
800 |
1000 |
1200 |
0 |
||||||
|
|
|
|
n[s] |
|
|
|
|
|
(a) |
|
|
|
|
Segment of Analysis |
|
|
||
|
d |
|
|
|
|
|
|
ncuff |
|
|
|
|
|
350 |
400 |
450 |
500 |
550 |
600 |
650 |
|
|
|
n [s] |
|
|
|
(b)
Figure 5.11: Definition of the segment of analysis for principal component analysis (PCA). (a) The original curve with indication of the instant of cuff release (first discontinuous vertical line), and start- (ncuff + 15 sec) and end-points (206 sec later) for PCA (second and third disconstinuous vertical lines); horizontal solid lines indicate the maximum flow-mediated dilation (FMD) and basal FMD levels used for the computation of classical FMDc. Horizontal solid lines indicate the maximum FMD ( max) and basal FMD levels ( basal), which are relative to a reference frame ( r). (b) A zoom into the segment that will undergo PCA. The segment of analysis is indicated with a thick solid line.
To be more specific, let CT (n) be an FMD curve (Fig. 5.11(a)). In order to analyze only FMD effects, a segment of this curve, C(n), was selected for PCA (Fig. 5.11(b)). This segment is defined to have a duration of D = 206 sec starting d = 15 sec after the release of the cuff pressure. In this way, all segments are synchronized with the FMD onset and have the same duration, which in our acquisition protocol is enough to reach the post-FMD basal level in all subjects without overlapping with the NMD test. We disregard the first 15 sec since that portion of the curve is mostly noisy due to motion artifacts. FMDc values were also measured by visually selecting the maximal and basal FMD levels. Since the whole curve is relative to the diameter of the reference frame, FMDc can be calculated directly from this plot. By considering the C(n) curve as a
D-dimensional vector, c, ROBPCA will be applied to the vector set {ci/Ci(no)}, of normalized relative values, where i = 1 . . . n is the subject index. In our experience, it is convenient to compute the EigenFMD modes with respect to a frame that represents the basal level, hence the division by Ci(no) (cf. next
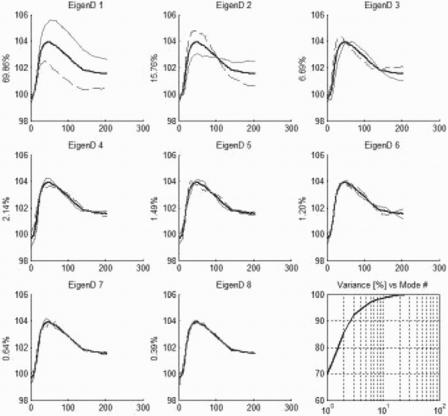
250 |
Frangi, Laclaustra, and Yang |
section). This renormalization corrects for small fluctuations in the baseline due to patient movements in the maneuver of cuff deflation, which themselves can introduce artifactual variation into the statistical analysis. This experiment contains n = 161 subjects, whose curves did not have any apparent artifacts in the FMD segment. Figure 5.12 shows the resulting eigenmodes of the relative FMD curves, which will be referred as EigenFMD modes in the sequel.
Figure 5.12: Representation of the variation explained by the first eight eigenFMD modes. The average flow-mediated dilation (FMD) curve, and the curves resulting of adding/subtracting the corresponding EigenFMD mode of variation to the mean, weighted by one standard deviation, are plotted in bold line and in thin solid/dashed lines, respectively. The y-axis label of each EigenFMD plot indicates the percentage of variance explained by the corresponding EigenFMD. The lower-right plot shows the accumulated percentage of variance versus the number of EigenFMD modes taken into account. EigenFMD modes were computed using the ROBPCA method.
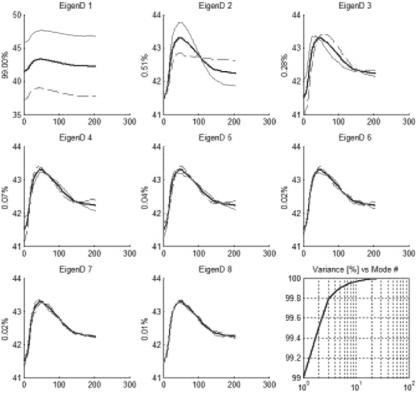
Computerized Analysis and Vasodilation Parameterization of FMD |
251 |
A similar analysis can be performed by applying the ROBPCA technique to the curves of absolute diameter. This analysis can now reveal correlations between risk factors and the absolute vessel diameter as evidenced by some previous clinical studies. To this end, we manually measured the diameter of the reference frame of each subject, r, using the technique presented in Appendix 5.8. Subsequently, each vector ci was unrelativized according to cia = ci · r. Figure 5.13 shows the corresponding modes, which are referred as EigenD
Figure 5.13: Representation of the variation explained by the first eight EigenD modes. The average diameter curve, and the curves resulting of adding/subtracting the corresponding EigenD mode of variation to the mean, weighted by one standard deviation, are plotted in bold line and in thin solid/dashed lines, respectively. The y-axis label of each EigenD plot indicates the percentage of variance explained by the corresponding eigenmode. The lower-right plot shows the accumulated percentage of variance versus the number of EigenD modes taken into account. EigenD modes were computed using the ROBPCA method [33].