
- •Contents
- •Preface
- •1 Introduction: the significance of radiobiology and radiotherapy for cancer treatment
- •2 Irradiation-induced damage and the DNA damage response
- •3 Cell death after irradiation: how, when and why cells die
- •4 Quantifying cell kill and cell survival
- •5 Dose–response relationships in radiotherapy
- •6 Linear energy transfer and relative biological effectiveness
- •7 Tumour growth and response to radiation
- •8 Fractionation: the linear-quadratic approach
- •9 The linear-quadratic approach in clinical practice
- •10 Modified fractionation
- •11 Time factors in normal-tissue responses to irradiation
- •12 The dose-rate effect
- •13 Pathogenesis of normal-tissue side-effects
- •14 The volume effect in radiotherapy
- •15 The oxygen effect and fractionated radiotherapy
- •16 The tumour microenvironment and cellular hypoxia responses
- •17 Therapeutic approaches to tumour hypoxia
- •18 Combined radiotherapy and chemotherapy
- •19 Retreatment tolerance of normal tissues
- •20 Molecular image-guided radiotherapy with positron emission tomography
- •21 Molecular-targeted agents for enhancing tumour response
- •22 Biological response modifiers: normal tissues
- •23 Molecular targeting and patient individualization
- •24 Protons and other ions in radiotherapy
- •25 Second cancers after radiotherapy
- •Glossary of terms in radiation biology
- •Index
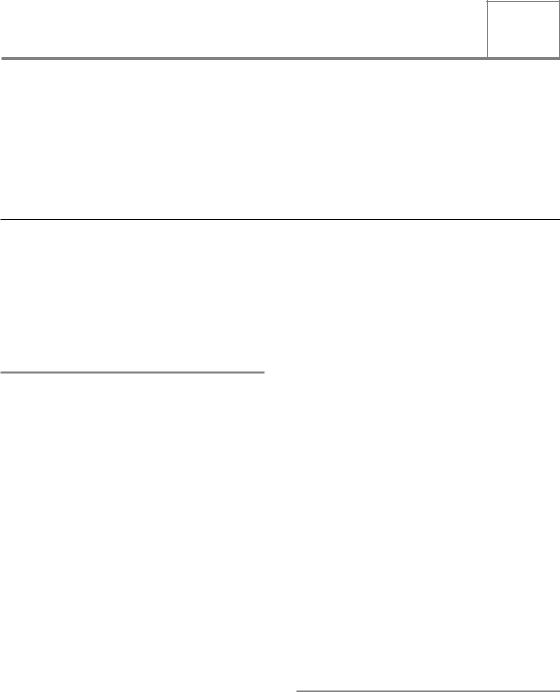
11
Time factors in normal-tissue responses to irradiation
WOLFGANG DÖRR
11.1 |
Introduction |
149 |
11.6 |
Consequential late effects |
155 |
11.2 |
Clinical observations |
149 |
11.7 |
Conclusions |
155 |
11.3 |
Experimental observations |
150 |
Key points |
155 |
|
11.4 |
Mechanisms of repopulation |
151 |
Bibliography |
155 |
|
11.5 |
Regulation of repopulation |
154 |
Further reading |
157 |
|
|
|
|
|
|
|
11.1 INTRODUCTION
The radiation exposure of normal tissues must be considered over a wide range of overall treatment durations (Fig. 11.1). First, on a short scale of minutes to hours, incomplete recovery of sublethal damage may reduce the radiation tolerance of a tissue. Second, over a range of days to weeks (i.e. during a course of fractionated radiotherapy with varying overall treatment times) radiation-induced tissue regeneration (‘repopulation’) may modulate radiation tolerance. This radiation-induced regeneration response is seen in early-responding tissues. Third, over a range of months to years, long-term restoration can occur in some tissues, which renders them more resistant to re-irradia- tion. Also, long-term progression of the damage can occur in other tissues, which causes decreased re-irradiation tolerance.
The impact of incomplete recovery within short time-intervals is covered in the chapters on fractionation and the linear-quadratic (LQ) approach (see Chapters 8 and 9). The changes in radiation tolerance that may occur in intervals that are clearly longer than the general duration of a radiotherapy course are discussed in Chapter 19 on retreatment tolerance. Therefore, this chapter
describes the impact of repopulation processes on early radiation effects.
Repopulation is the term used to describe the regeneration response of early-reacting tissues to fractionated irradiation, which results in an increase in radiation tolerance with increasing overall treatment time. The biological basis of repopulation is a complex restructuring of the proliferative organization of the tissue. A number of clinical and experimental observations can assist in illuminating the biological mechanisms underlying the repopulation processes. The majority of investigations have been performed in oral mucosa as the dose-limiting early side-effect in head and neck cancer radiotherapy, and hence this tissue will be mainly used as a model for the description of radiation-induced repopulation in earlyresponding normal tissues.
11.2 CLINICAL OBSERVATIONS
A number of clinical studies with accelerated radiotherapy protocols (i.e. with a shortened overall treatment time) have resulted in an aggravation of early radiation side-effects. The most prominent example is the CHART (continuous
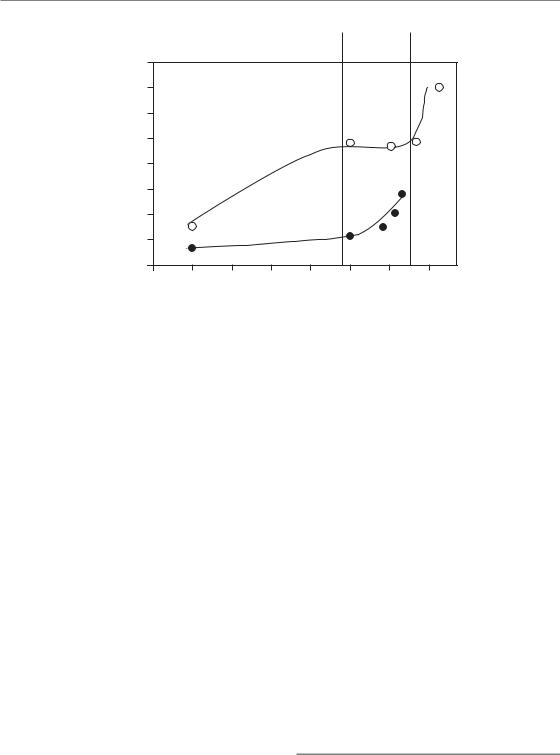
150 Time factors in normal-tissue responses to irradiation
Isoeffective dose (Gy)
|
|
|
|
|
|
Long-term |
160 |
Recovery kinetics |
|
Repopulation |
restoration |
||
|
|
|
|
|
|
|
140 |
|
|
|
|
|
|
120 |
|
|
|
|
|
|
100 |
|
|
|
|
|
|
80 |
Spinal cord |
|
|
|
|
|
|
|
|
|
|
|
|
60 |
|
|
|
|
|
|
40 |
|
|
|
|
|
|
20 |
Oral mucosa |
|
|
|
|
|
|
|
|
|
|
|
|
0 |
|
|
|
|
|
|
0.00001 0.0001 |
0.001 |
0.01 |
0.1 |
1 |
10 |
100 |
|
|
Time interval (days) |
|
|
Figure 11.1 Changes in normal tissue tolerance with time. The figure compiles data for mouse oral mucosa (Dörr and Kummermehr, 1990; Dörr et al., 1993) and for rat spinal cord (Ruifrok et al., 1992; Landuyt et al., 1997). From the original data, isoeffective doses in 3-Gy fractions have been calculated using α/β ratios of 2 Gy for spinal cord and
11 Gy for oral mucosa. Note the log scale of the abscissa. At short durations of radiation exposure ( 1 day), increasing completeness of recovery from sublethal damage increases tissue tolerance. At intermediate intervals from 1 day to several weeks (i.e. the duration of radiotherapy) tolerance increases by repopulation in early-responding tissues such as oral mucosa but not in late-responding tissues such as spinal cord. For long intervals clearly beyond the overall treatment time in radiotherapy, an increase in radiation tolerance by long term restoration is seen in some (e.g. spinal cord), but not all, late-responding tissues.
hyperfractionated accelerated radiation therapy) head and neck trial, where a total dose of 54 Gy was administered in 36 fractions in only 12 days, compared with 66 Gy given in 33 fractions in 6.5 weeks in the control arm (Dische et al., 1997). A significant shift of oral mucosal effects towards more severe, confluent reactions was observed, resulting in an incidence of 73 per cent with CHART versus 43 per cent with the conventional fractionation. Similarly, the EORTC 22851 trial, comparing 72 Gy in 5 weeks with 70 Gy given conventionally in 7 weeks, resulted in a clear increase in the rate of confluent mucositis during as well as 6 weeks after the end of radiotherapy in the accelerated arm (Horiot et al., 1997).
Oral mucositis heals in a much higher proportion of patients during the last treatment weeks compared with earlier times, when doses below 2 Gy are administered (Fletcher et al., 1962). This indicates a time delay before repopulation becomes effective. Furthermore, these data show that the
repopulation capacity is limited by the daily or weekly radiation dose. Oral mucositis has also been studied in split-course regimens (Maciejewski et al., 1991). In a first radiotherapy series with 32 Gy in 12 days, 90 per cent of the patients developed confluent reactions. After a split of 9–13 days, which was introduced to allow for healing of mucositis, a second series of radiotherapy (34–38 Gy/12–14 days) was administered. None of the same patients developed confluent mucosal reactions in the second series, despite the higher dose. This again indicates that the onset of repopulation occurs within the first weeks after the start of radiotherapy.
11.3 EXPERIMENTAL OBSERVATIONS
Changes in radiation tolerance with increasing overall treatment time have been studied in a number of early-responding tissues, such as
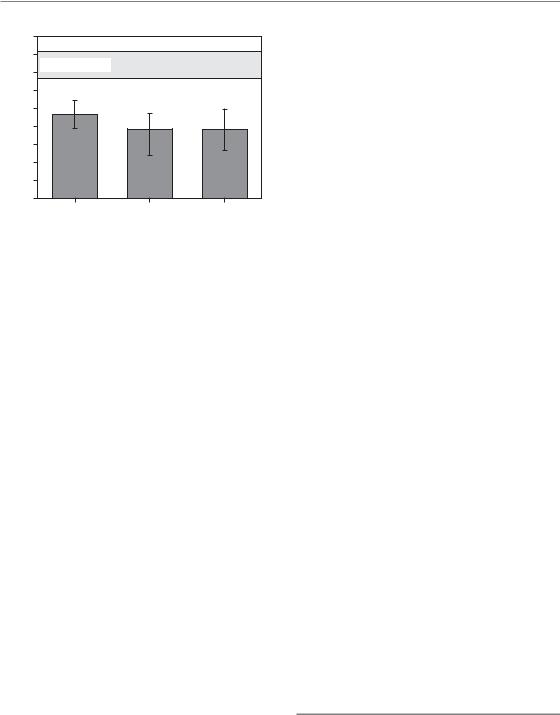
Mechanisms of repopulation 151
|
18 |
|
|
|
16 |
|
|
(Gy) |
Top-up dose only |
|
|
14 |
|
|
|
irradiation |
12 |
|
|
10 |
|
|
|
-up |
8 |
|
|
for top |
|
|
|
6 |
|
|
|
50 |
4 |
|
|
ED |
|
|
|
|
|
|
|
|
2 |
|
|
|
0 |
|
|
|
1 |
2 |
3 |
Treatment weeks
Figure 11.2 Changes in mucosal radiation tolerance with overall treatment time. Mouse tongue mucosa was irradiated with 5 3 Gy/week over 1, 2 or 3 weeks, followed by graded test doses (Dörr and Kummermehr, 1990). Irradiation over 1 week decreased the ED50 (dose at which mucosal ulceration occurs in 50 per cent of the animals) from about 15 Gy in control animals to 9.3 Gy, the difference reflecting the biological effect of 5 3 Gy. Despite administration of a further 15 Gy per week, the ED50 after 2 weeks was only slightly lower (7.7 Gy) and no further reduction was found after the third treatment week (ED50 7.6 Gy). Error bars indicate 95% confidence intervals. Reprinted with permission from Elsevier.
mouse epidermis, rat epidermis, pig epidermis, mouse lip mucosa and mouse tongue mucosa (Dörr, 2003a; Hopewell et al., 2003). One example is illustrated in Fig. 11.2 in which oral mucosa was irradiated with 5 3 Gy/week over 1, 2 or 3 weeks (Dörr and Kummermehr, 1990). Irradiation over 1 week clearly decreased the ED50 (dose, at which a response, i.e. ulceration, is expected in 50% of the animals) for the terminating test irradiation, which reflects residual tissue tolerance. However, despite ongoing irradiation in weeks 2 and 3, no further reduction in the test ED50 was found.
A similar pattern of the time-course of the changes in radiation tolerance during radiotherapy was found in most other tissues studied (Fig. 11.3). Tolerance remained constant within the initial treatment period, and subsequently increased almost linearly. The time of onset of this increase was tissue dependent at 5–7 days in mouse oral mucosa and skin, and 20–30 days in rat and pig skin (Hopewell et al., 2003). The initial drop at short treatment intervals can be related to changes in
the capacity for recovery of sublethal damage, which have been reported for these early-responding tissues (Dörr et al., 2000).
The capacity for repopulation, once these compensatory processes have started, was estimated by (Dörr, 2003a) in terms of the dose (number of 2-Gy fractions) compensated per day. In human oral mucosa, 0.5–1.0 fractions are counteracted per day, thus confirming the clinical results on mucositis healing despite ongoing radiotherapy (see above). These experimental data indicate similar numbers – close to one fraction of 2 Gy/day – for most tissues, with a few possible exceptions (e.g. mouse epidermis; Denekamp, 1973), which could be related to experimental design. In some experiments, where various weekly doses were applied, a dependence of the repopulation rate on the dose intensity (Gy/week) was observed.
In oral mucosa, the functional measurements of radiation tolerance have been supplemented by detailed histological assessments of changes in mucosal cell density and proliferation (Dörr and Kummermehr, 1990; Dörr, 1997). A reduction in cell number to approximately 70 per cent occurred during the first week of daily fractionated treatment. Subsequently, constant to increasing cell numbers were found, despite continuing irradiation at the same dose intensity. In good agreement with this, mucosal proliferation was significantly suppressed during the first week, but subsequently returned to subnormal to near-normal values (Dörr, 1997). Similar observations have been made in other tissues (Shirazi et al., 1995). In human oral mucosa, a reduction of cell production and consequently a steep decline in cell numbers were observed over the initial week of radiotherapy (Dörr et al., 2002). Subsequently, proliferation rates were partly restored and cell depletion was significantly slower.
11.4 MECHANISMS OF REPOPULATION
Three important observations have been made in both clinical and experimental studies:
1.dose is compensated with increasing overall treatment time once repopulation has become effective;
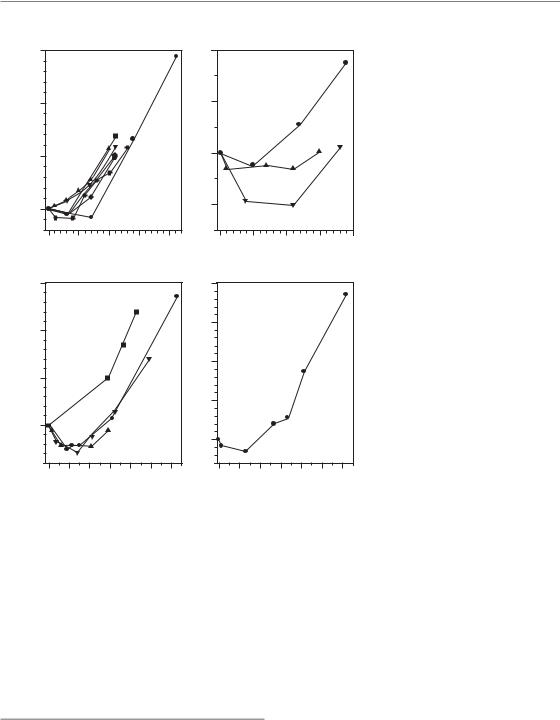
152 Time factors in normal-tissue responses to irradiation
Relative isoeffective dose (% of single dose EQD2)
Mouse oral mucosa |
Mouse skin |
250 |
|
|
|
|
140 |
|
|
|
|
200 |
|
|
|
7 |
120 |
|
|
8 |
|
|
|
|
|
|
|
|
|||
|
|
2 |
|
|
|
|
|
|
|
|
|
4 |
3 |
|
|
|
|
9 |
|
150 |
|
5 |
|
100 |
|
|
|
||
|
|
|
|
|
|
|
|
|
|
|
|
1 |
|
|
|
|
|
10 |
|
|
|
|
|
|
|
|
|
|
|
100 |
|
|
|
|
80 |
|
|
|
|
|
|
|
|
|
|
|
|
|
|
|
6 |
|
|
|
|
|
|
|
|
0 |
5 |
10 |
15 |
20 |
0 |
5 |
10 |
15 |
20 |
Rat epidermis |
Pig epidermis |
250 |
|
|
|
|
|
|
300 |
|
|
|
|
|
|
|
|
|
|
|
|
12 |
|
|
|
|
|
|
|
200 |
|
|
|
|
|
|
250 |
|
|
|
|
|
|
|
|
|
|
|
|
|
|
|
|
|
|
|
|
|
|
|
11 |
|
|
|
|
|
|
|
|
15 |
|
|
|
|
|
|
13 |
|
200 |
|
|
|
|
|
|
150 |
|
|
|
|
|
|
|
|
|
|
|
|
|
|
|
|
|
|
|
|
|
|
|
|
|
|
|
|
|
|
|
|
|
|
150 |
|
|
|
|
|
|
100 |
|
|
|
|
|
|
|
|
|
|
|
|
|
|
|
|
14 |
|
|
|
100 |
|
|
|
|
|
|
|
|
|
|
|
|
|
|
|
|
|
|
|
|
0 |
10 |
20 |
30 |
40 |
50 |
60 |
0 |
10 |
20 |
30 |
40 |
50 |
60 |
|
|
|
|
|
Overall treatment time (days) |
|
|
|
Figure 11.3 Time-course of repopulation: experimental results. From reports in the literature of studies with variations in overall treatment time, the isoeffective EQD2 (equivalent dose in 2 Gy fractions) has been calculated for the individual overall treatment times using an α/β ratio of 10 Gy. Note the different time scales of the abscissae. Treatment protocols and references are: 1, 2F (Ang et al., 1985); 2, 2 5F (Ang et al., 1985); 3, 10F (Ang et al., 1985);
4, 13 Gy 1F (Dörr and Kummermehr, 1990); 5, 10 Gy 1F (Dörr and Kummermehr, 1990); 6, 8 Gy 1F (Dörr and Spekl, unpublished); 7,
5 3 Gy/week (Dörr and Kummermehr, 1990); 8, 5 3 Gy/wk (Shirazi et al., 1995); 9, 5 3 Gy/week (Denekamp, 1973); 10, 2F (Ang et al., 1984); 11,
5 3 Gy/week (van Rongen and Kal, 1984); 12, 10F (Moulder and Fischer, 1976); 13, 3F/week (Moulder and Fischer, 1976); 14, 5F/week (Moulder and Fischer, 1976); 15, 2F (van den Aardweg et al., 1988).
2.the rate at which this compensation occurs is in the range of 5 2 Gy/week;
3.the loss of cells is counteracted after the lag phase before repopulation starts, resulting in more or less constant cell numbers.
In general, the mechanisms of repopulation that can explain these observations can be described by the following three As: asymmetry loss, acceleration, and abortive divisions (Dörr, 1997).
Asymmetry loss
According to the stem cell concept (see Chapter 13), the radiation tolerance of a tissue is defined by the number of tissue stem cells and their intrinsic radiosensitivity. Hence, radiation tolerance must decrease during fractionated irradiation
according to the daily stem cell kill. This is clearly seen during the time lag before the onset of repopulation. However, after repopulation has started, the effect of at least part of the radiation dose is counteracted. This indicates that new stem cells must be produced to replace those sterilized by the irradiation.
In unperturbed tissues, stem cells divide on average into one new stem cell and one differentiating cell (see Chapter 13). These divisions are called asymmetrical because two different cells are generated. In this setting, the number of stem cells in each cell generation remains constant, independent of the proliferation rate. For additional production of new stem cells, as postulated on the basis of dose compensation during repopulation, stem cell divisions must result in two stem cell daughters, a pattern that is depicted as symmetrical division.
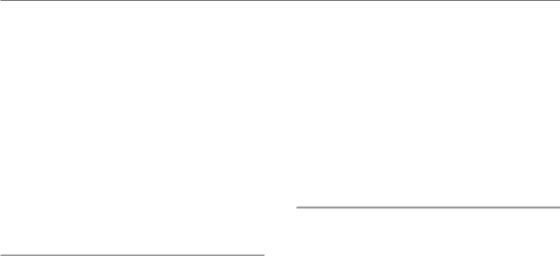
Mechanisms of repopulation 153
Therefore, an asymmetry loss of stem cell divisions is one essential mechanism underlying repopulation in normal tissues. This loss can be complete, with two stem cell daughters from each division, or incomplete, with, on average, less than two, but more than one, stem cell generated per stem cell division. A fraction of these divisions, presumably of cells with gross radiation-induced chromosomal damage, are abortive and can be observed histologically as abnormal mitotic figures (Dörr, 1997). These result in binucleate or multinucleate cells.
Acceleration of stem cell proliferation
As illustrated earlier, human oral mucosa and other tissues are able to compensate weekly doses of about five times 0.5–1.0 fractions of 2 Gy. For this to occur, assuming the surviving fraction of the stem cells after each radiation fraction to be about 0.5, five symmetrical divisions are needed within 7 days. This requires an average cell-cycle time of 1.4 days. Compared with cell-cycle times of at least 3.5 days in unperturbed tissue (Dörr, 1997), this indicates clear acceleration of stem cell proliferation as the second mechanism of repopulation. Cell-cycle times must be even shorter if the asymmetry loss is incomplete, or if higher doses (more stem cell kill) are compensated. The detailed interrelation between surviving fraction, number of symmetrical divisions between fractions and the proportion of symmetrical divisions is discussed in Dörr (1997).
The degree to which the stem cell proliferation is accelerated is highly dependent on the radiation dose administered (i.e. on the daily or weekly stem cell kill; Dörr, 2003a). For example, in oral mucosa irradiated with 5 3.5 Gy/week, this dose was completely counteracted by repopulation in weeks 2 and 3 (Dörr and Kummermehr, 1990). However, during treatment with 5 2.5 Gy/week, repopulation was exactly adjusted to compensate this lower dose, despite the clearly higher regenerative capacity.
In the vast majority of tissues, no specific marker yet exists that would allow for the specific identification of stem cells. Therefore, cell kinetic studies can only assess proliferation of the entire cell population. After the lag phase of repopulation, stem cells constitute only a minor fraction of the general
population, and hence such studies are not suitable to identify the acceleration at the stem cell level. However, in the intestinal crypts, which have well-defined localization of the stem cell population, a significant shortening of the stem cell-cycle time after irradiation has indeed been described (Withers, 1970), which reflects accelerated repopulation, albeit after single doses.
Abortive divisions
After the onset of repopulation, the overall cell number in the tissue remains at a near-constant, although reduced level. In contrast, it has been shown that differentiation and cell loss (e.g. at the surface of mucosal tissues) continue at a normal, physiological rate (Dörr et al., 1996). Also, cell production, which can be directly measured, continues, which is indirectly reflected by the lack of change in cell numbers (Dörr et al., 1994). Hence the question arises of whether this cell production can be based only on the surviving stem cells.
In unperturbed mucosa, with a relative stem cell number of 100 per cent, the cells proliferate with a cell cycle time of at least 3.5 days (Dörr et al., 1994). A dose of 5 2 Gy during the first treatment week, before repopulation sets in, reduces the stem cell number to clearly below 10 per cent. Hence, to result in the same number of cells as in controls, the remaining stem cells would have to proliferate with a cycle time of only a few hours (Dörr et al., 1994; Dörr, 1997). This is extremely unlikely on the basis of epithelial biology, which indicates a minimum cell-cycle time of 10–12 hours. Therefore, cells must be produced from other sources.
It has been shown in vitro (see Chapter 3) that ‘sterilized’ cells can undergo a limited number of divisions even after high doses of radiation. It can therefore be assumed, and indirectly concluded from experimental studies (Dörr et al., 1996), that similar, so-called abortive, divisions of sterilized or doomed cells can also occur in vivo. This limited proliferative activity results in cells that undergo near-normal differentiation, and hence counteract the ongoing cell loss. Quantitatively, in oral mucosa, the radiation-sterilized cells on average have to undergo two or three abortive divisions each to account for the cell production measured.
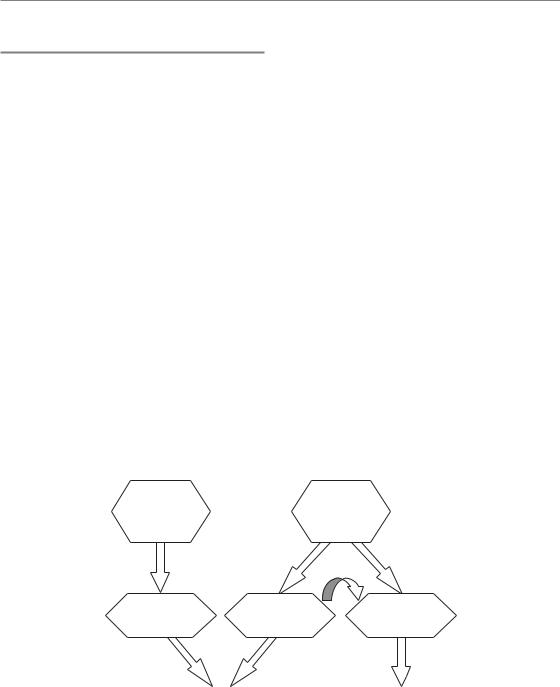
154 Time factors in normal-tissue responses to irradiation
11.5 REGULATION OF REPOPULATION
The time at which repopulation processes become effective, is tissue-specific (Fig. 11.3) and we may assume that it correlates with the turnover time of the tissue, and therefore the rate at which cells are lost. Reduced cell numbers may result in changes in intercellular communication or altered cell–matrix interactions (see Chapter 13). This correlation between tissue turnover and lag time of repopulation, however, is only weak (Dörr, 2003a), so other factors must also be important.
It has been demonstrated that the lag time before repopulation begins is shorter, if the radiation dose is higher (Dörr and Kummermehr, 1990; Dörr, 2003a). This suggests that the rate at which stem cells are depleted may regulate the onset of repopulation. Autoregulatory processes within the stem cell compartment have also been suggested for intestinal crypts (Paulus et al., 1992). The reduction in stem cell numbers can result in (still unidentified) intercellular signalling, which eventually prevents the daughter cells of the stem cell divisions from undergoing differentiation.
The regulation of the asymmetry loss is a very precise and rapidly responding mechanism. During
short treatment breaks, even during weekends, the overall cell production clearly increases (Dörr et al., 1994). However, the radiation tolerance of the tissue during these breaks remains almost constant, indicating that the cells that are produced are not stem cells (Dörr, 2003a). Hence, despite stimulated symmetrical stem cell divisions during the daily fractionated treatment, the stem cells quickly return to asymmetrical divisions if one radiation fraction is missing.
Acceleration of stem cell divisions may be caused by overall cell depletion and by impairment of the epithelial barrier function (Dörr et al., 1994; Shirazi et al., 1995), which may increase the normal signals that physiologically regulate proliferation. This hypothesis is supported by increased proliferation rates after chemical ablation of superficial mucosal material, which has been observed in mice and humans, or after mechanical ablation (‘tape stripping’) in rodents. However, this increased proliferation rate is not associated with increased tolerance to single-dose irradiation (i.e. increased stem cell numbers). This indicates that acceleration has occurred independently of the asymmetry loss. It must be assumed that sterilized cells initially continue to proliferate at
Stem cell |
Tissue |
depletion |
hypoplasia |
Stem cells |
Stem cells |
Damaged cells |
asymmetry loss |
acceleration |
abortive divisions |
Dose |
|
Compensation |
compensation |
|
of cell loss |
|
|
|
Figure 11.4 Regulation of repopulation. Stem cell depletion, via autoregulatory processes, results in the loss of the division asymmetry of the stem cells. Tissue hypoplasia controls the acceleration of stem cell division and presumably also the rate of abortive division, which is indirectly also defined by the proliferation rate of the stem cells. Asymmetry loss and acceleration of stem cell division account for compensation of dose, while abortive divisions of sterilized cells counteract the overall cell loss to maintain tissue function.
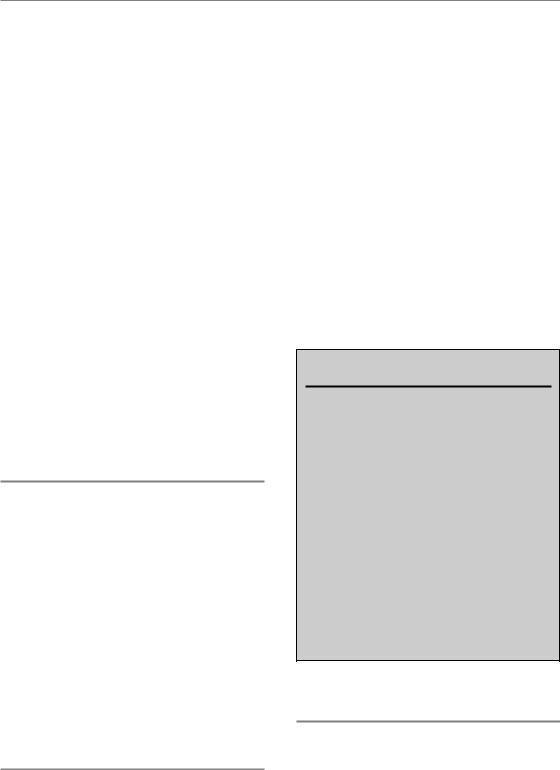
Bibliography 155
nearly the rate of the stem cell divisions before chromosomal damage accumulates and decreases the proliferation rate.
In combination with the asymmetry loss (e.g. by fractionated irradiation) artificially increased stem cell proliferation rates may shorten the lag time before repopulation becomes effective. This has been demonstrated for chemical ablation in oral mucosa (Maciejewski et al., 1991). A similar mechanism has been suggested for the increase in mucosal radiation tolerance after administration of keratinocyte growth factor (KGF, palifermin) in preclinical and clinical studies as reviewed in Dörr (2003b), and summarized in Chapter 22.
In conclusion (Fig. 11.4), the asymmetry loss in the stem cell compartment is regulated independently of the acceleration of proliferation, presumably by autoregulatory processes on the basis of stem cell depletion. Acceleration of the stem cell divisions is controlled by tissue hypoplasia. The stem cell proliferation rate then translates into the initial rate of abortive divisions of sterilized cells. However, the doomed cells may also directly respond to paracrine signals released owing to tissue hypoplasia.
11.6 CONSEQUENTIAL LATE EFFECTS
Consequential late effects are chronic normaltissue complications, which are influenced by the extent (i.e. severity and/or duration, of the early response in the same tissue or organ; Dörr and Hendry, 2001), as explained in Chapter 13. As the early response depends on the overall treatment time on the basis of repopulation processes, the same is therefore true for the corresponding consequential late effects. This has been demonstrated in experimental studies for intestinal fibrosis, and in clinical studies for skin telangiectasia, and for late (mucosa related) side-effects after head and neck irradiation, as reviewed in Dörr and Hendry (2001).
11.7 CONCLUSIONS
Repopulation processes that occur in earlyresponding normal tissues follow a very complex
biology. The major mechanisms are asymmetry loss and acceleration of stem cell division, as well as abortive divisions of sterilized cells. A number of parameters that modulate the repopulation response, such as dose intensity (weekly dose), have been identified. However, the precise regulatory mechanisms of tissue repopulation during fractionated radiotherapy still remain largely unclear. These regulatory signals would be attractive targets for biologically based modulation of radiation effects in early-responding normal tissues.
Taking into consideration all the underlying biology of repopulation indicates that a time factor, in the sense of compensated dose, should be included in mathematical models of normal-tissue complications only with great care and caution, as these models currently do not include the dominating repopulation-modifying factors.
Key points
1.The radiation tolerance of early-responding normal tissues increases with increasing overall treatment time.
2.This phenomenon is depicted as repopulation.
3.Repopulation starts after a tissue-specific lag time.
4.The biological basis is a complex restructuring of the proliferative tissue organization.
5.This includes asymmetry loss and acceleration of stem cell proliferation.
6.Abortive divisions of doomed cells significantly contribute to cell production.
7.Tissue hypoplasia controls stem cell acceleration and abortive divisions, while the asymmetry loss is regulated by stem cell depletion.
■BIBLIOGRAPHY
Ang KK, Landuyt W, Rijnders A, van der Schueren E (1984). Differences in repopulation kinetics in mouse skin during split course multiple fractions per day (MFD) or daily fractionated irradiations. Int J Radiat Oncol Biol Phys 10: 95–9.

156 Time factors in normal-tissue responses to irradiation
Ang KK, Xu FX, Vanuytsel L, van der Schueren E (1985). Repopulation kinetics in irradiated mouse lip mucosa: the relative importance of treatment protraction and time distribution of irradiations.
Radiat Res 101: 162–9.
Denekamp J (1973). Changes in the rate of repopulation during multifraction irradiation of mouse skin. Br J Radiol 46: 381–7.
Dische S, Saunders M, Barrett A, Harvey A, Gibson D, Parmar M (1997). A randomised multicentre trial of CHART versus conventional radiotherapy in head and neck cancer. Radiother Oncol 44: 123–36.
Dörr W (1997). Three As of repopulation during fractionated irradiation of squamous epithelia: asymmetry loss, acceleration of stem-cell
divisions and abortive divisions. Int J Radiat Biol 72: 635–43.
Dörr W (2003a). Modulation of repopulation processes in oral mucosa: experimental results. Int J Radiat Biol 79: 531–7.
Dörr W (2003b). Oral mucosa: response modification by keratinocyte growth factor. In: Nieder C, Milas L, Ang KK (eds) Modification of radiation response: cytokines, growth factors and other biological targets. Berlin: Springer-Verlag, 113–22.
Dörr W, Hendry JH (2001). Consequential late effects in normal tissues. Radiother Oncol 61: 223–31.
Dörr W, Kummermehr J (1990). Accelerated repopulation of mouse tongue epithelium during fractionated irradiations or following single doses.
Radiother Oncol 17: 249–59.
Dörr W, Breitner A, Kummermehr J (1993). Capacity and kinetics of SLD repair in mouse tongue epithelium.
Radiother Oncol 27: 36–45.
Dörr W, Emmendorfer H, Haide E, Kummermehr J (1994). Proliferation equivalent of ‘accelerated repopulation’ in mouse oral mucosa. Int J Radiat Biol 66: 157–67.
Dörr W, Emmendorfer H, Weber-Frisch M (1996). Tissue kinetics in mouse tongue mucosa during daily fractionated radiotherapy. Cell Prolif 29: 495–504.
Dörr W, Brankovic K, Hartmann B (2000). Repopulation in mouse oral mucosa: changes in the effect of dose fractionation. Int J Radiat Biol 76: 383–90.
Dörr W, Hamilton CS, Boyd T, Reed B, Denham JW (2002). Radiation-induced changes in cellularity and proliferation in human oral mucosa. Int J Radiat Oncol Biol Phys 52: 911–7.
Fletcher GH, MacComb WS, Shalek RJ (1962).
Radiation therapy in the management of cancer of the oral cavity and oropharynx. Springfield: Charles Thomas.
Hopewell JW, Nyman J, Turesson I (2003). Time factor for acute tissue reactions following fractionated irradiation: a balance between repopulation and enhanced radiosensitivity. Int J Radiat Biol 79: 513–24.
Horiot JC, Bontemps P, van den Bogaert W et al. (1997). Accelerated fractionation (AF) compared to conventional fractionation (CF) improves loco-regional control in the radiotherapy of advanced head and neck cancers: results of the EORTC 22851 randomized trial. Radiother Oncol 44: 111–21.
Landuyt W, Fowler J, Ruifrok A, Stuben G, van der Kogel A, van der Schueren E (1997). Kinetics of repair in the spinal cord of the rat. Radiother Oncol 45: 55–62.
Maciejewski B, Zajusz A, Pilecki B et al. (1991). Acute mucositis in the stimulated oral mucosa of patients during radiotherapy for head and neck cancer.
Radiother Oncol 22: 7–11.
Moulder JE, Fischer JJ (1976). Radiation reaction of rat skin. The role of the number of fractions and the overall treatment time. Cancer 37: 2762–7.
Paulus U, Potten CS, Loeffler M (1992). A model of the control of cellular regeneration in the intestinal crypt after perturbation based solely on local stem cell regulation. Cell Prolif 25: 559–78.
Ruifrok AC, Kleiboer BJ, van der Kogel AJ (1992). Reirradiation tolerance of the immature rat spinal cord. Radiother Oncol 23: 249–56.
Shirazi A, Liu K, Trott KR (1995). Epidermal morphology, cell proliferation and repopulation in mouse skin during daily fractionated irradiation. Int J Radiat Biol 68: 215–21.
van den Aardweg GJ, Hopewell JW, Simmonds RH (1988). Repair and recovery in the epithelial and vascular connective tissues of pig skin after irradiation. Radiother Oncol 11: 73–82.
van Rongen E, Kal HB (1984). Acute reactions in rat feet exposed to multiple fractions of X-rays per day.
Radiother Oncol 2: 141–50.
Withers HR (1970). Cellular kinetics of intestinal mucosa after irradiation. In: Burdette WJ (ed.)
Carcinoma of the colon and antecedent epithelium. Springfield: Charles Thomas, 243–57.

Further reading 157
■ FURTHER READING
Bentzen SM (1994). Radiobiological considerations in the design of clinical trials. Radiother Oncol 32: 1–11.
Denekamp J (1975). Changes in the rate of proliferation in normal tissues after irradiation. In: Nygaard OF, Adler HI, Sinclair WK (eds) Radiation research. Biomedical, chemical and physical perspectives. New York: Academic Press, 810–25.
Denham JW, Walker QJ, Lamb DS et al. (1996). Mucosal regeneration during radiotherapy. Trans Tasman Radiation Oncology Group (TROG). Radiother Oncol 41: 109–18.
Trott KR, Kummermehr J (1993). The time factor and repopulation in tumors and normal tissues. Semin Radiat Oncol 3: 115–25.