
Molecular Fluorescence
.pdf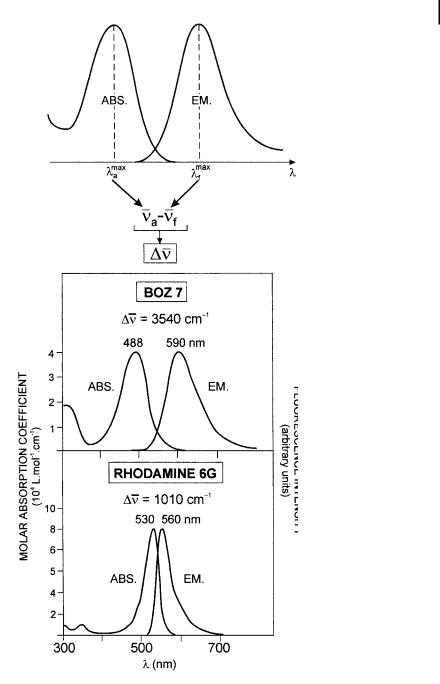
3.4 Effects of molecular structure on fluorescence 55
Fig. 3.3. Definition of the Stokes shift. Examples of Stokes shift: benzoxazinone derivative (BOZ 7) and rhodamine 6G.

563 Characteristics of fluorescence emission
Tab. 3.3. Heavy atom e ect on emissive properties of naphthalene (from Wehry, 1990)
|
FF |
kisc /sC1 |
FP |
tT /s |
Naphthalene |
0.55 |
1:6 106 |
0.051 |
2.3 |
1-Fluoronaphthalene |
0.84 |
5:7 105 |
0.056 |
1.5 |
1-Chloronaphthalene |
0.058 |
4:9 107 |
0.30 |
0.29 |
1-Bromonaphthalene |
0.0016 |
1:9 109 |
0.27 |
0.02 |
1-Iodonaphthalene |
<0.0005 |
>6 109 |
0.38 |
0.002 |
p ! p transitions, i.e. about 10 6 s. Such a slow process cannot compete with the dominant non-radiative processes. This explains the low fluorescence quantum yields of many molecules in which the lowest excited state is n–p in nature. This is the case for most azo compounds and some compounds containing carbonyl groups and nitrogen heterocycles (with pyridine-type nitrogens) (see Sections 3.4.2.3 and 3.4.3).
3.4.2
Substituted aromatic hydrocarbons
The e ect of substituents on the fluorescence characteristics of aromatic hydrocarbons is quite complex and generalizations should be made with caution. Both the nature and position of a substituent can alter these characteristics.
3.4.2.1 Internal heavy atom e ect
In general, the presence of heavy atoms as substituents of aromatic molecules (e.g. Br, I) results in fluorescence quenching (internal heavy atom e ect) because of the increased probability of intersystem crossing. In fact, intersystem crossing is favored by spin–orbit coupling whose e ciency has a Z4 dependence (Z is the atomic number). Table 3.3 exemplifies this e ect.
However, the heavy atom e ect can be small for some aromatic hydrocarbons if:
(i) the fluorescence quantum yield is large so that de-excitation by fluorescence emission dominates all other de-excitation processes; (ii) the fluorescence quantum yield is very low so that the increase in e ciency of intersystem crossing is relatively small; (iii) there is no triplet state energetically close to the fluorescing state (e.g. perylene)10).
3.4.2.2 Electron-donating substituents: COH, COR, CNH2, CNHR, CNR2
In general, substitution with electron-donating groups induces an increase in the molar absorption coe cient and a shift in both absorption and fluorescence spec-
10)The energy di erence between S1 and the accepting triplet state has to be small enough for intersystem crossing to compete e ectively with fluorescence. This is not the case with perylene, as demonstrated by the fact
that its fluorescence quantum yield is nearly identical to those of bromosubstituted perylenes (Dreeskamp H., Koch E. and Zander M. (1975) Chem. Phys. Lett. 31, 251).
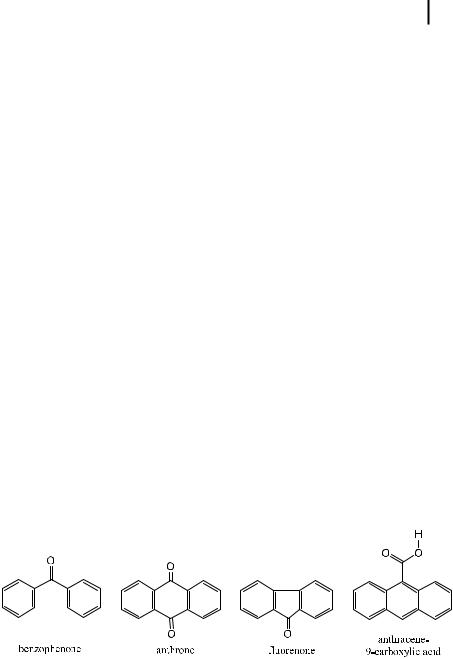
3.4 Effects of molecular structure on fluorescence 57
tra. Moreover, these spectra are broad and often structureless compared to the parent aromatic hydrocarbons (e.g. 1- and 2-naphthol compared to naphthalene).
The presence of lone pairs of electrons on the oxygen and nitrogen atoms does not change the p–p nature of the transitions of the parent molecule. These lone pairs are indeed involved directly in p bonding with the aromatic system, in contrast to the lone pairs of electrons of carbonyl substituents (see Section 3.4.2.3) or heterocyclic nitrogen (see Section 3.4.3). To make the distinction between the two types of lone pairs, Kasha and Rawls suggested using the term l orbital for the lonepair orbitals of aromatic amines and phenols. A significant intramolecular charge transfer character of the relevant transitions is expected for planar aromatic amines and phenol; this is confirmed by the fact that the fluorescence spectra are broad and structureless.
If, for steric reasons, the aNH2 group is twisted out of the plane of the aromatic ring, the degree of conjugation of the l orbitals is decreased, but the transitions corresponding to the promotion of an electron from an l orbital to a p orbital are still di erent (in particular more intense) to the n ! p transitions involving the lone pairs of a carbonyl or nitro group. Departure from coplanarity with the aromatic ring is also pronounced with aOR substituents, whereas an aOH group is nearly coplanar.
The absorption and emission characteristics of phenols and aromatic amines are pH-dependent. These aspects will be discussed in Section 4.5.
3.4.2.3 Electron-withdrawing substituents: carbonyl and nitro compounds
The fluorescence properties of aromatic carbonyl compounds are complex and often di cult to predict.
Many aromatic aldehydes and ketones (e.g. benzophenone, anthrone, 1- and 2- naphthaldehyde) have a low-lying n–p excited state and thus exhibit low fluorescence quantum yields, as explained above. The dominant de-excitation pathway is intersystem crossing (whose e ciency has been found to be close to 1 for benzophenone).
Some aromatic carbonyl compounds have a low-lying p–p excited state and thus have a reasonable quantum yield (e.g. 0.12 for fluorenone in ethanol at 77 K and 0.01 at room temperature). However, if an n–p state lies only slightly higher in energy, the fluorescence quantum yield strongly depends on the polarity of the

58 3 Characteristics of fluorescence emission
solvent (proximity e ect). In fact, in some solvents, the energy of the n–p state can become lower than that of the p–p state. When the polarity and the hydrogen bonding power of the solvent increases, the n–p state shifts to higher energy whereas the p–p state shifts to lower energy. Therefore, intense fluorescence can be observed in polar solvents and weak fluorescence in nonpolar solvents (e.g. xanthone).
When an aromatic molecule has a carboxylic group as a substituent, photophysical e ects due to conformational changes can be observed. For instance, anthracene-9-carboxylic acid exhibits a broad fluorescence spectrum deprived of apparent vibronic bands, in contrast to its absorption spectrum and to both absorption and fluorescence spectra of the conjugate base (similar to the anthracene spectrum). Such a di erence between the fluorescence spectra of the acidic and basic forms can be explained in terms of conformation of the carboxylate group aCOO , which should be almost perpendicular to the ring so that the p system of the anthracene ring is only slightly perturbed. On the contrary, the carboxylic group aCOOH may be in a position close to the coplanarity of the ring; the resulting interaction induces an intramolecular charge-transfer character to the p ! p transition. Charge-transfer fluorescence bands are indeed usually broad and structureless. However, because the absorption spectrum of the acidic form exhibits vibronic bands, the rotation of the aCOOH is likely to be photoinduced.
In general, the fluorescence of aromatic hydrocarbons possessing an aNO2 substituent is not detectable. The existence of a low-lying n ! p transition explains the e cient intersystem crossing process (e.g. for 2-nitronaphthalene, the quantum yield for intersystem crossing is 0.83 in benzene solution at room temperature). Many nitroaromatics are indeed phosphorescent. However, in some cases, the quantum yield for intersystem crossing is significantly less than 1. Therefore, the absence of detectable fluorescence is likely to be due to a high rate of S1 ! S0 internal conversion, which may be related to the considerable charge-transfer character of the excited state, as a result of the strong electron-withdrawing power of the aNO2 group.
It should be mentioned that many nitroaromatics undergo photodegradation. For instance, 9-nitroanthracene is transformed into anthraquinone upon illumination.
3.4.2.4 Sulfonates
The solubility in water of many fluorophores is achieved by grafting sulfonate groups. Fortunately, these groups only slightly a ect the fluorescence characteristics of the parent molecule. In general, there is a small red-shift of the fluorescence spectrum, whose vibrational structure is somewhat blurred, and the fluorescence quantum yield is slightly decreased.
Finally, it should be emphasized that the fluorescence characteristics of aromatic hydrocarbons containing more than one substituent are di cult to predict. These e ects cannot be simply extrapolated from those of individual substituents. For instance, in spite of the presence of a nitro group, o- and m-nitroaniline and 3- nitro-N,N-dimethylaniline exhibit fluorescence.
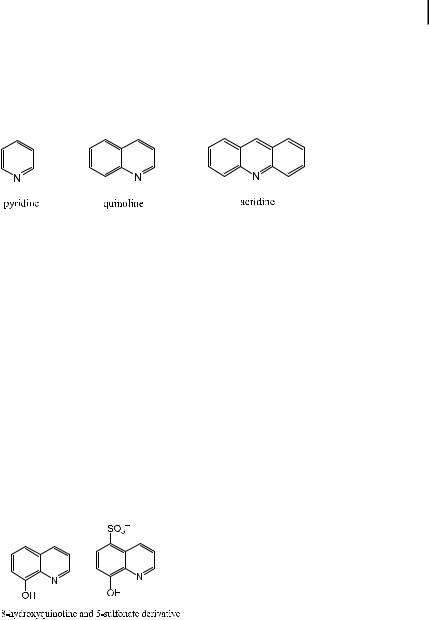
3.4 Effects of molecular structure on fluorescence 59
3.4.3
Heterocyclic compounds
Compounds called azarenes containing one or more heterocyclic nitrogen atoms (e.g. pyridine, quinoline, acridine) have low-lying n ! p transitions, which explains their relatively low fluorescence quantum yields in hydrocarbons.
However, the fluorescence characteristics of these compounds are strongly solvent-dependent. In protic solvents such as alcohols, hydrogen bonds can be formed between the nitrogen atoms and the solvent molecules. This results in an inversion of the lowest-lying n–p and p–p states. As the lowest-lying transition becomes of p ! p character in these solvents, the fluorescence quantum yield is much higher than in hydrocarbon solvents.
It should be noted that the electron density on the nitrogen atom is reduced on excitation, so that the ability to form hydrogen bonds is lower in the excited state. The ground state is thus more stabilized by hydrogen bonding than the excited state. This results in a red-shift of the spectra when going from nonpolar solvents to hydrogen bond donating solvents.
In some heterocyclic compounds such as acridine, the n–p absorption band is di cult to distinguish from the much more intense p–p absorption bands.
There are many interesting derivatives of quinoline and acridine obtained by substitution. In particular, 8-hydroxyquinoline (oxine) is the second complexing agent in importance after EDTA. Sulfonation in position 5 leads to a compound which is soluble in water and that exhibits outstanding fluorogenic properties (i.e. fluorescence enhancement) on complexation with metal ions (e.g. aluminum).
When a heteronitrogen is singly bonded to carbon atoms in a heterocycle, as in pyrrole rings (e.g. indole, carbazole), the transitions involving the non-bonding electrons have properties similar to those of p ! p transitions. In fact, the nonbonding orbital is perpendicular to the plane of the ring, which allows it to overlap the p orbitals on the adjacent carbon atoms. The relatively high fluorescence quantum yield of carbazole and indole can be explained in this way. Tryptophan is an important derivative of indole, whose photophysical properties have been
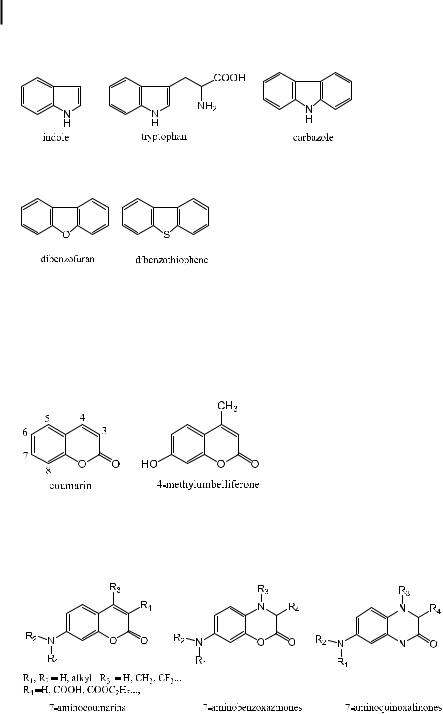
60 3 Characteristics of fluorescence emission
extensively studied because of its importance in fluorescence investigations of proteins.
The properties of the related heterocycles containing oxygen and sulfur (e.g. dibenzofuran, dibenzothiophene) can be similarly interpreted.
Many fluorophores of practical interest are heterocyclic: coumarins, rhodamines, pyronines, fluoresceins, oxazines, etc. A few examples will now be presented.
Coumarin itself has a poor quantum yield, but appropriate substitution leads to fluorescent compounds emitting in the blue–green region (400–550 nm). Substitution in position 4 by a methyl group leads to umbelliferone. 7-Hydroxycoumarins are very sensitive to pH. For example, 4-methyl-7-hydroxycoumarin (4-methyl- umbelliferone) can be used as a fluorescent pH probe (see Chapter 10).
7-Aminocoumarins are of particular interest because they possess an electrondonating group (amino group) conjugated to an electron-withdrawing group (carbonyl group). This results in a photoinduced charge transfer (see Section 3.4.4).
Aminoquinoxalinones and aminobenzoxazinones exhibit similar properties.
The two well-known classes of highly fluorescent dyes, rhodamines and fluoresceins, are derivatives of xanthene.
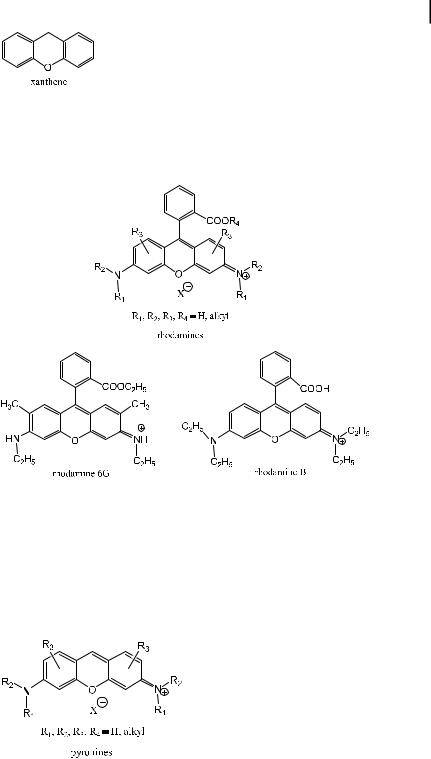
3.4 Effects of molecular structure on fluorescence 61
Rhodamines (e.g. rhodamine 6G, rhodamine B) were among the first fluorescent dyes to be used as laser dyes. In contrast to coumarins, their absorption and emission spectra are quite narrow and the Stokes shift is small. They emit fluorescence in the range 500–700 nm.
It is worth noting that the carboxyphenyl group of rhodamines is only slightly involved in the conjugation of the p electron system because it is almost perpendicular to the xanthenic ring for steric reasons. Thus, replacement of this group by a hydrogen atom should not greatly a ect the photophysical properties. In fact, the resulting compounds, called pyronines, exhibit almost identical properties to those of the corresponding rhodamines: their absorption and emission spectra are shifted by 1–3 nm with respect to the rhodamines, and the Stokes shift is slightly smaller.
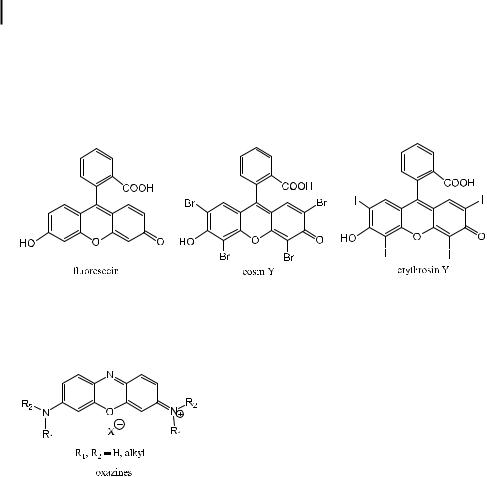
62 3 Characteristics of fluorescence emission
The second family of xanthene dyes is fluorescein and its derivatives. Fluorescein itself is only slightly fluorescent in alcohol solution. In contrast, the alkali salt obtained by addition of alkali exhibits the well-known yellow–green fluorescence characteristic of the fluorescein dianion (uranin). Fluorescein and its derivatives, e.g. eosin Y and erythrosin Y, are known to be very sensitive to pH and can thus be used as pH fluorescent probes (see Chapter 10).
In pyronines, replacement of the carbon atom of the central ring (opposite to the oxygen atom) by a nitrogen atom produces oxazine dyes, which are used as laser dyes emitting in the range 600–750 nm.
3.4.4
Compounds undergoing photoinduced intramolecular charge transfer (ICT) and internal rotation
Excitation of a fluorophore induces the motion of an electron from one orbital to another. If the initial and final orbitals are separated in space, the electronic transition is accompanied by an almost instantaneous change in the dipole moment of the fluorophore. When the latter possesses an electron-donating group (e.g. aNH2, aNMe2, aCH3O) conjugated to an electron-withdrawing group (e.g. >CbO, aCN), the increase in dipole moment can be quite large. Consequently, the excited state reached upon excitation (called the Franck–Condon state or locally excited state, LE) is not in equilibrium with the surrounding solvent molecules if the latter are polar. In a medium that is su ciently fluid, the solvent molecules rotate during the lifetime of the excited state until the solvation shell is in thermodynamic equilibrium with the fluorophore. A relaxed intramolecular charge transfer (ICT) state is then reached.
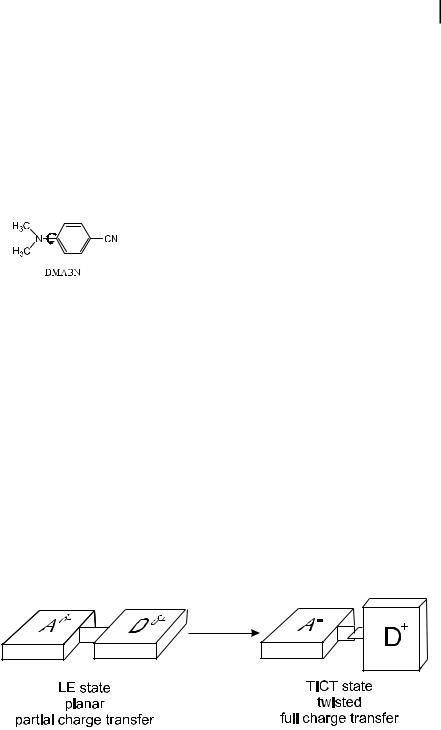
3.4 Effects of molecular structure on fluorescence 63
Such a solvent relaxation explains the increase in the red-shift of the fluorescence spectrum as the polarity of the solvent increases. The e ect of polarity on fluorescence emission will be further discussed in Chapter 7, together with polarity probes. Moreover, when a cation receptor is linked to an intramolecular charge transfer fluorophore so that the bound cation can interact with either the donor group or the acceptor group, the ICT is perturbed; the consequent changes in photophysical properties of the fluorophore can be used for sensing cations (see Section 10.3.3).
Relaxation towards an ICT state may be accompanied by internal rotation within the fluorophore. The prime example of great interest is 4-N,N-dimethylamino- benzonitrile (DMABN).
This molecule has been the object of many studies because, in spite of its simplicity, it exhibits dual fluorescence in polar solvents. This intriguing phenomenon can be explained in the following way (Lippert et al., 1987). In the ground state, the molecule is almost planar, which corresponds to the maximum conjugation between the dimethylamino group and the phenyl ring. According to the Franck– Condon principle, the locally excited state (LE) is still planar, but solvent relaxation takes place with a concomitant rotation of the dimethylamino group until it is twisted at right angles and the conjugation is lost. In the resulting TICT (Twisted Intramolecular Charge Transfer) state, stabilized by the polar solvent molecules, there is a total charge separation between the dimethylamino group and the cyanophenyl moiety (Scheme 3.3).
In addition to the fluorescence band due to emission from the LE state (‘normal’ band), an emission band corresponding to emission from the TICT state is observed at higher wavelengths (‘anomalous’ band) (Figure 3.4).
The twisting assumption can be demonstrated by comparing the fluorescence characteristics of the bridged model compounds 2 and 3 with those of DMABN (1) in a polar solvent: no twist is possible in compound 2 and LE fluorescence is solely
Scheme 3.3
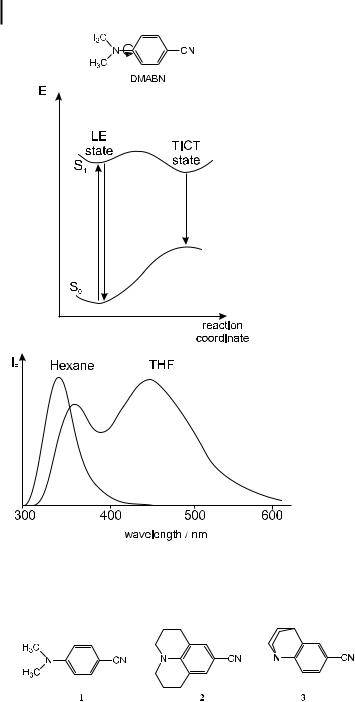
64 3 Characteristics of fluorescence emission
Fig. 3.4. Potential energy diagram of DMABN (top); the reaction coordinate contains both solvent relaxation and rotation of the dimethylamino group. Room temperature fluorescence spectrum in hexane and tetrahydrofurane (bottom) (adapted from Lippert et al., 1987).
observed; the twisted compound 3 exhibits only the TICT fluorescence band. In contrast, DMABN shows both LE and TICT fluorescence.
Internal rotation, accompanying solvent relaxation or not, can occur in many fluorophores, but dual fluorescence and right angle twist (required for full charge