
Engineering and Manufacturing for Biotechnology - Marcel Hofman & Philippe Thonart
.pdfNew matrices and bioencapsulation processes
3. Materials for encapsulation
Any material that can be turned into hydrogels by physicochemical means is in principle suitable for the encapsulation of biocatalysts. The formation of the gel can either be due to ion interaction or chemical bonding or can also be facilitated by thermal solidification. The polymers used can either be of natural origin or synthetic materials.
3.1. NATURAL POLYSACCHARIDES FOR IONOTROPIC GELATION
The classical material for encapsulation is the well-known alginate (Kierstan and Bucke,
1977; Vorlop and Klein, 1983; Smidsrød and Skjåk-Bræk, 1990). The major source of alginate is found in the cell walls and the intracellular spaces of brown seaweed.
The use of alginate as an immobilising agent in most applications rests in its ability to form gels that can develop and set at room temperature by interacting with calcium ions.
Due to this ionotrophic gelation, the reaction conditions are mild, and bacteria, eukaryotic microorganisms and also higher cells show excellent survival rates.
Excessive research has been done on alginate, but the stability of the resulting gel is often too low when working with powerful growing cells like fungi or when the beads are used in a reactor with high shearing strain. Also, the gels are susceptible to calciumleaching what will result in deterioration when working with phosphate or citrate buffered media. Moreover, alginate is readily biodegraded when working under non- sterile conditions and is thus not suitable for bulk-use when sterility cannot be ensured.
Other biopolymers have to be mentioned which have been tested for immobilisation purposes. Pectinate also can gelate in the presence of calcium or, depending on the type of pectinate when heated and cooled down, in the presence of sucrose (Berger and Rühlemann, 1998). Carrageenan molecules in solution aggregate to a double helix, which can be stabilised by interaction with potassium or ammonia (Chibata et a/., 1987). Hence it can be used with phosphate-buffered media which is advantageous compared to alginate. The mass product chitosan is recovered from deacetylation of chitin and is a polycation. Therefore it forms stable hydrogels in the presence of polyanions like e.g. tripolyphosphate (Vorlop and Klein, 1987).
3.2. SYNTHETIC HYDROGELS BY CHEMICAL REACTION
Synthetic gels can be formed by polymerisation or cross-linking of prepolymers or monomers. The disadvantage of these prepolymers is that the chemical reaction is executed in the presence of the microorganisms which causes significant losses in activity in most cases. To circumvent this drawback, efforts were taken to soften the reaction conditions. The development of polycarbamoyl sulphonate (PCS) as a replacement for polyurethanes (PU) is one example (Muscat et al., 1996). By blocking the toxic isocyanate groups during the polymerisation, a less toxic prepolymer can be obtained.
In the industrially employed PEGASUS-process, different polyethylene glycol (PEG) prepolymers are used to immobilise nitrifying sludge (Emori et al., 1996). The cells are protected from the cross-linking reagent during immobilisation by a
301
Ulrich Jahnz, Peter Wittlich, Ulf Prüsse and Klaus-Dieter Vorlop
macromolecular coagulant. The obtained PEG pellets consist of 3 mm blocks and show good mechanical properties. However, due to large dimension, the diffusional properties are not optimal and final specific activity of immobilised biomass is limited.
3.3. HYDROGELS FROM POLYVINYL ALCOHOL
Polyvinyl alcohols (PVA) are hydrophilic polymers whose aqueous solutions are capable of gelling on their own when stored for prolonged time. Hydrogen bonds between hydroxyl groups of neighbouring polymer chains form a non-covalent network. However, this process takes days and the gels obtained at temperatures above 0°C usually are rather weak and thus not suitable.
A different effect is obtained when PVA solution is subjected to freezing (Lozinsky and Plieva, 1998; Lozinsky, 1998). Due to phase separation during the freezing process the formation of hydrogen bonds is enhanced and the resulting hydrogel is significantly stronger. Hydrogels from PVA by this cryogelation are mechanically very stable and show more or less no abrasion when employed in stirred reactors. In gelated form PVA is hardly biodegradable and thus can be used when working under non-sterile conditions. Chemically the hydrogels can be utilised with any physiological compound since they do not dissolve. Merely by heating to above 60°C the hydrogels can be melted.
Parameters influencing the gel-strength are the degree of deacetylation of the used PVA, the chain length of the polymer, its concentration in the solution, and the rate of thawing. Usually concentrations of 7 to 15 % (w/w) of polymer with a molecular weight of about 80 to 100 kDa are used. The slower the thawing process, the more rigid the resulting gel will be. Especially the temperature range of about is crucial for a satisfactory stability and the emerging hydrogel should be thawed with only a few degrees per hour in this scope.
An alternative to the slow-thawing method for the reinforcement of the hydrogel is the multiple freezing-thawing, which is scarcely used since it consumes significant amounts of energy. In both cases the freezing often causes significant loss of microbial activity when living cells are to be immobilised.
Another known means of gelating a PVA-solution is the dripping into boric acid, but the resulting hydrogel is less stable and rather brittle.
4. LentiKats®
As mentioned above the method of cryogelation of PVA often inflicts a loss in biological activity. To counteract the stress we developed a method that allows the gelation of PVA-solutions at room temperature by means of controlled partial drying. Due to their characteristic lenticular shape the resulting particles are named LentiKats®.
4.1. DESCRIPTION OF PROPERTIES
Ready-to-use LentiKat®Liquid solution is mixed with the biocatalyst, i.e. cells or enzyme preparation, and small droplets are floored on a suitable surface. By exposing
302
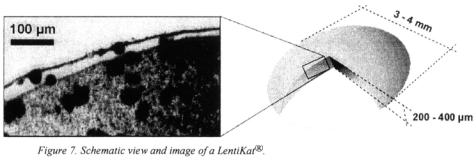
New matrices and bioencapsulation processes
these droplets to air, the water starts to evaporate and thus leads to enhanced formation of hydrogen bonds. Once about 70% of the polymer-biocatalyst-solution have been removed, the hydrogel is stable enough and can be re-swollen in a stabilising solution before the ready LentiKats® are employed.
The particles formed by this procedure combine the advantages of both large and small beads: On the one hand side they measure about 3 to 4 mm in diameter and can be retained by established sieve technology or rapidly by settling. On the other hand they are only 200 to thick and thus cause hardly any diffusional limitations to the enclosed biocatalysts.
Since LentiKats® are based on polyvinyl alcohol they have the same properties as discussed for the PVA cryogels. But based on the very mild encapsulation conditions, very high survival rates can be obtained. The complete immobilisation procedure takes place in less than one hour and also the stress of the partial drying is tolerable for most organisms as shown below.
4.2. PRODUCTION DEVICES FOR LABAND TECHNICAL SCALE
For first trials it is sufficient to form the droplets by a simple lab-syringe on a standard petri dish. Once the results show that the method is in principle compatible to the tested biocatalyst, it is advisable to change the method of preparing the LentiKats® to keep identical conditions for different experiments. The mechanical properties of the produced LentiKats® and the survival rates of entrapped microorganisms strongly depend on the time of gelation and the uniformity in size of the droplets. Using a syringe creates droplets differing in size and, even more crucial, this successive approach leads to unequally distributed times of gelation. Moreover the amount of particles which can be manually produced is very limited and even for lab-scale applications it is a tedious work.
As an improvement a new device was developed to produce more than 400 identical droplets simultaneously in one step. Based on the well-known principle of printing technology a special printer head was designed for multiple transfer of equal amounts of PVA solution to a special surface (figure 8). Handling the printer is a cyclic sequence of the following steps: Loading each tip with the same amount of polymer solution by
303
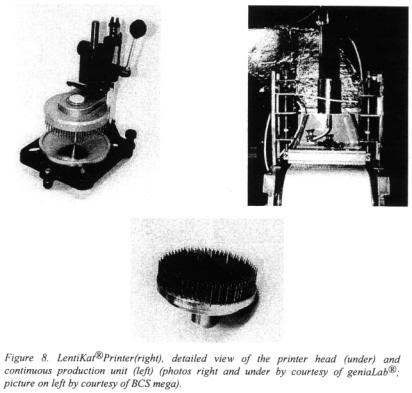
Ulrich Jahnz, Peter Wittlich, Ulf Prüsse and Klaus-Dieter Vorlop
dripping into, replacing the stock of polymer solution with a standard 145-mm-petri- dish, lowering the printer head and flooring the droplets on the petri-dish and finally exposing the fresh droplets to gelation conditions.
After appropriate time gelation is abruptly terminated by flooding with a stabilising solution. The described procedure guarantees that all particles are generated in the same way and no delays occur. This is especially important when the data of consecutively run experiments have to be compared and differences caused by the immobilisation process itself have to be precluded. Up to approx. 100 grams of identically immobilised material can be prepared in acceptable time for lab-scale applications under sterile or non-sterile conditions.
For the production of technical and industrial amounts of LentiKats®, a conveyor belt system was designed and built. Here a set of parallel nozzles drip the solution onto a continuously moving belt which runs in a drying chamber. After passing through the chamber the ready LentiKats® are scraped off and can be employed. The current machine produces technical amounts but can easily be scaled up since the principle of dripping, drying and scraping off is well established in various parts of the food industry and appropriate machinery is available for all scales.
304
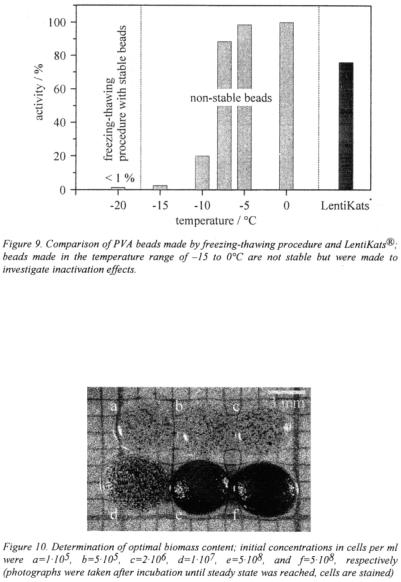
New matrices and bioencapsulation processes
4.3. EXAMPLES FOR APPLICATIONS OF LENTIKATS®
The benefiting influence of avoiding extreme temperature conditions in contrast to the cryogelated PVA-hydrogels was shown by immobilising a mixed culture of sensitive nitrifying bacteria (Nitrosomonas europaea and Nitrobacter winogradskyi). The initial activity of biomass could be raised from below 1 % (entrapped by freezing-thawing method, -20°C) to 75 % for immobilisation in LentiKats® compared to suspended cells of nitrifiers.
As another example of successful immobilisation in LentiKats®, the anaerobic bacterium Clostridium butyricum producing 1,3-propanediol from glycerol was encapsulated. The optimal initial biomass concentration was 2·107 cells/ml regarding the final hydrogel. LentiKats® with this concentration showed the highest activity.
305
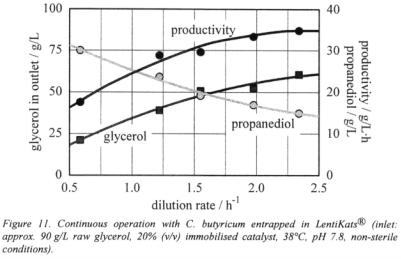
Ulrich Jahnz, Peter Wittlich, Ulf Prüsse and Klaus-Dieter Vorlop
After 10 weeks continuous operation under non-sterile conditions in a stirred 0.5-L- fermenter the LentiKats® showed no breakup. A productivity of 35 g/(lh) propanediol from raw glycerol was reached. This is the highest productivity known for this process so far (Wittlich et al., 2000).
5. Conclusions
In this paper we presented two techniques, both suitable for the encapsulation of biological catalysts. The JetCutting method produces monodisperse beads from highly viscous fluids. Due to its enormous production capacity it is applicable not only for laband technical scale but is a new device for industrial production of beads.
LentiKats® provide a new matrix for immobilisation, which has extraordinary properties and allows encapsulation at low costs. Due to mild conditions, e.g. no harsh chemicals and the use of room-temperature for gelation, even sensitive organisms show high rates of survival as was shown with nitrifying bacteria. The improved shape of LentiKats® reduces diffusion limitations and leads to very high values for specific activity and productivity.
References
Berger, R., Rühlemann, I. (1988) Stable ionotrophic gel for cell immobilisation using high molecular weight pectic acid, Ada Biotechnol., 8, 401-405
Brandenberger, H., Widmer, F. (1997) A new multinozzle encapsulation/immobilisation system, in Godia,
F., Poncelet, D. (eds.) Proceedings of the International Workshop Bioencapsulation VI, Barcelona, poster 9.
306
New matrices and bioencapsulation processes
Chibata, I., Tosa, T., Sato, T., Takata, I. (1987) Immobilisation of cells in carrageenan, Methods Enzymol., 135, 189-198.
Cougghlan, M.P., Kierstan M.P.J. (1987) Preparation and applications of immobilised microorganisms: a survey of recent reports, J. Microbiol. Methods, 8, 51-90.
Emori, H., Mikawa, K., Hamaya, M., Yamaguche, T., Tanaka, K., Takeshima, T. (1996) PEGASUS Innovative biological nitrogen removal process using entrapped nitrifiers, in Wijffels, R.H., Buitelaar, R.M., Bucke, C. Tramper, J. (eds.) Immobilised Cells: Basics and Applications, Elsevier Sciences B.V.
Kennedy, J.F., Melo, E.H.M (1990) Immobilised enzymes and cells, Chem. Eng. Prog., 86, 81-89.
Kierstan, M., Bucke, C. (1977) The immobilisation of microbial cells, subcellular organelles, and enzymes in calcium alginate gels, Biotechnol Bioeng 19 (3), 387-97.
Leenen, E.J.T.M., Dos Santos, V.A.P.M., Tramper J., Wijffels, R.H. (1996) Characteristics and selection criteria of support materials for immobilisation of nitrifying bacteria, in Wijffels et al. (eds.)
Immobilised CellsBasics and Applications, Elsevier Sciences B.V., 205-212.
Lozinsky, V.I. (1998) Cryotropic gelation of poly(vinyl alcohol), Russian Chemical Reviews, English
Edition.,67-7, 573-586.
Lozinsky, V.I., Plieva, F.M. (1998) Poly(vinyl alcohol) cryogels employed as matrices for cell immobilisation. D. Overview of recent research and developments, Enzyme Microbiol Technol., 23, 227242.
Muscat, A., Prüße., U., Vorlop, K.-D. (1996) Stable support materials for the immobilisation of viable cells, in Wijffels, R.H., Buitelaar, R.M., Bucke, C. Tramper, J. (eds.) Immobilised Cells: Basics and Applications, Elsevier Sciences B.V., 55-61.
Prüße, U., Bruske, F., Breford, J. and Vorlop K.-D. (1998). Improvement of the Jet Cutting method for the production of spherical particles from viscous polymer solutions, Chem. Eng Technol. 21, 153-157.
Smidsrød, V., Skjåk-Bræk, G. (1990) Alginate as immobilisation matrix for cells, Trends Biotechnol., 8, 71-
78.
Tanaka, K., Sumino, T., Nakamura, H., Ogasawara, T. and Emori, H. (1996) Application of nitrification by cells immobilised in polyethylene glycol, in Wijffels, R.H. et al. (eds.) Immobilised Cells Basics and
Applications, Elsevier Sciences, 622-632.
Vorlop, K.-D., Klein, J. (1983) New developments in the field of cell immobilisation: formation of biocatalysts by ionotrophic gelation, in Lafferty, R.M. (editor) Enzyme Technology, Springer Berlin, 219-235.
Vorlop, K.-D., Breford, J. (1994). German Patent DE 4424998.
Vorlop, K.-D., Klein, J. (1985) Immobilisation techniques, in Moo-Young (editor) Cells in Comprehensive Biotechnology 2, Pergamon Press, 203-224.
Vorlop, K.-D., Klein, J. (1987) Entrapment of microbial cells in chitosan, Methods Enzymol., 135, 259-268. Wittlich, P., Schlieker, M., Willke, T., Vorlop, K.-D. (2000) Leistungssteigerung biotechnischer Prozesse
durch neuartige Immobilisierungsmethoden für Biokatalysatoren am Beispiel der 1,3-Propandiol-
307
PART VII
DOWNSTREAM PROCESSING
INDUSTRIAL DOWNSTREAM PROCESSING
MADS LAUSTSEN
Novo Nordisk A/S
1. Introduction.
Industrial downstream challenges and solutions are highly dependent on the application of the product in question. The downstream processing field does cover both high price and high purity products like pharmaceuticals and fine chemicals, as it covers low cost production of bulk proteins like detergent enzymes.
To present a clear picture of the state of the art within industrial downstream processing, in which all relevant techniques and problem solutions have been discussed is therefore not an easy task. Further, where focus in public research is publication of ideas and results, knowledge and developed techniques in the industries will normally be held confidential where possible.
Indeed industry is often quite reluctant to disclose details regarding production processes and strategies as in many cases small differences in production cost or in development speed can make the difference between success or failure.
A drawback of these confidentiality issues is at times that alignment of public research with industrial needs is difficult.
This paper discusses some of the more important aspects of current downstream processing in industrial scale and future challenges for the industries. As a special focus some of the main differences between pharmaceutical production and bulk enzyme production are highlighted.
2. General aspects connected to downstream processing.
2.1. INTELLECTUAL PROPERTY RIGHTS.
Patent filing has for years been used extensively for protecting products, production organisms, potential protein engineering sites together with cloning and expression tools. Industrial fermentation and purification processes have previously been less protected as processes and developed techniques have been held confidential. However, in the later years a larger number of patent applications have also been filed within the
311
M. Hofman and P. Thonart (eds.), Engineering and Manufacturing for Biotechnology, 311–324.
© 2001 Kluwer Academic Publishers. Printed in the Netherlands.
Mads Laustsen
pharmaceutical downstream field. Also patent filing related to bulk enzyme production has started increasing. A major reason for this is the importance of freedom of operation, as even production processes that could have been running for years potentially can be hit by an issued patent. Infringement of third parts valid patent is something that can be very problematic. For pharmaceuticals it is indeed a must that the process developed is not violating patent rights as such processes are very troublesome to change. For enzyme production customers often want guaranty that the enzyme product and the process it has been produced by do not violate any third parts patent rights.
Patent filing within fermentation and recovery/purification processes is therefore very much done for safe guarding current and future freedom of operation.
As patent filing has been strengthened within the field, one can obtain information about industrial downstream processes and focus by looking into new patent applications from the industries.
2.2. PUBLIC RESEARCH IN DOWNSTREAM PROCESSING.
Research in the downstream field possesses some extra difficulties for universities and other public research centres as relevant research often needs to be performed with industrial relevant process streams - fermentation broth or partially purified product. Such process streams, or even information about them, will often not be handed out from companies. Further, for especially primary separation trials broth needs to be fresh
why |
trials have to be performed close to the production site. Because of these problems |
it is |
often easier for many companies to do research and development for process |
streams of which large quantities of production relevant product is needed, like for primary separation and crystallisation trials.
Much of the public research is and has been dedicated to chromatographic purification - the low volume, high purity area with fine chemical and pharmaceutical purification as the target. There are several good reasons for that. It is obviously of significant importance to the public health that industries are capable of producing safe and pure pharmaceuticals. With increased sensibility of quality analysis, increasing purity demands have followed, these setting higher demands to purification processes. Further, as industrially relevant chromatography trials can be performed in bench scale with a minimum of process liquid requirement, this research is relatively easy to perform for public institutes.
2.3. QUALITY.
Quality issues play a central role in process development, as it does in the final production processes. Where in the health care field quality is controlled through pharmaceutical GMP with strict demands to process validation and process documentation, the enzyme field has other less strict control systems. Production of food and feed enzymes has to comply with Food GMP regulations. Here with a clear focus on ensuring sanitary processing. Food enzyme production under Kosher
312