
- •Preface
- •1 A Voyage of Discovery
- •1.2 Goals
- •1.3 Organization
- •1.4 The Big Picture
- •1.5 Further Reading
- •2 The Historical Setting
- •2.2 Eras of Oceanographic Exploration
- •2.3 Milestones in the Understanding of the Ocean
- •2.4 Evolution of some Theoretical Ideas
- •2.5 The Role of Observations in Oceanography
- •2.6 Important Concepts
- •3 The Physical Setting
- •3.1 Ocean and Seas
- •3.2 Dimensions of the ocean
- •3.3 Sea-Floor Features
- •3.4 Measuring the Depth of the Ocean
- •3.5 Sea Floor Charts and Data Sets
- •3.6 Sound in the Ocean
- •3.7 Important Concepts
- •4.1 The Earth in Space
- •4.2 Atmospheric Wind Systems
- •4.3 The Planetary Boundary Layer
- •4.4 Measurement of Wind
- •4.5 Calculations of Wind
- •4.6 Wind Stress
- •4.7 Important Concepts
- •5 The Oceanic Heat Budget
- •5.1 The Oceanic Heat Budget
- •5.2 Heat-Budget Terms
- •5.3 Direct Calculation of Fluxes
- •5.4 Indirect Calculation of Fluxes: Bulk Formulas
- •5.5 Global Data Sets for Fluxes
- •5.6 Geographic Distribution of Terms
- •5.7 Meridional Heat Transport
- •5.8 Variations in Solar Constant
- •5.9 Important Concepts
- •6.2 Definition of Temperature
- •6.4 The Oceanic Mixed Layer and Thermocline
- •6.5 Density
- •6.6 Measurement of Temperature
- •6.7 Measurement of Conductivity or Salinity
- •6.8 Measurement of Pressure
- •6.10 Light in the Ocean and Absorption of Light
- •6.11 Important Concepts
- •7.1 Dominant Forces for Ocean Dynamics
- •7.2 Coordinate System
- •7.3 Types of Flow in the ocean
- •7.4 Conservation of Mass and Salt
- •7.5 The Total Derivative (D/Dt)
- •7.6 Momentum Equation
- •7.7 Conservation of Mass: The Continuity Equation
- •7.8 Solutions to the Equations of Motion
- •7.9 Important Concepts
- •8.2 Turbulence
- •8.3 Calculation of Reynolds Stress:
- •8.4 Mixing in the Ocean
- •8.5 Stability
- •8.6 Important Concepts
- •9 Response of the Upper Ocean to Winds
- •9.1 Inertial Motion
- •9.2 Ekman Layer at the Sea Surface
- •9.3 Ekman Mass Transport
- •9.4 Application of Ekman Theory
- •9.5 Langmuir Circulation
- •9.6 Important Concepts
- •10 Geostrophic Currents
- •10.1 Hydrostatic Equilibrium
- •10.2 Geostrophic Equations
- •10.3 Surface Geostrophic Currents From Altimetry
- •10.4 Geostrophic Currents From Hydrography
- •10.5 An Example Using Hydrographic Data
- •10.6 Comments on Geostrophic Currents
- •10.7 Currents From Hydrographic Sections
- •10.8 Lagrangian Measurements of Currents
- •10.9 Eulerian Measurements
- •10.10 Important Concepts
- •11.2 Western Boundary Currents
- •11.4 Observed Surface Circulation in the Atlantic
- •11.5 Important Concepts
- •12 Vorticity in the Ocean
- •12.2 Conservation of Vorticity
- •12.4 Vorticity and Ekman Pumping
- •12.5 Important Concepts
- •13.2 Importance of the Deep Circulation
- •13.3 Theory for the Deep Circulation
- •13.4 Observations of the Deep Circulation
- •13.5 Antarctic Circumpolar Current
- •13.6 Important Concepts
- •14 Equatorial Processes
- •14.1 Equatorial Processes
- •14.6 Important Concepts
- •15 Numerical Models
- •15.2 Numerical Models in Oceanography
- •15.3 Global Ocean Models
- •15.4 Coastal Models
- •15.5 Assimilation Models
- •15.6 Coupled Ocean and Atmosphere Models
- •15.7 Important Concepts
- •16 Ocean Waves
- •16.1 Linear Theory of Ocean Surface Waves
- •16.2 Nonlinear waves
- •16.3 Waves and the Concept of a Wave Spectrum
- •16.5 Wave Forecasting
- •16.6 Measurement of Waves
- •16.7 Important Concepts
- •17 Coastal Processes and Tides
- •17.1 Shoaling Waves and Coastal Processes
- •17.2 Tsunamis
- •17.3 Storm Surges
- •17.4 Theory of Ocean Tides
- •17.5 Tidal Prediction
- •17.6 Important Concepts
- •References
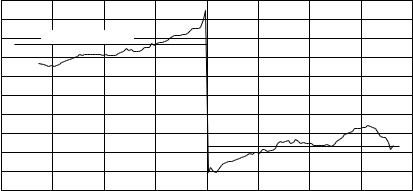
9.3. EKMAN MASS TRANSPORT |
143 |
|
80 |
|
|
|
|
|
|
|
|
(degrees) |
60 |
Ralph & Niiler (2000) |
|
|
|
|
|
|
|
40 |
|
|
|
|
|
|
|
|
|
20 |
New Data |
|
|
|
|
|
|
||
|
|
|
|
|
|
|
|
||
Wind |
|
|
|
|
|
|
|
|
|
0 |
|
|
|
|
|
|
|
|
|
the |
–20 |
|
|
|
|
|
|
|
|
to |
|
|
|
|
|
|
|
|
|
|
|
|
|
|
|
|
|
|
|
Angle |
–40 |
|
|
|
|
|
|
|
|
–60 |
|
|
|
|
|
|
|
|
|
|
|
|
|
|
|
|
|
|
|
|
–80 |
|
|
|
|
|
|
|
|
|
–80 |
–60 |
–40 |
–20 |
0 |
20 |
40 |
60 |
80 |
Latitude (degrees)
Figure 9.6 Angle between the wind and flow at the surface calculated by Maximenko and Niiler using positions from drifters drogued at 15 m with satellite-altimeter, gravity, and grace data and winds from the ncar/ncep reanalysis.
falls below the critical value (Pollard et al. 1973). This idea, when included in p
mixed-layer theories, leads to a surface current V0 that is proportional to N/f
|
V0 U10p |
|
|
|
|
|
|
N/f |
(9.22) |
||||
where N is the stability frequency defined by (8.36). Furthermore |
|
|||||
Az U102 /N |
and DE U10/p |
|
|
|
||
N f |
(9.23) |
Notice that (9.22) and (9.23) are now dimensionally correct. The equations used earlier, (9.14), (9.16), (9.20), and (9.21) all required a dimensional coe cient.
9.3Ekman Mass Transport
Flow in the Ekman layer at the sea surface carries mass. For many reasons we may want to know the total mass transported in the layer. The Ekman mass transport ME is defined as the integral of the Ekman velocity UE , VE from the surface to a depth d below the Ekman layer. The two components of the transport are MEx, MEy :
0 |
0 |
|
MEx = Z−d ρUE dz, |
MEy = Z−d ρVE dz |
(9.24) |
The transport has units kg/(m·s). It is the mass of water passing through a vertical plane one meter wide that is perpendicular to the transport and extending from the surface to depth −d (figure 9.7).
We calculate the Ekman mass transports by integrating (8.15) in (9.24):
f Z−d |
ρ VE dz = f MEy = − Z−d dTxz |
|
|
0 |
0 |
|
|
|
f MEy = −Txz z=0 + Txz |
z=−d |
(9.25) |
|
|
|
|
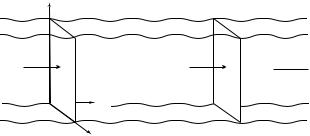
144 CHAPTER 9. RESPONSE OF THE UPPER OCEAN TO WINDS
z |
|
|
} 1m |
|
} Y |
U |
U |
Q = |
|
|
X |
x |
|
|
y |
|
|
Sea Surface
Y M X
r
Figure 9.7 Sketch for defining Left: mass transports, and Right: volume transports.
A few hundred meters below the surface the Ekman velocities approach zero, and the last term of (9.25) is zero. Thus mass transport is due only to wind stress at the sea surface (z = 0). In a similar way, we can calculate the transport in the x direction to obtain the two components of the Ekman transport :
f MEy = −Txz(0) |
(9.26a) |
f MEx = Tyz(0) |
(9.26b) |
where Txz(0), Tyz (0) are the two components of the stress at the sea surface. Notice that the transport is perpendicular to the wind stress, and to the right
of the wind in the northern hemisphere. If the wind is to the north in the positive y direction (a south wind), then Txz(0) = 0, MEy = 0, and MEx = Tyz (0)/f . In the northern hemisphere, f is positive, and the mass transport is in the x direction, to the east.
It may seem strange that the drag of the wind on the water leads to a current at right angles to the drag. The result follows from the assumption that friction is confined to a thin surface boundary layer, that it is zero in the interior of the ocean, and that the current is in equilibrium with the wind so that it is no longer accelerating.
Volume transport Q is the mass transport divided by the density of water and multiplied by the width perpendicular to the transport.
Qx = |
Y Mx |
, |
Qy = |
XMy |
(9.27) |
|
ρ |
ρ |
|||||
|
|
|
|
where Y is the north-south distance across which the eastward transport Qx is calculated, and X in the east-west distance across which the northward transport Qy is calculated. Volume transport has dimensions of cubic meters per second. A convenient unit for volume transport in the ocean is a million cubic meters per second. This unit is called a Sverdrup, and it is abbreviated Sv.
Recent observations of Ekman transport in the ocean agree with the theoretical values (9.26). Chereskin and Roemmich (1991) measured the Ekman volume transport across 11◦N in the Atlantic using an acoustic Doppler current profiler described in Chapter 10. They calculated a transport of Qy = 12.0 ±5.5 Sv (northward) from direct measurements of current, Qy = 8.8 ± 1.9 Sv from
9.4. APPLICATION OF EKMAN THEORY |
145 |
measured winds using (9.26) and (9.27), and Qy = 13.5 ± 0.3 Sv from mean winds averaged over many years at 11◦N.
Use of Transports Mass transports are widely used for two important reasons. First, the calculation is much more robust than calculations of velocities in the Ekman layer. By robust, I mean that the calculation is based on fewer assumptions, and that the results are more likely to be correct. Thus the calculated mass transport does not depend on knowing the distribution of velocity in the Ekman layer or the eddy viscosity.
Second, the variability of transport in space has important consequences. Let’s look at a few applications.
9.4Application of Ekman Theory
Because steady winds blowing on the sea surface produce an Ekman layer that transports water at right angles to the wind direction, any spatial variability of the wind, or winds blowing along some coasts, can lead to upwelling. And upwelling is important:
1.Upwelling enhances biological productivity, which feeds fisheries.
2.Cold upwelled water alters local weather. Weather onshore of regions of upwelling tend to have fog, low stratus clouds, a stable stratified atmosphere, little convection, and little rain.
3.Spatial variability of transports in the open ocean leads to upwelling and downwelling, which leads to redistribution of mass in the ocean, which leads to wind-driven geostrophic currents via Ekman pumping.
Coastal Upwelling To see how winds lead to upwelling, consider north winds blowing parallel to the California Coast (figure 9.8 left). The winds produce a mass transport away from the shore everywhere along the shore. The water pushed o shore can be replaced only by water from below the Ekman layer. This is upwelling (figure 9.8 right). Because the upwelled water is cold, the upwelling leads to a region of cold water at the surface along the coast. Figure 10.16 shows the distribution of cold water o the coast of California.
Upwelled water is colder than water normally found on the surface, and it is richer in nutrients. The nutrients fertilize phytoplankton in the mixed layer, which are eaten by zooplankton, which are eaten by small fish, which are eaten by larger fish and so on to infinity. As a result, upwelling regions are productive waters supporting the world’s major fisheries. The important regions are o shore of Peru, California, Somalia, Morocco, and Namibia.
Now I can answer the question I asked at the beginning of the chapter: Why is the climate of San Francisco so di erent from that of Norfolk, Virginia? Figures 4.2 or 9.8 show that wind along the California and Oregon coasts has a strong southward component. The wind causes upwelling along the coast, which leads to cold water close to shore. The shoreward component of the wind brings warmer air from far o shore over the colder water, which cools the incoming air close to the sea, leading to a thin, cool atmospheric boundary layer. As the air cools, fog forms along the coast. Finally, the cool layer of air is blown over

146 CHAPTER 9. RESPONSE OF THE UPPER OCEAN TO WINDS
Ekman |
Wind |
|
|
Transport |
|
|
|
|
|
|
|
ME |
|
|
U |
|
|
d |
|
|
Col |
|
|
|
|
|
|
|
ed |
Water |
|
l |
|
|
pwe |
l |
|
|
|
|
|
Land (California)
ME
100 - 300 m
|
|
|
r |
|
|
te |
|
|
a |
|
|
g |
W |
|
|
Upwellin |
|
|
|
100 km
Figure 9.8 Sketch of Ekman transport along a coast leading to upwelling of cold water along the coast. Left: Plan view. North winds along a west coast in the northern hemisphere cause Ekman transports away from the shore. Right: Cross section. The water transported o shore must be replaced by water upwelling from below the mixed layer.
San Francisco, cooling the city. The warmer air above the boundary layer, due to downward velocity of the Hadley circulation in the atmosphere (see figure 4.3), inhibits vertical convection, and rain is rare. Rain forms only when winter storms coming ashore bring strong convection higher up in the atmosphere.
In addition to upwelling, other processes influence weather in California and Virginia.
1.The oceanic mixed layer tends to be thin on the eastern side of ocean, and upwelling can easily bring up cold water.
2.Currents along the eastern side of the ocean at mid-latitudes tend to bring colder water from higher latitudes.
All these processes are reversed o shore of east coasts, leading to warm water close to shore, thick atmospheric boundary layers, and frequent convective rain. Thus Norfolk is much di erent that San Francisco due to upwelling and the direction of the coastal currents.
Ekman Pumping The horizontal variability of the wind blowing on the sea surface leads to horizontal variability of the Ekman transports. Because mass must be conserved, the spatial variability of the transports must lead to vertical velocities at the top of the Ekman layer. To calculate this velocity, we first integrate the continuity equation (7.19) in the vertical:
ρ Z−d |
∂x + |
∂y + ∂z dz = 0 |
|||||||||||
|
0 |
∂u |
∂v |
|
∂w |
|
|
|
|||||
∂x |
Z−d ρ u dz + |
|
∂y |
Z−d |
ρ v dz = −ρ Z−d ∂z dz |
||||||||
∂ |
0 |
|
|
|
∂ |
|
0 |
|
|
0 ∂w |
|||
|
|
|
|
|
|
|
|
∂MEy |
|
|
|
||
|
|
|
∂MEx |
+ |
= −ρ [w(0) − w(−d)] |
||||||||
|
|
|
∂x |
|
∂y |
By definition, the Ekman velocities approach zero at the base of the Ekman layer, and the vertical velocity at the base of the layer wE (−d) due to divergence