
Environmental Biotechnology - Jordening and Winter
.pdf
252 8 Principles of Anaerobic Degradation of Organic Compounds
Chien, C.-C., Leadbetter, E. R., Godchaux III, W., Sulfonate sulfur can be assimilated for fermentative growth, FEMS Microbiol. Lett. 1995, 129, 189–194.
Coates, J. D., Chakraborty, R., Lack, J. G., O’Connor, S. M., Cole, K. A., Bender, K. S., Achenbach, L. A., Anaerobic benzene oxidation coupled to nitrate reduction in pure culture by two strains of Dechloromonas, Nature 2001, 411, 1039–1043.
Cook, A. M., Laue, H., Junker, F., Microbial desulfonation, FEMS Microbiol. Rev. 1999,
22, 399–419.
Dagley, S., Catabolism of aromatic compounds by microorganisms, Adv. Microb. Physiol. 1971, 6, 1–46.
Dolfing, J., Zeyer, P., Binder-Eicher, P., Schwarzenbach, R. P., Isolation and characterization of a bacterium that mineralizes toluene in the absence of molecular oxygen,
Arch. Microbiol. 1990, 154, 336–341. Edwards, E. A., Grbic´-Galic´, D., Anaerobic
degradation of toluene and o-xylene by a methanogenic consortium, Appl. Environ. Microbiol. 1994, 60, 313–322.
Egli, T., Bally, M., Uetz, T., Microbial degradation of chelating agents used in detergents with special reference to nitrilotriacetic acid (NTA), Biodegradation 1990, 1, 121–132.
El Fantroussi, S., Naveau, H., Agathos, S. N., Anaerobic dechlorinating bacteria, Biotech- nol. Prog. 1998, 14, 167–188.
Engelmann, T., Kaufmann, F., Diekert, G., Isolation and characterization of a veratrol:corrinoid protein methyl transferase from Acetobacterium dehalogenans, Arch. Microbiol. 2001, 175, 376–383.
Elsner, M., Schwarzenbach, R. P., Haderlein, S. B., Reactivity of Fe(II)-bearing minerals toward reductive transformation of organic contaminants, Environ. Sci. Technol. 2004,
38, 799–807.
Evans, P. J., Mang, D. T., Kim, K. S., Young, L. Y., Anaerobic degradation of toluene by a denitrifying bacterium, Appl. Environ. Microbiol. 1991, 57, 1139–1145.
Evans, W. C., Biochemistry of the bacterial catabolism of aromatic compounds in anaerobic environments, Nature 1977, 270, 17–22.
Evans, W. C., Fuchs, G., Anaerobic degradation of aromatic compounds, Annu. Rev. Microbiol. 1988, 42, 289–317.
Fetzner, S., Lingens, F., Bacterial dehalogenases: biochemistry, genetics, and biotechnological applications, Microbiol. Rev. 1994, 58, 641– 685.
Foß, S., Harder, J., Thauera linaloolentis sp. nov. and Thauera terpenica sp. nov., isolated on oxygen-containing monoterpenes (linalool, menthol, and eucalyptol) and nitrate,
Syst. Appl. Microbiol. 1998, 21, 365–373. Foß, S., Heyen, U., Harder, J., Alcaligenes de-
fragrans sp. nov., description of four strains isolated on alkenoic monoterpenes ((c)-men- thene, α-pinene, 2-carene and α-phellan- drene) and nitrate, Syst. Appl. Microbiol. 1998,
21, 237–244.
Frings, J., Schramm, E., Schink, B., Enzymes involved in anaerobic polyethylene glycol degradation by Pelobacter venetianus and Bac- teroides strain PG1, Appl. Environ. Microbiol.
1992, 58, 2164– 2167.
Fuchs, G., El-Said, M., M., Altenschmidt, U., Koch, J., Lack, A. et al., Biochemistry of anaerobic biodegradation of aromatic compounds, in: Biochemistry of Microbial Degradation (Ratledge, C., ed.), pp. 513–553. Dordrecht 1994: Kluwer.
Gallert, C., Knoll, G., Winter, J., Anaerobic carboxylation of phenol to benzoate: use of deuterated phenols revealed carboxylation exclusively in the C4 position, Appl. Microbiol. Biotechnol. 1991, 36, 124–129.
Galushko, A., Minz, D., Schink, B., Widdel, F., Anaerobic degradation of naphthalene by a pure culture of a novel type of marine sul- fate-reducing bacterium, Environ. Microbiol. 1999, 1, 415– 420.
Gorny, N., Schink, B., Hydroquinone degradation via reductive dehydroxylation of gentisylCoA by a strictly anaerobic fermenting bacterium, Arch. Microbiol. 1994a, 161, 25–32.
Gorny, N., Schink, B., Complete anaerobic oxidation of hydroquinone by Desulfococcus sp. strain Hy5: indications of hydroquinone carboxylation to gentisate, Arch. Microbiol. 1994b, 162, 131–135.
Gorny, N., Schink, B., Anaerobic degradation of catechol by Desulfobacterium sp. strain Cat2 proceeds via carboxylation to protocatechuate, Appl. Environ. Microbiol. 1994c, 60, 3396–3340.
Gorny, N., Wahl, G., Brune, A., Schink, B., A strictly anaerobic nitrate-reducing bacterium growing with resorcinol and other aromatic compounds, Arch. Microbiol. 1992, 158, 48–53.

Haddock, J. D., Ferry, J. G., Purification and properties of phloroglucinol reductase from
Eubacterium oxidoreducens G-41, J. Biol. Chem. 1989, 264, 4423–4427.
Harder, J., Anaerobic methane oxidation by bacteria employing 14C-methane uncontaminated with 14C-carbon monoxide, Mar. Geol. 1997, 137, 13–23.
Harder, M., Probian, C., Microbial degradation of monoterpenes in the absence of molecular oxygen, Appl. Environ. Microbiol.
1995, 61, 3804–3808.
Harms, G., Zengler, K., Rabus, R., Aeckersberg, F., Minz, D. et al., Anaerobic oxidation of o-xylene, m-xylene, and homologous alkyl benzenes by new types of sulfate-re- ducing bacteria, Appl. Environ. Microbiol.
1999, 65, 999–1004.
Harwood, C. S., Burchhardt, G., Herrmann, H., Fuchs, G., Anaerobic metabolism of aromatic compounds via the benzoyl-CoA pathway, FEMS Microbiol. Rev. 1999, 22, 439–458.
Heider, J., Fuchs, G., Anaerobic metabolism of aromatic compounds, Eur. J. Biochem. 1997, 243, 577–596.
Heider, J., Spormann, A. M., Beller, H. R., Widdel, F., Anaerobic bacterial metabolism of hydrocarbons, FEMS Microbiol. Rev.
1999, 22, 459–473.
Heiss, G., Knackmuss, H. J., Bioelimination of trinitroaromatic compounds: immobilization versus mineralization, Curr. Opin. Microbiol. 2002, 5, 282–287.
Hippe, H., Caspari, D., Fiebig, K., Gottschalk, G., Utilization of trimethylamine and other N-methyl compounds for growth and methane formation by Methanosarcina barkeri, Proc. Natl. Acad. Sci. USA 1979, 76, 494–498.
Holliger, C., Wohlfarth, G., Diekert, G., Reductive dechlorination in the energy metabolism of anaerobic bacteria, FEMS Microbiol. Rev. 1999, 22, 383–398.
Hopper, D. J., Incorporation of [18O]water in the formation of p-hydroxybenzyl alcohol by the p-cresol methylhydroxylase from Pseu- domonas putida, Biochem. J. 1978, 175, 345–347.
Hopper, D. J., Bossert, I. D., Rhodes-Roberts, M. E., p-Cresol methylhydroxylase from a denitrifying bacterium involved in anaerobic degradation of p-cresol, J. Bacteriol. 1991, 173, 1298–1301.
References 253
Hutzinger, O., The Handbook of Environmental Chemistry. Berlin 1982: Springer-Verlag.
Hylemon, P. B., Harder, J., Biotransformation of monoterpenes, bile acids, and other isoprenoids in anaerobic ecosystems, FEMS Microbiol. Rev. 1999, 22, 475–488.
Janssen, P. H., Schink, B., 14CO2 exchange with acetoacetate catalyzed by dialyzed cellfree extracts of the bacterial strain BunN grown with acetone and nitrate, Eur. J. Biochem. 1995, 228, 677– 682.
Johnson, H. A., Spormann, A. M., In vitro studies on the initial reactions of anaerobic ethylbenzene mineralization, J. Bacteriol. 1999, 181, 5662–5668.
Johnson, H. A., Pelletier, D. A., Spormann, A. M., Isolation and characterization of anaerobic ethylbenzene dehydrogenase, a novel Mo- Fe-S enzyme, J. Bacteriol. 2001, 183, 4536–4542.
Kaufmann, F., Wohlfahrt, G., Diekert, G., Isolation of O-demethylase, an ether-cleaving enzyme system of the homoacetogenic strain MC, Arch. Microbiol. 1997, 168, 136–142.
Kaufmann, F., Wohlfarth, G., Diekert, G., O- demethylase from Acetobacterium dehalogenans: cloning, sequencing, and active expression of the gene encoding the corrinoid protein, Eur. J. Biochem. 1998, 257, 515–521.
Keltjens, J. T., Vogels, G. D., Conversion of methanol and methylamines to methane and carbon dioxide, in: Methanogenesis (Ferry, J. G., ed.), pp. 253–303. New York 1993: Chapman & Hall.
Kerem, Z., Bao, W., Hammel, K. E., Rapid polyether cleavage via extracellular one-elec- tron oxidation by a brown-rot basidiomycete,
Proc. Natl. Acad. Sci. USA 1998, 95, 10373–10377.
Kiene, R. P., Oremland, R. S., Catena, A., Miller, L. G., Capone, D. G., Metabolism of reduced methylated sulfur compounds in anaerobic sediments and by a pure culture of an estuarine methanogen, Appl. Environ. Microbiol. 1986, 52, 1037– 1045.
Kluge, C., Tschech, A., Fuchs, G., Anaerobic metabolism of resorcylic acids (m−dihydrox− ybenzoic acids) and resorcinol (1,3-benzened- iol) in a fermenting and in a denitrifying bacterium, Arch. Microbiol. 1990, 155, 68–74.
Koch, J., Eisenreich, W., Bacher, A., Fuchs, G., Products of enzymatic reduction of benzoylCoA, a key reaction in anaerobic aromatic metabolism, Eur. J. Biochem. 1993, 211, 649–661.

254 8 Principles of Anaerobic Degradation of Organic Compounds
Kondo, H., Ishimoto, M., Purification and properties of sulfoacetaldehyde sulfolyase, a thiamine pyrophosphate-dependent enzyme forming sulfite and acetate, J. Biochem. 1975, 78, 317–325.
Kreft, J.-U., Schink, B., Demethylation and further degradation of phenyl methylethers by the sulfide-methylating homoacetogenic bacterium strain TMBS4, Arch. Microbiol. 1993, 159, 308–315.
Kreft, J., Schink, B., O-Demethylation by the homoacetogenic anaerobe Holophaga foetida studied by a new photometric methylation assay using electrochemically produced cob(I)alamin, Eur. J. Biochem. 1994, 226, 945–951.
Krieger, C. J., Beller, H. R., Reinhard, M., Spormann, A. M., Initial reactions in anaerobic oxidation of m-xylene by the denitrifying bacterium Azoarcus sp. strain T, J. Bacteriol. 1999, 181, 6403–6410.
Krumholz, L. R., Bryant, M. P., Characterization of the pyrogallol–phloroglucinol isomerase of Eubacterium oxidoreducens, J. Bacteriol. 1988, 170, 2472–2479.
Lack, A., Fuchs, G., Carboxylation of phenylphosphate by phenol caboxylase, an enzyme system of anaerobic phenol metabolism in a denitrifying Pseudomonas sp., J. Bacteriol. 1992, 174, 3629–3636.
Lack, A., Fuchs, G., Evidence that phenol phosphorylation to phenylphosphate is the first step in anaerobic phenol metabolism in a denitrifying Pseudomonas sp., Arch. Microbiol. 1994, 161, 306–311.
Laue, H., Denger, K., Cook, A., Taurine reduction in anaerobic respiration of Bilophila wadsworthia RZA-TAU, Appl. Environ. Microbiol. 1997a) 63, 2016–2021.
Laue, H., Denger, K., Cook, A., Fermentation of cysteate by a sulfate-reducing bacterium,
Arch. Microbiol. 1997b) 168, 210–214. Lengeler, J. W., Drews, G., Schlegel, H. G.
(eds.), Biology of the Prokaryotes, Stuttgart
1999: Georg Thieme Verlag.
Lovley, D. R., Coates, J. D., Woodward, J. C., Phillips, E. J. P., Benzene oxidation coupled to sulfate reduction, Appl. Environ. Microbiol. 1995, 61, 953–958.
Lovley, D. R., Woodward, J. C., Chapelle, F. H., Rapid anaerobic benzene oxidation with a variety of chelated Fe(III) forms, Appl. Environ. Microbiol. 1996, 62, 288–291.
Lukins, H. B., Foster, J. W., Methylketone metabolism in hydrocarbon utilizing mycobacteria, J. Bacteriol. 1963, 85, 1074–1087.
Maillard, J., Schumacher, W., Vazquez, F., Regeard, C., Hagen, W. R., Holliger, C., Characterization of the corrinoid iron-sulfur protein tetrachloroethene reductive dehalogenase of
Dehalobacter restrictus, Appl. Environ. Microbiol. 2003, 69, 4628–4638.
Meckenstock, R. U., Krieger, R., Ensign, S., Kroneck, P. M. H., Schink, B., Acetylene hydratase of Pelobacter acetylenicus: molecular and spectroscopic properties of the tungsten iron-sulfur enzyme, Eur. J. Biochem. 1999, 264, 176–182.
Müller, J. A., Galushko, A. S., Kappler, A., Schink, B., Anaerobic degradation of m-cres- ol by Desulfobacterium cetonicum is initiated by formation of 3-hydroxybenzylsuccinate,
Arch. Microbiol. 1999, 172, 287–294.
Müller, J. A., Schink, B., Initial steps in the fermentation of 3-hydroxybenzoate by Sporoto- maculum hydroxybenzoicum, Arch. Microbiol.
2000, 173, 288–295.
Nauhaus, K., Boetius, A., Kruger, M., Widdel, F., In vitro demonstration of anaerobic oxidation of methane coupled to sulphate reduction in sediment from a marine gas hydrate area, Environ. Microbiol. 2002, 4, 296–305.
Naumova, R. P., Selivanovskaya, S. Y., Cherepneva, I. E., Conversion of 2,4,6-trinitroto- luene under conditions of oxygen and nitrate respiration of Pseudomonas fluorescens, Appl. Biochem. Microbiol. 1989, 24, 409–413.
Neumann, A., Siebert, A., Trescher, T., Reinhardt, S., Wohlfarth, G., Diekert, G., Tetrachloroethene reductive dehalogenase of De- halospirillum multivorans: substrate specificity of the native enzyme and its corrinoid cofactor, Arch. Microbiol. 2002, 177, 420–426.
Neumann, A., Wohlfarth, G., Diekert, G., Tetrachloroethene dehalogenase from Dehalospi- rillum multivorans: cloning, sequencing of the encoding genes, and expression of the pceA gene in Escherichia coli, J. Bacteriol. 1998, 180, 4140–4145.
Nörtemann, B., Total degradation of EDTA by mixed cultures and a bacterial isolate, Appl. Environ. Microbiol. 1992, 58, 671–676.
Oremland, R. S., Kiene, R. P., Mathrani, I., Whiticar, M. J., Boone, D. R., Description of an estuarine methylotrophic methanogen which grows on dimethylsulfide, Appl. Environ. Microbiol. 1989, 55, 994–1002.

Pecher, K., Haderlein, S. B., Schwarzenbach, R. P., Reduction of polyhalogenated methanes by surface-bound Fe(II) in aqueous suspensions of iron oxides, Environ. Sci. Technol. 2002) 36, 1734–1741.
Peters, F., Rother, M., Boll, M., Selenocys- teine-containing proteins in anaerobic benzoate metabolism of Desulfococcus multivorans, J. Bacteriol. 2004, 186, 2156–2163.
Philipp, B., Schink, B., Evidence of two oxidative reaction steps initiating anaerobic degradation of resorcinol (1,3-dihydroxyben- zene) by the denitrifying bacterium Azoar- cus anaerobius, J. Bacteriol. 1998, 180, 3644–3649.
Platen, H., Schink, B., Methanogenic degradation of acetone by an enrichment culture,
Arch. Microbiol. 1987, 149, 136–141.
Platen, H., Schink, B., Anaerobic degradation of acetone and higher ketones via carboxylation by newly isolated denitrizing bacteria, J. Gen. Microbiol. 1989, 135, 883–891.
Platen, H., Schink, B., Enzymes involved in anaerobic degradation of acetone by a denitrifying bacterium, Biodegradation 1991, 1, 243–251.
Platen, H., Temmes, A., Schink, B., Anaerobic degradation of acetone by Desulfococcus biacutus sp. nov., Arch. Microbiol. 1990, 154, 355–361.
Preuss, A., Fimpel, J., Diekert, G., Anaerobic transformation of 2,4,6-trinitrotoluene (TNT), Arch. Microbiol. 1993, 159, 345–355.
Rabus, R., Heider, J., Initial reactions of anaerobic metabolism of alkyl benzenes in denitrifying and sulfate-reducing bacteria,
Arch. Microbiol. 1998, 170, 337–384. Rabus, R., Widdel, F., Anaerobic degradation
of ethyl benzene and other aromatic hydrocarbons by new denitrifying bacteria, Arch. Microbiol. 1995, 163, 96–103.
Rabus, R., Nordhaus, R., Ludwig, W., Widdel, F., Complete oxidation of toluene under strictly anaerobic conditions by a new sulfate-reducing bacterium, Appl. Environ. Microbiol. 1993, 59, 1444–1451.
Rabus, R., Wilkes, H., Behrends, A., Armstroff, A., Fischer, T., Pierik, A. J., Widdel, F., Anaerobic initial reaction of n-alkanes in a denitrifying bacterium: evidence for (1-methylpentyl)succinate as initial product and for involvement of an organic radical in n−hexane metabolism, J. Bacteriol. 2001, 183, 1707–1715.
References 255
Reichenbecher, W., Brune, A., Schink, B., Transhydroxylase of Pelobacter acidigallici: a molybdoenzyme catalyzing the conversion of pyrogallol to phloroglucinol, Biochim. Biophys. Acta 1994, 1204, 217–224.
Reichenbecher, W., Rüdiger, A., Kroneck, P. M. H., Schink, B., One molecule of molybdopterin guanine dinucleotide is associated with each subunit of the heterodimeric Mo- Fe-S protein transhydroxylase of Pelobacter acidigallici as determined by SDS/PAGE and mass spectrometry, Eur. J. Biochem. 1996, 237, 406–413.
Rosner, B., Schink, B., Purification and characterization of acetylene hydratase of Pelobacter acetylenicus, a tungsten iron-sulfur protein, J. Bacteriol. 1995, 177, 5767–5772.
Rudolphi, A., Tschech, A. Fuchs, G., Anaerobic degradation of cresols by denitrifying bacteria, Arch. Microbiol. 1991, 155, 238–248.
Rueter, P., Rabus, R., Wilkes, H., Aeckersberg, F., Rainey, F. A. et al., Anaerobic oxidation of hydrocarbons in crude oil by denitrifying bacteria, Nature 1994, 372, 445–458.
Schink, B., Degradation of unsaturated hydrocarbons by methanogenic enrichment cultures, FEMS Microbiol. Ecol. 1985a, 31, 69–77.
Schink, B., Fermentation of acetylene by an obligate anaerobe, Pelobacter acetylenicus sp. nov., Arch. Microbiol. 1985b, 142, 295–301.
Schink, B., Principles and limits of anaerobic degradation: environmental and technological aspects, in: Biology of Anaerobic Microorganisms (Zehnder, A. J. B., ed.), pp. 771–846. New York 1988: Wiley.
Schink, B., Chances and limits of anaerobic degradation of organic compounds, in: Mik- robielle Eliminierung chlororganischer Verbindungen (Cuno, M., ed.), Schriftenreihe Biologische Abwasserreinigung Vol. 6, pp. 57–67. Berlin 1995: Technische Universität.
Schink, B., Energetics of syntrophic cooperations in methanogenic degradation, Microbi- ol. Mol. Biol. Rev. 1997, 61, 262–280.
Schink, B., Pfennig, N., Fermentation of trihydroxybenzenes by Pelobacter acidigallici gen. nov. sp. nov., a new strictly anaerobic nonspore forming bacterium, Arch. Microbiol. 1982, 133, 195–201.
Schink, B., Brune, A., Schnell, S., Anaerobic degradation of aromatic compounds, in: Mi- crobial Degradation of Natural Compounds
(Winkelmann, G., ed.), pp. 219–242. Weinheim 1992: VCH.

256 8 Principles of Anaerobic Degradation of Organic Compounds
Schink, B., Philipp, B., Müller, J., Anaerobic degradation of phenolic compounds. Natur- wissenschaften 2000, 87, 12–23.
Schnell, S., Schink, B., Anaerobic aniline degradation via reductive deamination of 4-aminobenzoyl CoA in Desulfobacterium anilini, Arch. Microbiol. 1991, 155, 183–190.
Schnell, S., Brune, A., Schink, B., Degradation of hydroxy hydroquinone by the strictly anaerobic fermenting bacterium Pelobacter massiliensis sp. nov., Arch. Microbiol. 1991,
155, 511–516.
Schocher, R. J., Seyfried, B., Vazquez, F., Zeyer, J., Anaerobic degradation of toluene by pure cultures of denitrifying bacteria,
Arch. Microbiol. 1991, 157, 7–12.
Schöcke, L., Schink, B., Biochemistry and energetics of fermentative benzoate degradation by Syntrophus gentianae, Arch. Microbiol. 1999, 171, 331–337.
Schramm, E., Schink, B., Ether-cleaving enzyme and diol dehydratase involved in anaerobic polyethylene glycol degradation by an Acetobacterium sp., Biodegradation 1991,
2, 71–79.
Schumacher, W., Holliger, C., Zehnder, A. J., Hagen, W. R., Redox chemistry of cobalamin and iron-sulfur cofactors in the tetrachloroethene reductase of Dehalobacter restrictus, FEBS Lett. 1997, 409, 421–425.
Seyfried, B., Glod, G., Schocher, R., Tschech, A., Zeyer, J., Anaerobic degradation of toluene by pure cultures of denitrifying bacteria, Appl. Environ. Microbiol. 1994, 60, 4047–4052.
Sluis, M. K., Ensign, S. A., Purification and characterization of acetone carboxylase in a CO2-dependent pathway of acetone metabolism by Xanthobacter strain Py2, Proc. Natl. Acad. Sci. USA 1997, 94, 8456–8461.
Sluis, M. K., Small, F. J., Allen, J. R., Ensign, S. A., Involvement of an ATP-dependent carboxylase in a CO2-dependent pathway of acetone metabolism by Xanthobacter strain Py2, J. Bacteriol. 1996, 178, 4020–4026.
Sluis, M. K., Larsen, R. A., Krum, J. G., Anderson, R., Metcalf, W. W., Ensign, S. A., Biochemical, molecular, and genetic analyses of the acetone carboxylases from Xan- thobacter autotrophicus strain Py2 and Rhod- obacter capsulatus strain B10, J. Bacteriol.
2002, 184, 2969–2977.
Spormann, A., M., Widdel, F., Metabolism of alkylbenzenes, alkanes, and other hydrocar-
bons in anaerobic bacteria, Biodegradation 2000, 11, 85–105.
Stupperich, E., Konle, R., Corrinoid-dependent methyl transfer reactions are involved in methanol and 3,4-dimethoxybenzoate metabolism by Sporomusa ovata, Appl. Environ. Microbiol. 1993, 59, 3110–3116.
Suflita, J. M., Horowitz, A., Shelton, D. R., Tiedje, J. M., Dehalogenation: a novel pathway for the anaerobic biodegradation of haloaromatic compounds, Science 1982, 218, 1115–1117.
Suflita, J. M., Liang, L.-N., Saxena, A., The anaerobic degradation of o-, m-, and p-cresol by sulfate-reducing bacterial enrichment cultures obtained from a shallow anoxic aquifer,
J. Ind. Microbiol. 1989, 4, 255–266.
Tarvin, D., Buswell, A. M., The methane fermentation of organic acids and carbohydrates, J. Am. Chem. Soc. 1934, 56, 1751–1755.
Taylor, D. G., Trudgill, P. W., Gripps, R. E., Harris, P. R., The microbial metabolism of acetone, J. Gen. Microbiol. 1980, 118, 159–170.
Thauer, R. K., Jungermann, K., Decker, K., Energy conservation in chemotrophic anaerobic bacteria, Bacteriol. Rev. 1977, 41, 100–180.
Tschech, A., Fuchs, G., Anaerobic degradation of phenol via carboxylation to 4-hydroxyben- zoate: in vitro study of isotope exchange between 14CO2 and 4-hydroxybenzoate, Arch. Microbiol. 1989, 152, 594–599.
Tschech, A., Schink, B., Fermentative degradation of resorcinol and resorcylic acids, Arch. Microbiol. 1985, 143, 52–59.
Verfurth, K., Pierik, A. J., Leutwein, C., Zorn, S., Heider, J., Substrate specificities and electron paramagnetic resonance properties of benzylsuccinate synthases in anaerobic toluene and m-xylene metabolism, Arch. Microbiol. 2004, 181, 155–162.
Vogel, T. M., Grbic´-Galic´, D., Incorporation of oxygen from water into toluene and benzene during anaerobic fermentative transformation, Appl. Environ. Microbiol. 1986, 51, 200–202.
Vorbeck, C., Lenke, H., Fischer, P., Knackmuss, H. J., Identification of a hydride–Mei- senheimer complex as a metabolite of 2,4,6- trinitrotoluene by a Mycobacterium strain, J. Bacteriol. 1994, 176, 932–934.
Wagener, S., Schink, B., Anaerobic degradation of non-ionic and anionic surfactants in
|
References |
257 |
|
|
|
enrichment cultures and fixed-bed reactors, |
Zehnder, A. J. B., Brock, T. D., Anaerobic |
|
Water Res. 1987, 21, 615–622. |
methane oxidation: occurrence and ecology, |
|
Wagener, S., Schink, B., Fermentative degra- |
Appl. Environ. Microbiol. 1980, 39, 194–204. |
|
dation of non-ionic surfactants and polyeth- |
Zehnder, A. J. B., Stumm, W., Geochemistry |
|
ylene glycol by enrichment cultures and by |
and biogeochemistry of anaerobic habitats, |
|
pure cultures of homoacetogenic and pro- |
in: Biology of Anaerobic Microorganisms |
|
pionate-forming bacteria, Appl. Environ. Mi- |
(Zehnder, A. J. B., ed.), pp. 1–38. New York |
|
crobiol. 1988, 54, 561–565. |
1988: Wiley. |
|
Wilkes, H., Rabus, R., Fischer, T., Armstroff, |
Zhang, X., Young, L. Y., Carboxylation as an |
|
A., Behrends, A., Widdel, F., Anaerobic |
initial reaction in the anaerobic metabolism |
|
degradation of n-hexane in a denitrifying |
of naphthalene and phenanthrene by sulfido- |
|
bacterium: further degradation of the initial |
genic consortia, Appl. Environ. Microbiol. |
|
intermediate (1-methylpentyl)succinate via |
1997, 63, 4759–4764. |
|
skeleton rearrangement, Arch. Microbiol. |
|
|
2002, 177, 235–243. |
|
|

259
9
Soil Remediation and Disposal
Michael Koning, Karsten Hupe, and Rainer Stegmann
9.1 Introduction
For the treatment of contaminated sites, securing as well as remediation methods are applied. While remediation achieves decontamination or reduction of pollutants, securing sets up technical barriers for the protection of the environment. Since the source of contamination remains in place and the technical barriers are subject to aging and environmental influences, securing measures often represent only a time restricted solution and future remediation activities become necessary.
Remediation methods are classified according to their operation location as well as to processing aspects. Thus, ex situ and in situ processes are available on the one hand, and thermal, chemical, physical, and biological processes on the other. The ex situ processes require excavation of the contaminated soil and soil treatment either at the site (on-site remediation) or at an external soil treatment plant (off-site remediation). In contrast, in situ treatment takes place at the site in the contaminated soil itself, without any soil excavation.
Thermal processes are used for the treatment of highly concentrated organic pollutants, but they are suitable only to a small extent for the elimination of heavy metals. With soil scrubbing, the coarse-grain fraction >63 µm is purified, transferring the pollutants into the water phase and/or into the fine-grain fraction. This fine fraction is highly loaded with pollutants and thus has to be treated and disposed of afterwards. The biopile process is applied on a large scale as a state-of-the-art technology. It is an effective process for the treatment of biologically degradable pollutants such as mineral oil and its derivatives, aliphatic hydrocarbons, phenols, formaldehyde, and other soil contaminants.
In remediation practice, there is a trend toward actions with a minimum of requirements. Contaminated sites are remediated or secured, depending on the intended after-use, e.g., housing, development of commercial or industrial facilities, or as recreational areas. Depending on the kind of use, specific target values have to be met after treatment. If the contaminated site is not being used and so far no major dangerous contamination of the groundwater, surface water, etc. has occurred,
Environmental Biotechnology. Concepts and Applications. Edited by H.-J. Jördening and J. Winter Copyright © 2005 WILEY-VCH Verlag GmbH & Co. KGaA, Weinheim
ISBN: 3-527-30585-8

260 9 Soil Remediation and Disposal
one relies more and more on natural attenuation processes in soil and groundwater. Economical active (e.g., selective biostimulation) and passive in situ measures (reactive walls, funnel and gate systems) are developed and need to be investigated with regard to their long-term effectiveness.
For the ex situ remediation of soils that are nearly not amenable to treatment (silty soils contaminated with chlorinated hydrocarbons, polycyclic aromatic hydrocarbons, or tar oil) combined processes with soil scrubbing as basic process are increasingly being considered. For the subsequent treatment of problematic polluted residues (fine-grain fraction, process waters, etc.), a variety of processes are available, e.g., suspension bioreactors, oxidation with ozone or H2O2, and thermal treatment.
Experiences in dealing with hazardous old sites have basically shown that balancing pre-investigations are of essential importance regarding the process evolution as well as the evaluation of remediation measures. Far-reaching investigations have been carried out in the field of microbiological (ex situ) treatment, and standardizations have been defined (Dechema, 1992; GDCh, 1996). In the future, emphasis should be placed on process evaluation for the in situ treatment so as to predict the processes of natural attenuation by balancing as far as possible the fate of pollutants.
9.2
Thermal Processes
Thermal soil purification is based mainly on transfer of the pollutants from the soil matrix into the gas phase by thermal energy input. The pollutants are released from the soil by vaporization and then burned. The polluted gas is purified further.
The different processing concepts for the thermal purification of contaminated soils are characterized by variations of the process parameters (e.g., temperature range, retention time for solids and waste gas in certain temperature zones, supply of oxygen, supply of reactive gases for gasification, supply of inert gas, kind of heat input, and optimum heat utilization, etc.). The large variability has led to a multitude of ex situ and in situ process combinations.
9.2.1
Thermal Ex Situ Processes
The basic principle of a thermal soil purification plant includes the following processing steps (Figure 9.1):
1.soil conditioning
2.thermal treatment
3.waste gas purification
In soil conditioning, the contaminated soil is freed of interfering foreign matter (e.g., scrap, plastics) broken, sieved, and homogenized by mechanical preparation processes to be of a particle size consistent with the technical requirements of the subsequent thermal treatment [<20 mm (fluidized bed) to 80 mm]. During thermal
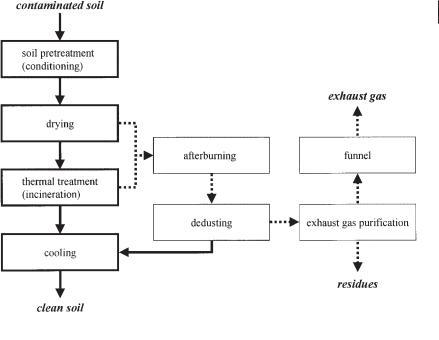
9.2 Thermal Processes 261
Fig. 9.1 The thermal ex situ treatment process.
treatment the soil is heated so that volatile pollutants are stripped from it. In the gas phase above the soil, the combustion of pollutants takes place, but in this phase complete destruction of volatile pollutants cannot be achieved. For this reason the gases are burned in an afterburner chamber at high temperature (~1200 °C) for a certain retention time. Under these conditions dioxins are also destroyed.
Two different technologies are mainly used in thermal soil treatment:
•processes with an exclusively thermal effect: directly and indirectly heated processes (pyrolysis) with subsequent gas treatment (afterburning, condensation) (rarely used)
•processes with a thermal effect and additional measures (common practice)
Processes having an exclusively thermal effect (directly and indirectly heated) are conventional processes available on a large scale, using, e.g., rotary kiln plants, fluid- ized-bed plants, and sintering strand plants, for which long-term experience in practice has been achieved. Most of the processes work in the low-temperature range of 100 to 550 °C (residence time of the soil in the furnace 10–20 min; throughput 20 to 30 Mg (megagramms) h–1 (VDI, 2002)) at which the structure of the soil is not fundamentally changed and humic components are only partly destroyed. In the early days of thermal soil treatment, high-temperature processes were practiced in which the soil was heated to temperatures of 800 to 1100 °C (residence time of the soil in the furnace 20–45 min; throughput 2 to 7 Mg h–1 (VDI, 2002)). Under these conditions, partial liquefying or sintering of the soil particles is possible. At the same

262 9 Soil Remediation and Disposal
time, the organic components and the clay minerals of the soil are destroyed to a large extent, hydroxides are changed into oxides, and primary minerals are crushed by gritting. Furthermore, the quantity of NOx increases rapidly at temperatures >1000 °C, so that special equipment could be necessary for decreasing NOx in the waste gas.
Depending on the contents of pollutant, water, and fine soil particles, thermal treatment with indirect heating is carried out under reducing conditions (pyrolysis) in a temperature range between 400 and 600 °C (residence time of the soil in the furnace approx. 45 min; throughput 5 to 13 Mg h–1 (VDI, 2002)).
The processes using an exclusively thermal effect show significant differences regarding the waste gas purification systems used. The selection of the waste gas purification system used, is decisive influenced by the local regulations on air emissions. Usually, aggregates are used, which are normally used for flue gas purification in large-scale power plants and in waste incineration plants. The waste gas purification equipment in these processes mainly contains three partial units:
1.high-temperature afterburners
2.dedusters
3.flue gas purifiers
The separation of fine soil particles from the gas stream (dedusting) is done by means of cyclones, hot gas filters, or combinations of different filter techniques specifically adapted to the process. Although the organic pollutants (hydrocarbons) are completely oxidized during the afterburning step (high temperature afterburning) at 900–1300 °C, flue gas purification minimizes the amounts of inorganic pollutants such as hydrogen chloride, hydrogen fluoride, and sulfur dioxide and brings the levels of dust and heavy metal emissions down to target values. Fundamentally, flue gas purification processes can be divided into a dry sorption process, a wet cleaning process (wet scrubbers), and a combination of both (semidry process). If reduction of nitrogen oxides is required, special measures are necessary. The efficiency of the various processes increases from the dry sorption through the semidry up to the wet scrubber process – as do the total costs. But at the same time, the degree of pollutant separation effected by these processes increases so that – especially regarding the wet cleaning processes – high waste gas quality requirements can be met. To guarantee the target values of the waste gas, activated carbon filters are used as a final step in almost all processes. An operation without excess water can be achieved by treatment of the washing water (heavy metal precipitation and stabilization) and subsequent recirculation for semidry and wet cleansing processes (Fortmann and Jahns, 1996).
Processes involving thermal effects and additional measures include various designs that use a temperature range of 60–350 °C, at which the pollutants (especially low-boiling and medium-boiling hydrocarbons) are stripped from the soil by the influence of heat and additional measures (e.g., steam stripping, vacuum). The efficiency of the process depends not only on the effect of the temperature, but also on the physical properties of the pollutants.