
Environmental Biotechnology - Jordening and Winter
.pdf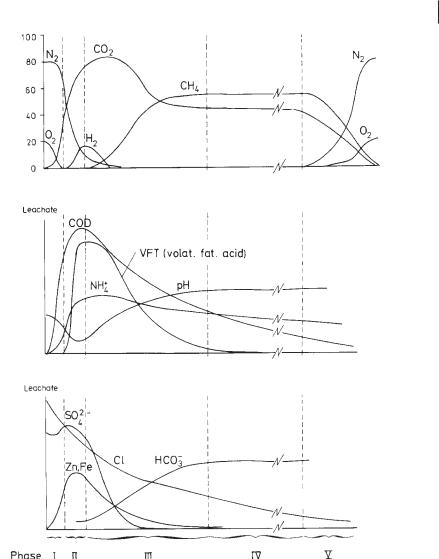
15.2 Biochemical Processes in Sanitary Landfills 377
Time courses of compositions of leachate and gas in a landfill cell (Christensen and Kjeldsen, 1989).
binson, 1989), the latter due to hydrolysis and fermentation of proteinaceous compounds in particular.
The second intermediate anaerobic phase (Phase III, Figure 15.1) starts with slow growth of methanogenic bacteria. This growth may be inhibited by an excess of organic volatile acids, which are toxic to methanogenic bacteria at concentrations of 6000–16 000 mg L–1 (Stegmann and Spendlin, 1989). The methane concentration in the gas increases, while hydrogen, carbon dioxide, and volatile fatty acids decrease.

378 15 Landfill Systems, Sanitary Landfilling of Solid Wastes, and Long-term Problems with Leachate
Moreover, the concentration of sulfate decreases owing to biologic reduction. Conversion of fatty acids causes an increase in pH values and alkalinity, with a consequent decrease in solubility of calcium, iron, manganese, and heavy metals. The latter are probably precipitated as sulfides. Ammonia is released and is not converted in the anaerobic environment.
The third phase of anaerobic degradation (Phase IV, Figure 15.1) is characterized by methanogenic fermentation elicited by methanogenic bacteria. At this stage, the composition of leachate is characterized by almost neutral pH values and low concentrations of volatile acids and total dissolved solids; the biogas generally consists of more than 50% methane. This confirms that solubilization of the majority of organic components has decreased at this stage of landfill operation, although the process of waste stabilization will continue for several decades.
Leachates produced during this phase are characterized by relatively low BOD5 values and low ratios of BOD5/COD. Ammonia continues to be released by the firststage acetogenic process.
Table 15.1 shows the ranges of leachate concentrations depending on the degradation phase for some relevant parameters. Ehrig (1990) compiled leachate concentrations from German landfills from the 1970s and 1980s. According to his evaluation the organics (COD, BOD5, TOC), as well as AOX, SO4, Ca, Mg, Fe, Mn, Zn, and Cr, are determined by the biochemical processes in the landfill, and there are striking differences between the acid phase and the methanogenic phase.
Kruse (1994) investigated 33 landfills in Northern Germany, the leachate concentrations mainly derive from the late 1980s and early 1990s. He defined three characteristic periods according to the BOD5/COD ratio:
• acid phase: |
BOD5/COD ≥ 0.4 |
• intermediate phase: |
0.4 > BOD5/COD > 0.2 |
• methanogenic phase: |
BOD5/COD ≤ 0.2 |
Between the two investigations there are significant differences concerning the quantities of organic parameters. In the younger landfills (Kruse, 1994), leachate concentrations of COD, BOD5, and TOC are lower than those determined by Ehrig (1990) some 10 years earlier. This can be explained by developments in the technology of waste landfilling. In many younger landfills waste deposition and compaction in thin layers, in combination with an aerobic pretreated bottom layer, were carried out. This led to a reduction in the period for the acid phase and to an accelerated conversion of organic leachate components into the gaseous phase, as well as the degradation of organics to methane and carbon dioxide.
15.2.3
Factors Affecting Leachate Composition
The chemical composition of leachate depends on several parameters, including those concerning waste mass and site localization and those deriving from design and management of the landfill. Of the former. the main factors influencing leachate quality are discussed in this section.

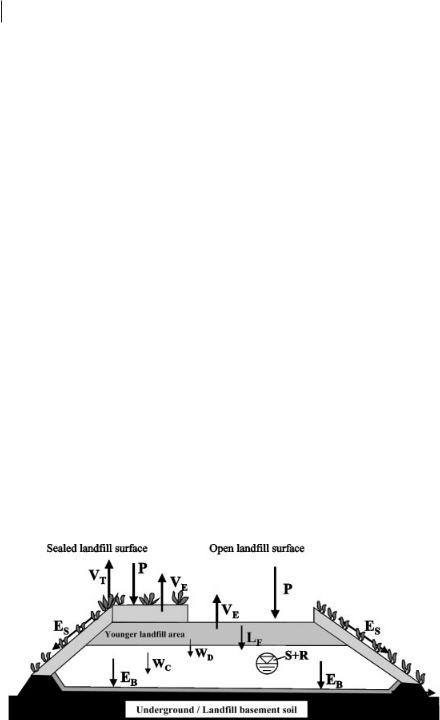

15.3 Sanitary Landfilling and Leachate Control Strategies 381
15.2.3.3Landfill Age
Variations in leachate composition and in quantity of pollutants removed from waste are often attributed to landfill age, defined as the time from the deposition of waste or the time from the first appearance of leachate. Landfill age obviously plays an important role in the determination of leachate characteristics governed by the type of waste stabilization processes. We should emphasize that variations in composition of leachate do not depend exclusively on landfill age but also on the degree of waste stabilization and the volume of water that infiltrates the landfill. The pollutant load in leachate generally reaches maximum values during the first years of operation of a landfill (2–3 years) and then gradually decreases in the following years. This trend is generally applicable to organic components, the main indicators of organic pollution (COD, BOD5, TOC), microorganism population, and the main inorganic ions (heavy metals, Cl, SO4, etc.).
15.3
Sanitary Landfilling and Leachate Control Strategies
15.3.1
Leachate Problems in Landfills
The most typical detrimental effect of leachate discharge into the environment is that of groundwater pollution. To prevent this, the first step in landfill design development was to site the landfill far from the groundwater table and/or far from groundwater extraction wells. Thus more attention was focused on studying the hydrogeology of the area so as to identify the best siting of the landfill.
A further step in landfill technology was to site the landfill in low-permeability soil and/or to engineer impermeable liners to contain wastes and leachate. Containment, however, poses the problem of leachate treatment.
Discharge of leachate into the environment is today considered under more restrictive views. The reasons for this are:
•many severe instances of groundwater pollution by landfills
•the greater hazard posed by the size of landfill (larger than in the past)
•the need to comply with more and more restrictive legislation regarding quality standards for wastewater discharges
The leachate problem accompanies a landfill from its beginning to many decades after its closure. Therefore, leachate management facilities should also last and their effectiveness be ensured over a long period of time.
15.3.2
Sanitary Landfilling and Legal Requirements
In view of all the aspects mentioned above, leachate control strategies involve the input (waste and water), the reactor (landfill), and the output (leachate and gas). This

382 15 Landfill Systems, Sanitary Landfilling of Solid Wastes, and Long-term Problems with Leachate
is one of the reasons why the German Landfill Ordinance (DEPV, 2002), the Technical Instructions on Waste from Human Settlements (TASI, 1993), laid down standards for disposal, including the collection, treatment, storage, and landfilling of wastes from human settlements. State-of-the-art technology is required and, with the so-called multibarrier concept, it is the waste to be dumped itself that forms the most important barrier. The other barriers are the geological barrier of the landfill site, base sealing with an effective drainage system, and surface sealing after a landfill section has been completely filled. Considering these major aspects the Instructions on Waste from Human Settlements define two classes of landfills:
•landfill class I
–particularly high standards for mineralization levels of the waste to be dumped
–relatively low standards for landfill deposit sites and landfill sealing (base and surface sealing)
•landfill class II
–lower standards for mineralization levels of the waste to be dumped (mainly for municipal solid wastes)
–considerably higher standards for landfill deposit sites and landfill sealing than apply to landfill class I
15.3.3
Control of Waste Input and Pretreatment before Deposition
The first step in the waste input control strategy should be that of minimizing the amount of waste to be landfilled. This can be achieved by waste avoidance, separate collection activities, recycling centers for recyclables, incineration, and mechani- cal–biological pretreatment of residual municipal solid waste (MSW) or composting of biowaste.
With regard to its properties, the waste can be separated into different fractions: a light fraction of high calorific value, in some instances also a mineral fraction and a fraction rich in organics can be gained. Some of these fractions also have reutilization potential. The mechanical–biological pretreatment of MSW can be used within a waste management concept as a sole process or in combination with thermal pretreatment (Fig. 15.3).
After the mechanical–biological pretreatment, significant reductions in the remaining emissions, in the range 70%–90% in terms of loads and concentrations, are possible. Figure 15.4 shows as an example the low leachate concentration of a landfill section filled with MBP residues.
15.3.4
Control of Water Input and Surface Sealing Systems
The strategy for water input control is strictly related to the quality of the waste to be landfilled. For nonbiodegradable waste and according to its potential hazard to the environment, prevention of water infiltration can be used as the main option (usually by means of top sealing). In contrast, for biodegradable waste, water input must
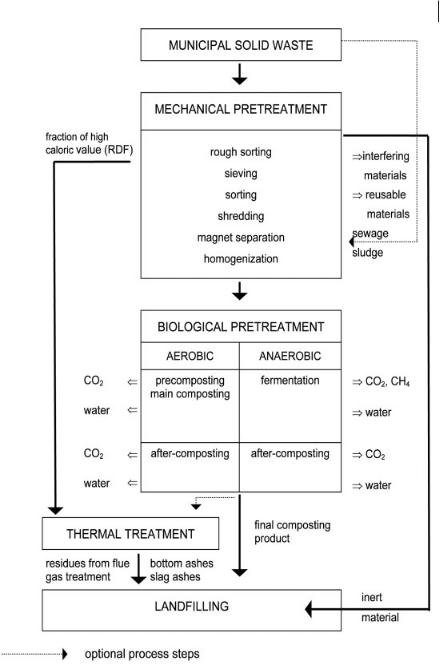
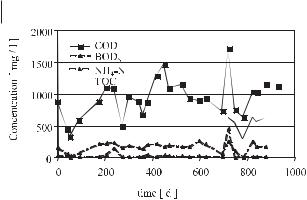
384 15 Landfill Systems, Sanitary Landfilling of Solid Wastes, and Long-term Problems with Leachate
Fig. 15.4 Effects of mechanical–biological treatment on leachate concentrations in an MBP landfill section.
be assured until a high degree of biostabilization is achieved. Here, water input should be limited to the strictly necessary amount and minimization techniques should be used. The most important parameters in this regard are (Stegmann et al., 1992):
•siting of landfills in low precipitation areas, if possible
•planting the topsoil with species characterized by high evapotranspiration
•surface lining in critical hydrological conditions
•surface water drainage and diversion
•high compaction of the refuse in place
•measures to prevent risks of cracking owing to differential settlement
Furthermore, utilization of intermediate covers in the landfill operation area could represent a useful leachate minimization technique.
The two final surface sealing systems that must be used, according to the regulations of the TASI (TASI, 1993), are shown in Figure 15.5.
15.3.5
Control of Leachate Discharge into the Environment and Base Sealing Systems
As mentioned above, uncontrolled leachate discharge into the environment is the main pollution risk of waste deposition. For this reason, regulations are more restrictive today (DepV, 2002, TASI, 1993). The following tools are used:
•The lining system should be based on the multibarrier effect (double or triple liners). Quality control of materials and construction should be improved to ensure higher safety and durability.
•A rational drainage and collection system is important to avoid accumulation of leachate inside the landfill. The main problems of drainage systems are proper choice of materials, clogging, durability, and maintenance.
In Figure 15.6 the base sealing systems for class I and II landfills, according to the requirements of the TA Siedlungsabfall (TASI, 1993), are shown. The sealing
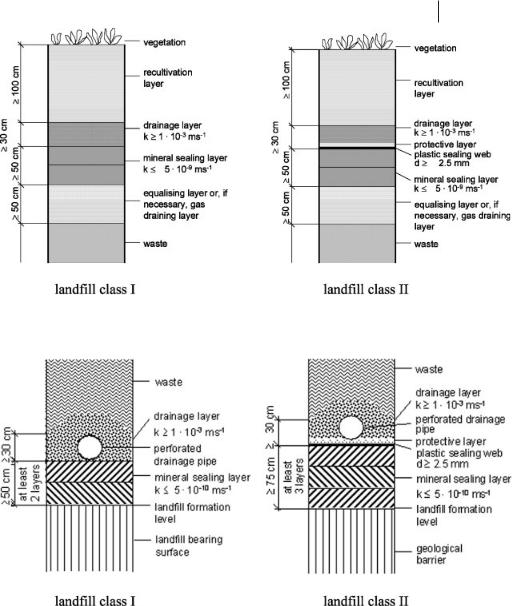
15.3 Sanitary Landfilling and Leachate Control Strategies 385
Fig. 15.5 Landfill surface sealing systems (TASI, 1993).
Fig. 15.6 Landfill base sealing systems (TASI, 1993).
systems must be arranged on the landfill base and on the sloping areas. For landfill class II, the geological barrier should comprise naturally arranged, slightly permeable bedrock several meters thick. If this requirement is not met, a homogenous equalizing layer at least 3 m thick and having a permeability of k ≤ 1 × 10–7 ms–1 has to be installed.
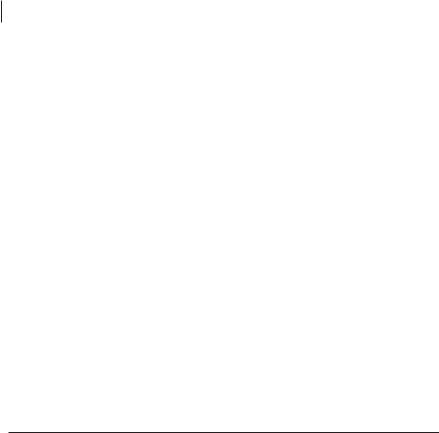
386 15 Landfill Systems, Sanitary Landfilling of Solid Wastes, and Long-term Problems with Leachate
The landfill base must be at least 1 m above the highest expected groundwater level, once settling under the load of the landfill has come to an end.
The surface of the base sealing system must be formed in the manner of a roof profile. Once the sealing bearing surface has finished settling, the surface of the sealing layer must exhibit a transverse gradient of ≥3% and a longitudinal gradient of ≥1%. Perforated pipes (collectors), additionally capable of being rinsed and monitored, must be provided for the collection and discharge of leachate. The leachate must be channeled by means of free flow into drainage shafts that must be installed outside the dumping area.
15.3.6
Leachate Treatment
Leachate has always been considered a problematic wastewater from the point of view of treatment, because it is highly polluted and its quality and quantity are modified with time in the same landfill. Today, according to the increasingly restrictive limits for wastewater discharge, complicated and costly treatment facilities are required.
Table 15.2 shows the current German requirements for leachate quality before discharge. Several parameters are of great importance for the treatment technology that has to be applied, mainly COD and AOX, but also nitrogen and BOD5. The first
Table 15.2 Limiting concentrations for the discharge of treated leachate according to German standards (51. Anhang Rahmen-AbwasserV, 1996).
Parameter |
Limiting Concentration |
|
mg L–1 |
|
|
COD |
200 |
BOD5 |
20 |
Nitrogen, total (sum of NH4 + NO2 + NO3) |
70 |
Phosphorus, total |
3 |
Hydrocarbons |
10 |
Nitrite-nitrogen |
2 |
AOX |
0.5 |
Mercury |
0.05 |
Cadmium |
0.1 |
Chromium |
0.5 |
Chromium (VI) |
0.1 |
Nickel |
1 |
Lead |
0.5 |
Copper |
0.5 |
Zinc |
2 |
Cyanide, easy liberatable |
0.2 |
Sulfide |
1 |
|
|