
Kluwer - Handbook of Biomedical Image Analysis Vol
.1.pdfPreface
Chapter 1 presents IVUS. Intravascular ultrasound images represent a unique tool to guide interventional coronary procedures; this technique allows to supervise the cross-sectional locations of the vessel morphology and to provide quantitative and qualitative information about the causes and severity of coronary diseases. At the moment, the automatic extraction of this kind of information is performed without taking into account the basic signal principles that guide the process of image generation. In this handbook, we overview the main physical principles and factors that affect the IVUS generation; we propose a simple physics-based approach for IVUS image simulation that is defined as a discrete representation of the tissue by individual scatterers elements with given spatial distribution and backscattering differential cross sections. In order to generate the physical model that allows to construct synthetic IVUS images, we analyze the process of pulse emission, transmission, and reception of the ultrasound signal as well as its interaction with the different tissues scatterers of the simulated artery. In order to obtain the 3D synthetic image sequences, we involve the dynamic behavior of the heart/arteries and the catheter movement in the image generation model. Having an image formation model allows to study the physics parameters that participate during the image generation and to achieve a better understanding and robust interpretation of IVUS image structures. Moreover, this model allows to comprehend, simulate, and solve several limitations of IVUS sequences, to extract important image parameters to be taken into account when developing robust image processing algorithms as well as to construct wide synthetic image sequence databases in order to validate different image processing techniques.
Chapter 2 presents research in PET. The last few decades of the twentieth century have witnessed significant advances in multidimensional medical imaging, which enabled us to view noninvasively the anatomic structure of internal organs with unprecedented precision and to recognize any gross pathology of organs and diseases without the need to “open” the body. This marked a new era of medical diagnostics with many invasive and potentially morbid procedures being substituted by noninvasive cross-sectional
xi
xii |
Preface |
imaging. Continuing advances in instrumentation and computer technologies also accelerated the development of various multidimensional imaging modalities that possess a great potential for providing, in addition to structural information, dynamic and functional information on biochemical and pathophysiologic processes or organs of the human body. There is no doubt that substantial progress has been achieved in delivering health care more efficiently and in improving disease management, and that diagnostic imaging techniques have played a decisive role in routine clinical practice in almost all disciplines of contemporary medicine. With further development of functional imaging techniques, in conjunction with continuing progress in molecular biology and functional genomics, it is anticipated that we will be able to visualize and determine the actual molecular errors in a specific disease very soon, and be able to incorporate this biological information into clinical management of that particular group of patients. This is definitely not achievable with the use of structural imaging techniques. In this chapter, we will take a quick tour of a functional imaging technique called positron emission tomography (PET), which is a primer biologic imaging tool able to provide in vivo quantitative functional information in most organ systems of the body. An overview of this imaging technique including the basic principles and instrumentation, methods of image reconstruction from projections, some specific correction factors necessary to achieve quantitative images are presented. Basic assumptions and special requirements for quantitation are briefly discussed. Quantitative analysis techniques based on the framework of tracer kinetic modeling for absolute quantification of physiological parameters of interest are also introduced in this chapter.
Chapter 3 presents MRI. With time and experience, flow characteristics in human body have become ideal modality for the evaluation of vascular disease. With this aim, in this chapter, the topic of magnetic resonance angiography (MRA) is discussed with emphasis of physical principles of MRA techniques in current practice for acquisition and display of vascular anatomy as well as flow measurement. The main techniques are described, 1 TOF (time of flight) MRA; 2. phase contrast (PC) MRA. Each technique can be performed using 2D Fourier transform (2DFT) or 3D FT(3DFT). Each of these techniques lends itself to a different type of clinical application. TOF MRA is based on 2D or 3D gradient echo (CRE) technique. For 2D TOF MRA, use of presaturation pulse and slice selection above or below each slice are discussed to eliminate the signal from vessels flowing in the opposite direction. Usually, FC (flow compensation) is used perpendicular to the vessel lumen. Considerations on selection of scan parameters TR and TE are discussed. The performance is highlighted in faster scanning and capability of increased FRE because each slice is an entry slice. 3D TOF MRA pulse sequence for 3D TOF MRA is described as capable of obtaining a slab of up to 60 slices. Basic physics principles are highlighted for inflow effects (TOF), TR effect, imaging volume effect, flow phase effects (volume imaging), gradient motion rephasing by velocity rephasing, high order rephasing, inflow rephasing, and turbulant flow effect. Various acquisition methods are discussed in current practice such as inflow (TOF), flow adjusted gradient (FLAG), rapid
Preface |
xiii |
sequential excitation (RSE), and black blood MRA. Flow imaging is highlighted to get better magnitude contrast, phase contrast, inflow 3D (volume imaging), and 2D(TOF), TR. Other advancements are discussed in the field of MRA such as flow suppression, inflow and maximum intensity projection (MIP) processing, quantification of flow in tissue by real time acquisition and evaluation (RACE), phase mapping, and bolus tracking. Physical principles of inflow analysis in vessels are current interest in MRA methods. In summary, new MRA approaches are on the horizon which promise earlier diagnosis of tissue ischemia by tissue-free water and perfusion besides vascular anatomy and pathology. Time and experience will demonstrate the possibility of combining parenchymal and vascular anatomy with end-organ perfusion into a single imaging examination. Recent state-of-the-art on the physical basis of MRA methods is introduced to interested readers.
Chapter 4 presents work in level sets. In this chapter, we will begin with a concise description of the basic level set method, its origins, and why it has become so popular in a wide range of applications. We will then discuss many of the modifications and enhancements to the level set method which have made it a robust tool for propagating fronts. These modifications will include the use of the fast marching method and the more general ordered upwind methods, narrow-banding, velocity extensions, and particle level set methods. The chapter will conclude with techniques used for coupling the leve set method to different physical problems, including coupling to elliptic solvers such as the ghost-fluid method, immersed interface method, and the extended finite element method. Implementation details will be provided throughout the text so that the methods can be quickly implemented, as well as specific examples of results obtained with these methods.
Chapter 5 presents work in shaping in shading. In many applications, for instance, visual inspection in robot vision, autonomous land vehicle navigation to name a few, a procedure of recovering three-dimensional surfaces of unknown objects is of considerable practical interest. In this chapter, we consider one of the reconstruction methods: shape from shading (SFS). The chapter is organized as follows: the first section serves as a brief review of the SFS models, their history, and recent developments. Section 2 provides certain mathematical background related to SFS. It discusses some selected numerical methods for solving discretized SFS problems. The emphasis is given to the well developed method: finite difference method (FDM). Section 3 is devoted to the illustration of numerical techniques for solving SFS problems. It concerns related algorithms and their implementations. The section ends with a discussion about the advantages and disadvantages of the algorithms introduced in this section. The last section attempts to introduce the recently developed wavelet-based methods by using an example. A part of the section, however, is distributed to a brief introduction of the basic facts of wavelet theory. In the hope of readers being able to extrapolate the elements presented here to initiate the understanding of the subject on his/her own, the chapter concludes with some remarks on other advanced methods. Finally, we include an intensive set of references to make up
xiv |
Preface |
whatever important spirits for which the authors have indeed hardly to touch in this short chapter.
Chapter 6 presents work in the area of wavelet transforms. Wavelet transforms and other multiscale analysis functions have been used for compact signal and image representations in denoising, compression, and feature detection processing problems for about 20 years. Numerous research works have proven that space-frequency and space-scale expansions with this family of analysis functions provided a very efficient framework for signal or image data. The wavelet transform itself offers great design flexibility. Basis selection, spatial-frequency tiling, and various wavelet threshold strategies can be optimized for best adaptation to a processing application, data characteristics, and feature of interest. Fast implementation of wavelet transforms using a filter-bank framework enables real time processing capability. Instead of trying to replace standard image processing techniques, wavelet transforms offer an efficient representation of the signal, finely tuned to its intrinsic properties. By combining such representations with simple processing techniques in the transform domain, multiscale analysis can accomplish remarkable performance and efficiency for many image processing problems. Multiscale analysis has been found particularly successful for image denoising and enhancement problems given that a suitable separation of signal and noise can be achieved in the transform domain (i.e. after projection of an observation signal) based on their distinct localization and distribution in the spatial-frequency domain. With better correlation of significant features, wavelets were also proven to be very useful for detection and matching applications. One of the most important features of wavelet transforms is their multiresolution representation. Physiological analogies have suggested that wavelet transforms are similar to low level visual perception. From texture recognition and segmentation to image registration, such multiresolution analysis gives the possibility of investigating a particular problem at various spatial-frequency (scales). In many cases, a “coarse to fine” procedure can be implemented to improve the computational efficiency and robustness to data variations and noise.
Without trying to cover all the issues and research aspects of wavelet in medical imaging, we focus our discussion in this chapter on three topics: image denoising/enhancement, image segmentation, and image registration using wavelet transforms. We will introduce the wavelet multiscale analysis frame work and summarize related research work in this area and describe recent state-of-the-art techniques.
Chapter 7 presents work in deformable models. Traditional deformable models cannot deal with topological changes and suffer from the strong sensitivity to the initial contour position. Besides, when using deformable surfaces, memory limitations can lower the performance of segmentation applications for large 3D images, or even make it undoable. In this chapter we describe some techniques to address these limitations. The problem of topological changes is addressed by the T-surfaces model by embedding a deformable model in the framework of a simplicial decomposition of the domain. The sensitivity to initialization has been addressed through automatic techniques for obtaining an initial contour
Preface |
xv |
closer to the desired boundary. Efficient memory utilization can be obtained through out-of-core techniques. Also, diffusion schemes can be explored in order to improve the convergence of the model toward the desired boundaries. We demonstrate the discussed techniques for segmentation of both gray level and color medical images. Finally, we present perspectives and challenges in the area of deformable models.
Chapter 8 presents work in the area of application. This chapter describes level set techniques for extracting surface models from a broad variety of biological volume datasets. These techniques have been incorporated into a more general framework that includes other volume processing algorithms. The volume datasets are produced from standard 3D imaging devices such as MR, CT, ultrasound and are all noisy samplings of complex biological structures with boundaries that have low and often varying contrasts. The level set segmentation method, which is well documented in the literature, creates a new volume from the input data by solving an initial value partial differential equation (PDE) with user-defined feature-extracting terms. Given the local/global nature of these terms, proper initialization of the level set algorithm is extremely important. Thus, level set deformations alone are not sufficient, they must be combined with powerful preprocessing and data analysis techniques in order to produce successful segmentations. In this chapter the authors describe the preprocessing and data analysis techniques that have been developed for a number of segmentation applications, as well as the general structure of our framework. Several standard volume processing algorithms have been incorporated into the framework in order to segment datasets generated from MRI, CT and TEM scans. A technique based on moving least-squares has been developed for segmenting multiple nonuniform scans of a single object. New scalar measures have been defined for extracting structures from diffusion tensor MRI scans. Finally, a direct approach to the segmentation of incomplete tomographic data using density parameter estimation is presented. These techniques, combined with level set surface deformations, allow us to segment many different types of biological volume datasets.
Chapter 9 presents work in the area of segmentation using the tools of level sets and statistics. The book also demonstrates a chapter in the area of mathematical foundations of the statistical-based approaches that have been known to provide robust performance on medical imaging. Other approaches-based level sets will also be covered. We will introduce 2D and 3D phantoms to validate the theory. Applications will include CT, MRI, and MRA volume segmentation of the human brain, and CT segmentation of the lungs.
Chapter 10 presents work in the area of level sets with classifier embedded for snakes. The standard geometric or geodesic active contour is a powerful segmentation method, whose performance however is commonly affected by the presence of weak edges and noise. Since image modalities of all types in medical imaging are prone to such outcomes, it is important for geometric snakes to develop some level of immunity toward them. In this chapter, a region-aided geometric snake, enhanced for more tolerance toward weak edges and noise,
xvi |
Preface |
is introduced. It is based on the principle of the conjunction of the traditional gradient flow forces with new region constraints. We refer to this as the regionaided geometric snake or RAGS. The RAGS formulation is easily extended to deal with color images. Quantitative comparisons with other well-known geometric snakes in synthetic noisy images are presented. We also show the evaluation of RAGS with application to the localization of the optic disk in color retinal images. Many other images are also used to demonstrate the proposed method.
Finally, Chapter 11 presents work in the area of level set applications for missing boundaries. The authors present application of the semi-implicit complementary volume numerical scheme to solving level set formulation of Riemannian mean curvature flow in image segmentation, edge detection, missing boundary completion, and subjective contour extraction. Our computational method is robust, efficient, and stable without any restriction on a time step. The computational results related to medical image segmentation with partly missing boundaries and subjective contour extraction are presented.
Contents
1. |
A Basic Model for IVUS Image Simulation . . . . . . . . . . . . . . . . . . . . . . . |
1 |
|
Misael Rosales and Petia Radeva |
|
2. |
Quantitative Functional Imaging with Positron Emission |
|
|
Tomography: Principles and Instrumentation . . . . . . . . . . . . . . . . . . |
57 |
|
Koon-Pong Wong |
|
3. |
Advances in Magnetic Resonance Angiography and Physical |
|
|
Principles . . . . . . . . . . . . . . . . . . . . . . . . . . . . . . . . . . . . . . . . . . . . . . . . . |
117 |
|
Rakesh Sharma, and Avdhesh Sharma |
|
4. |
Recent Advances in the Level Set Method . . . . . . . . . . . . . . . . . . . . . . . |
201 |
|
David Chopp |
|
5. |
Shape From Shading Models . . . . . . . . . . . . . . . . . . . . . . . . . . . . . . . . . . |
257 |
|
Xiaoping Shen and Lin Yang |
|
6. |
Wavelets in Medical Image Processing: Denoising, Segmentation, |
|
|
and Registration . . . . . . . . . . . . . . . . . . . . . . . . . . . . . . . . . . . . . . . . . . . |
305 |
|
Yinpeng Jin, Elsa Angelini, and Andrew Laine |
|
7. |
Improving the Initialization, Convergence, and Memory Utilization |
|
|
for Deformable Models . . . . . . . . . . . . . . . . . . . . . . . . . . . . . . . . . . . . . |
359 |
|
Gilson A. Giraldi, Paulo S. Rodrigues, Leandro S. Marturelli, and |
|
|
Rodrigo L. S. Silva |
|
8. |
Level Set Segmentation of Biological Volume Datasets . . . . . . . . . . . . |
415 |
|
David Breen, Ross Whitaker, Ken Museth, and Leonid Zhukov |
|
9. |
Advanced Segmentation Techniques . . . . . . . . . . . . . . . . . . . . . . . . . . . . |
479 |
|
Aly A. Farag, Mohamed N. Ahmed, Ayman El-Baz, and |
|
|
Hossam Hassan |
|
10. |
A Region-Aided Color Geometric Snake . . . . . . . . . . . . . . . . . . . . . . . . . |
535 |
|
Xianghua Xie and Majid Mirmehdi |
|
xvii
xviii |
Preface |
11. Co-Volume Level Set Method in Subjective Surface
Based Medical Image Segmentation . . . . . . . . . . . . . . . . . . . . . . . . . . 583 Karol Mikula, Alessandro Sarti, and Fiorella Sgallari
The Editors . . . . . . . . . . . . . . . . . . . . . . . . . . . . . . . . . . . . . . . . . . . . . . . . . . . . . 627
Index . . . . . . . . . . . . . . . . . . . . . . . . . . . . . . . . . . . . . . . . . . . . . . . . . . . . . . . . . . 633
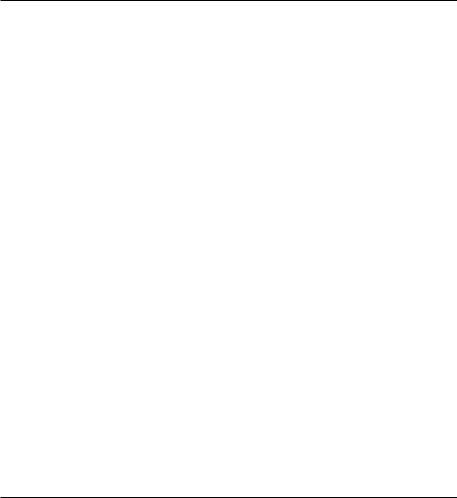
Chapter 1
A Basic Model for IVUS Image
Simulation
Misael Rosales1 and Petia Radeva2
Introduction
Arteriosclerotic cardiovascular diseases [1], which increase their incidence in vertiginous form, were the principal cause of mortality in the industrialized countries in the latter half of the 1950s, and cause more deaths today than deaths due to cancer and accidents. In effect, arteriosclerotic diseases in general (infarct to miocardio, angina of chest, cerebrovascular accidents, and arterial diseases of the legs and arms) have caused, and continue causing, more deaths and increased consultations in emergency rooms and doctors’ offices than any other disease in this century, including AIDS. Coronary arterial diseases develop due to a narrowing (stenosis) of the walls of the coronary arteries, caused by the accumulation of fibrotic material and calcium crystals [2]. In humans, the development of this type of plaque normally takes between 15 and 20 years. There exist complicated injuries, which generate the formation of thrombus; the rupture of the plaque can be considered an important mechanism of acute coronary syndromes. An imminent problem if the arteries narrow too much due to stenosis is that the blood flow diminishes significantly. A total occlusion can take
1 Laboratorio de Fısica´ Aplicada, Facultad de Ciencias, Departamento de Fısica´ de la
Universidad de los Andes Merida,´ Venezuela
2 Centre de Visio´ per Computador, Universidad Autonoma´ de Barcelona, Edifici O, Campus
UAB, 08193 Bellaterra, Barcelona, Spain
1

2 |
Rosales and Radeva |
Figure 1.1: Typical 2D IVUS image indicating the location of the principal morphological arterial structures and artifacts.
place if the increase in stenosis persists and can become serious due to a thrombosis. The result can be an infarct. The introduction of intravascular ultrasound (IVUS) [3, 4] in the field of medical image as an exploratory technique has made a significant change to the understanding of thearterial diseases and individual patterns of diseases in coronary arteries. Although coronary angiography [5, 6] provides with 2D information about the coronary anatomy, serving as a guide in operations, it has limitations when not allowed to access the mechanism of the disease, its composition, and its extent. On the contrary, the IVUS technique shows the cross-section (Fig. 1.1) of the artery, allowing an evaluation of the plaque as well as of the different layers in the arterial wall. The IVUS image [2, 5, 6] provides qualitative (Fig. 1.2) information about the causes and severity of the narrowing of the arterial lumen, distinguishes the thrombus of the arteriosclerotic plaque, shows calcium deposits in the arterial wall, evaluates the changes and complications in the coronary arteries that occur after an intervention such as angioplasty, evaluates and diagnoses coronary arterial aneurysms, and diagnoses fissures of arterial coronary plaques: determination and location, dimensions, type (eccentric and concentric), and composition of the arteriosclerotic plaque.