
Kluwer - Handbook of Biomedical Image Analysis Vol
.2.pdf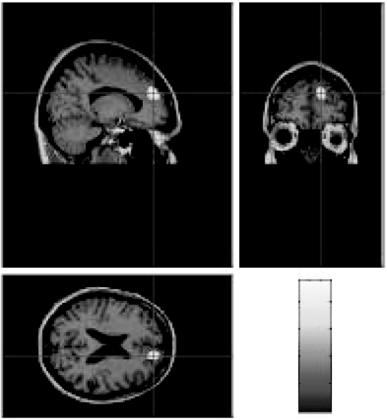
40 |
Leemput et al. |
8
6
4
2
0
Figure 1.20: Cross-sectional overlay of the detected focal cortical thickening locus. The colour scale increases with statistical significance. Significance is measures by comparison of feature measurements (cortical thickness over intensity gradient) to a control subject database (Color Slide).
1.7 Application to Schizophrenia
Several studies have reported morphological differences in the brains of schizophrenic patients when compared to normal controls [73], such as enlargement of the cerebral ventricles and decreased or reversed cerebral asymmetries. These findings suggest the presence of structural brain pathology in schizophrenia. Some hypotheses have been proposed about schizophrenia as a syndrome of anomalies in normal brain development [74] whose origin may be genetically determined. Characterization of the morphological processes in schizophrenia
Model-Based Brain Tissue Classification |
41 |
may lead to new pharmacological treatments aimed at prevention and cure rather than suppression of symptoms. There is in addition a particular focus on asymmetry as the defining characteristic of the human brain and the correlate of language. Techniques are required for describing and quantifying cerebral asymmetries to determine where these are located, how variable they are between individuals, and how the distribution in individuals with schizophrenia differs from that in the general population.
As an application of the framework proposed in this chapter, we present here a method for fully automatic quantification of cerebral grey and white matter asymmetry from MR images. White and grey matter are segmented after bias correction by the intensity-based tissue classification algorithm presented in section 1.2. Separation of the computed white and grey matter probability maps into left and right halves is achieved by nonrigid registration of the study image to a template MR image in which left and right hemispheres have been carefully segmented. The delineations of left and right hemispheres in the template image were transformed into binary label images, which were subsequently edited by morphological operations to match the brain envelope rather than the individual gyri and sulci to be more robust against differences in local cortical topology. The template image is matched to the study image by a combination of affine [21] and locally nonrigid transformations [24]. The resulting deformation field is then applied to the label images, such that matched outlines of left and right hemispheres are obtained. These are used to separate left and right halves in the original grey and white matter segmentations and, at the same time, to remove nonrelevant structures such as the cerebellum and brain stem. Finally, volumes for grey and white matter for each half of the brain separately are computed by integrating the corresponding probability maps within the brain regions of interest defined by the matched template image. Figure 1.21 illustrates that the grey and white matter segmentation maps obtained from the original images are correctly split in separate maps for left and right hemispheres by nonrigid registration with the labelled template image.
Various authors have presented alternative techniques to separate the brain hemispheres by the so-called midsagittal plane, defined as the plane that best fits the interhemispheric fissure of the brain [76] or as the plane that maximizes similarity between the image and its reflection relative to this plane [77, 78]. The advantage of the approach of intensity-driven non-rigid registration to a labelled template image, as presented here, is that it does not assume the boundary
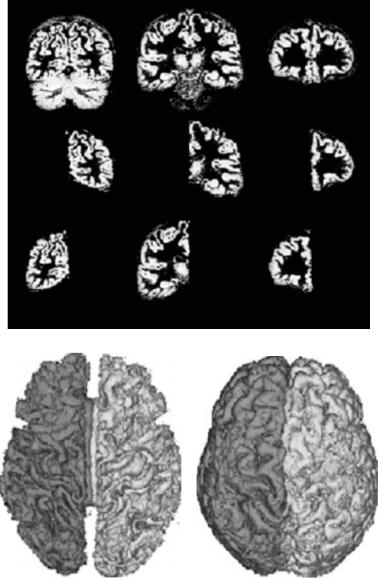
(a)
(b) |
(c) |
Figure 1.21: Left–right hemisphere grey matter and white matter separation on high quality high resolution data, consisting of a single sagittal T1-weighted image (Siemens Vision 1.5 T scanner, 3D MPRAGE, 256 256 matrix, 1.25 mm slice thickness, 128 slices, FOV = 256 mm, TR = 11.4 ms, TE = 4.4 ms) with good contrast between grey matter, white matter, and the tissues surrounding the brain. (a): Coronal sections through the grey matter segmentation map before (top row) and after left–right and cerebrum–cerebellum separation. (b) and (c): 3D Rendering of the segmented white and grey matter respectively. (Source: Ref. [75].)
Model-Based Brain Tissue Classification |
43 |
between both hemispheres to be planar. We refer the reader to [75] for further details and discussion of the results obtained.
1.8 Conclusion
The model-based brain tissue classification framework presented here was setup to analyze MR signal abnormalities in neuropathological disorders in large sets of multispectral MR data in a reproducible and fully automatic way. The overall strategy adopted was to build statistical models for normal brain MR images, with emphasis on accurate intensity models. Signal abnormalities are detected as model outliers, i.e., voxels that cannot be well explained by the model. Special attention has been paid to automatically estimate all model parameters from the data itself to eliminate subjective manual tuning and training.
As discussed in section 1.1.1, geometry-driven and intensity-driven methods are the two main paradigms for model-based segmentation. In this chapter, both paradigms are used in a sequential order. Complex intensity models are developed that automatically fit to the data. As a result, multispectral MR data are segmented fully automatically without prior knowledge about the appearance of the different tissue types in the images. Bias fields are automatically corrected for, and the partial volume effect is explicitly taken into account. The intensity models are complemented with image-based brain atlas models of prior tissue probabilities. These atlases are first iconically matched to the images and subsequently used as constraints during the tissue classifications. The matching was originally limited to affine transformations between atlas and study image (see also [79], but was later extended to nonrigid transformations as well (see also [14, 50, 75, 80]).
These attempts to combine the ability of intensity-driven methods to capture local shape variations with the general description of global anatomy provided by geometry-driven methods have been limited to a sequential use of both methods in separate processing steps. Atlas maps of prior distributions of the different tissue classes are first geometrically aligned to the images to be segmented. These transformed maps provide an initial approximate segmentation to initialize the classification algorithm but also provide an estimate of the prior class probabilities for each voxel during further iterations.
44 |
Leemput et al. |
In [13] an attempt was made to intertwine statistical intensity-based tissue classification and nonlinear registration of a digital anatomical template to segment both normal and abnormal anatomy. The algorithm iterates between a classification step to identify tissues and an elastic matching step to align a template of normal anatomy with the classified tissues. The alignment of the anatomical template is used to modify the classification to produce a spatially varying, rather than a global classification. The steps are iterated until the matched anatomy and the classification agree. However, this method currently needs manual supervision, and it still needs to be investigated how reliably this method can be automated. Moreover, the iterative procedure is not derived as the solution of an optimization problem. As a result, there is no guarantee that convergence, if at all, to a plausible solution can be obtained.
Wyatt and Noble [81], on the other hand, suggested a joint solution to the linked processes of segmentation and registration. They cast this as a maximum a posteriori (MAP) estimation of the segmentation labels and the geometric transformation parameters and pose the solution using MRF. Their results indicate that the addition of spatial priors (in the form of intermediate segmentation maps) leads to substantially greater robustness in rigid registration and the combination of data via registration improves the segmentation accuracy. However, their formulation is poorly suited for generalization to nonrigid registration.
D’Agostino et al. [82] explored the possibility of nonrigid image registration by maximizing an information theoretic measure of the similarity of voxel object labels directly, rather than of voxel intensities. Applied to intersubject MR brain image matching, such labels are obtained by the intensity-based tissue segmentation presented in this chapter, assigning each voxel a probability to belong to a particular tissue class. Using class labels as features for nonrigid image registration opens perspectives for integrating registration and segmentation as two cooperative processes in a single framework, by considering one of the images as an atlas that is nonrigidly warped onto the other and that provides a priori tissue distribution maps to guide the segmentation of the other image. The possibilities of such a method are enormous, since it would allow fully automated partial volume segmentation and bias correction of multispectral MR images with unknown tissue contrast, while deforming a label atlas at the same time. The quantification of intensity abnormalities could be confined to anatomical regions of interest. The brain could be automatically segmented into relevant substructures, allowing the quantification of changes in shape and volume, over
Model-Based Brain Tissue Classification |
45 |
time in one individual patient, or between populations. Knowledge of the deformation of the label atlas would allow nonrigid multimodal registration of images of different patients and provide a common reference frame for population studies. Deriving realistic statistical models for the shape of the human brain is, therefore, a major challenge for further research.
Questions
1.What is the parametric model of the MR bias field proposed in this chapter?
2.How are the parameters of the MR bias field estimated?
3.What are alternative models for the MR bias field?
4.How is the atlas-based geometric prior constructed?
5.How is the atlas of priors integrated into the classification?
6.What are the drawbacks of using such an atlas of priors and how to deal with it?
7.What is the Markov random field model used in brain tissue classification?
8.How do we limit the overregularization of Markov random fields?

46 |
Leemput et al. |
Bibliography
[1]Santago, P. and Gage, H., Quantification of MR brain images by mixture density and partial volume modeling, IEEE Trans. Med. Imaging, Vol. 12, No. 3, pp. 566–574, 1993.
[2]Laidlaw, D. H., Fleischer, K. W., and Barr, A. H., Partial-volume Bayesian classification of material mixtures in MR volume data using voxel histograms, IEEE Trans. Med. Imaging, Vol. 17, No. 1, pp. 74–86, 1998.
[3]Choi, H. S., Haynor, D. R., and Kim, Y., Partial volume tissue classification of multichannel magnetic resonance images—A mixel model, IEEE Trans. Med. Imaging, Vol. 10, No. 3, pp. 395–407, 1991.
[4]Wells, W., III, Grimson, W., Kikinis, R., and Jolesz, F., Adaptive segmentation of MRI data, IEEE Trans. Med. Imaging, Vol. 15, No. 4, pp. 429–442, 1996.
[5]Held, K., Kops, E. R., Krause, B. J., Wells, W. M., III, Kikinis, R., and Muller¨-Gartner,¨ H. W., Markov random field segmentation of brain MR images, IEEE Trans. Med. Imaging, Vol. 16, No. 6, pp. 878–886, 1997.
[6]Guillemaud, R. and Brady, M., Estimating the bias field of MR Images, IEEE Trans. Med. Imaging, Vol. 16, No. 3, pp. 238–251, 1997.
[7]Kass, M., Witkin, A., and Terzopoulos, D., Snakes: Active contour models, Int. J. Comput Vision, Vol. 1, No. 4, pp. 321–331, 1988.
[8]McInerney, T. and Terzopoulos, D., Deformable models in medical image analysis: A survey, Med. Image Anal., Vol. 2, No. 1, pp. 1–36, 1996.
[9]Lotj¨onen,¨ J., Reissman, P.-J., Mangin, I., and Katila, T., Model extraction from magnetic resonance volume data using the deformable pyramid, Med. Image Anal., Vol. 3, No. 4, pp. 387–406, 1999.
[10]Zeng, X., Staib, L., Schultz, R., and Duncan, J., Segmentation and measurement of the cortex from 3D MR images using coupled surfaces propagation, IEEE Trans. Med. Imaging, Vol. 18, No. 10, pp. 927–937, 1999.
Model-Based Brain Tissue Classification |
47 |
[11]Gonzalez´ Ballester, M., Zisserman, A., and Brady, M., Segmentation and measurement of brain structures in MRI including confidence bounds, Med. Image Anal., Vol. 4, pp. 189–200, 2000.
[12]Xu, C., Pham, D., Rettmann, M., Yu, D., and Prince, J., Reconstruction of the human cerebral cortex from magnetic resonance images, IEEE Trans. Med. Imaging, Vol. 18, No. 6, pp. 467–480, 1999.
[13]Warfield, S., Kaus, M., Jolesz, F., and Kikinis, R., Adaptive, template moderated, spatially varying statistical classification, Med. Image Anal., Vol. 4, No. 1, pp. 43–55, 2000.
[14]Collins, D. L., Zijdenbos, A. P., Barr, W. F. C., and Evans, A. C., ANIMAL+INSECT: Improved cortical structure segmentation, In: Proceedings of the Annual Symposium on Information Processing in Medical Imaging, Kuba, A., Samal, M., and Todd-Pokropek, A., eds., Lecture Notes in Computer Science, Vol. 1613, Springer, Berlin, pp. 210–223, 1999.
[15]Liang, Z., MacFall, J. R., and Harrington, D. P., Parameter estimation and tissue segmentation from multispectral MR images, IEEE Trans. Med. Imaging, Vol. 13, No. 3, pp. 441–449, 1994.
[16]Schroeter, P., Vesin, J.-M., Langenberger, T., and Meuli, R., Robust parameter estimation of intensity distributions for brain magnetic resonance images, IEEE Trans. Med. Imaging, Vol. 17, No. 2, pp. 172–186, 1998.
[17]Wilson, D. and Noble, J., An adaptive segmentation algorithm for time- of-flight MRA data, IEEE Trans. Med. Imaging, Vol. 18, No. 10, pp. 938– 945, 1999.
[18]Dempster, A. P., Laird, N. M., and Rubin, D. B., Maximum likelihood from incomplete data via the EM algorithm, J. R. Stat. Soc., Vol. 39, pp. 1–38, 1977.
[19]Wu, Z., Chung, H.-W., and Wehrli, F., A Bayesian approach to subvoxel tissue classification in NMR microscopic images of trabecular bone, MRM, Vol. 31, pp. 302–308, 1994.
48 |
Leemput et al. |
[20]Evans, A., Collins, D., Mills, S., Brown, E., Kelly, R., and Peters, T., 3D statistical neuroanatomical models from 305 MRI volumes, In: Proceeding of the IEEE Nuclear Science Symposium and Medical Imaging Conference, pp. 1813–1817, 1993.
[21]Maes, F., Collignon, A., Vandermeulen, D., Marchal, G., and Suetens, P., Multi-modality image registration by maximization of mutual information, IEEE Trans. Med. Imaging, Vol. 16, No. 2, pp. 187–198, 1997.
[22]Maes, F., Vandermeulen, D., and Suetens, P., Medical image registration using mutual information, Proc. IEEE, Vol. 91, No. 10, pp. 1699–1722, 2003.
[23]Van Leemput, K., Maes, F., Vandermeulen, D., and Suetens, P., Automated model-based bias field correction of MR images of the brain, IEEE Trans. Med. Imaging, Vol. 18, No. 10, pp. 885–896, 1999.
[24]D’Agostino, E., Maes, F., Vandermeulen, D., and Suetens, P., A viscous fluid model for multimodal non-rigid image registration using mutual information, Med. Image Anal., Vol. 7, No. 4, pp. 565–575, 2003.
[25]Simmons, A., Tofts, P., Barker, G., and Arridge, S., Sources of intensity nonuniformity in spin echo images at 1.5 T, Magn. Reson. Med., Vol. 32, pp. 121–128, 1994.
[26]Sled, J. G. and Pike, G. B., Understanding Intensity Non-Uniformity in MRI, In: Proceedings of Medical Image Computing and ComputerAssisted Intervention, MICCAI’98, Lecture Notes in Computer Science, Vol. 1496, Springer, Berlin, pp. 614–622, 1998.
[27]Van Leemput, K., Maes, F., Vandermeulen, D., and Suetens, P., A unifying framework for partial volume segmentation of brain MR images, IEEE Trans. Med. Imaging, Vol. 22, No. 1, pp. 105–119, 2003.
[28]Tincher, M., Meyer, C., Gupta, R., and Williams, D., Polynomial modeling and reduction of RF body coil spatial inhomogeneity in MRI, IEEE Trans. Med. Imaging, Vol. 12, No. 2, pp. 361–365, 1993.
Model-Based Brain Tissue Classification |
49 |
[29]Moyher, S. E., Vigneron, D. B., and Nelson, S. J., Surface coil MR imaging of the human brain with an analytic reception profile correction, J. Magn. Reson. Imaging, Vol. 5, No. 2, pp. 139–144, 1995.
[30]Gonzalez´ Ballester, M. A., Morphometric Analysis of Brain Structures in MRI, Ph.D. Thesis, Department of Engineering Science, University of Oxford, 1999.
[31]Dawant, B. M., Zijdenbos, A. P., and Margolin, R., Correction of intensity variations in MR images for computer-aided tissue classification, IEEE Trans. Med. Imaging, Vol. 12, No. 4, pp. 770–781, 1993.
[32]Meyer, C., Bland, P., and Pipe, J., Retrospective correction of MRI amplitude inhomogeneities, In: Proceedings of the First International Conference on Computer Vision, Virtual Reality, and Robotics in Medicine, CVRMED’95, Ayache, N., ed., Lecture Notes in Computer Science, Vol. 905, Springer, Nice, France, pp. 513–522, 1995.
[33]Brechbuhler,¨ C., Gerig, G., and Szekely,´ G., Compensation of spatial inhomogeneity in MRI based on a parametric bias estimate, In: Proceedings of Visualization in Biomedical Computing, VBC’96, Lecture Notes in Computer Science, Vol. 1131, Springer, Berlin, pp. 141–146, 1996.
[34]Sled, J. G., Zijdenbos, A. P., and Evans, A. C., A comparison of retrospective intensity non-uniformity correction methods for MRI, In: Proceedings of XVth International Conference on Information Processing in Medical Imaging, IPMI’97, Lecture Notes in Computer Science, Vol. 1230, Springer, Berlin, pp. 459–464, 1997.
[35]Styner, M., Brechbuhler,¨ C., Szekely,´ G., and Gerig, G., Parametric estimate of intensity inhomogeneities applied to MRI, IEEE Trans. Med. Imaging, Vol. 19, No. 3, pp. 153–165, 2000.
[36]Sled, J. G., Zijdenbos, A. P., and Evans, A. C., A Nonparametric method for automatic correction of intensity nonuniformity in MRI Data, IEEE Trans. Med. Imaging, Vol. 17, No. 1, pp. 87–97, 1998.
[37]Mangin, J.-F., Entropy minimization for automatic correction of intensity nonuniformity, In: Proceedings of IEEE Workshop on Mathematical