
- •Contents
- •Foreword to the English translation
- •Preface
- •1 Introduction
- •1.1 Historical review
- •1.2 The birth of the concept of crystal growth
- •1.3 Morphology, perfection, and homogeneity
- •1.4 Complicated and complex systems
- •References
- •Suggested reading
- •2 Crystal forms
- •2.1 Morphology of crystals – the problems
- •References
- •Suggested reading
- •3 Crystal growth
- •3.1 Equilibrium thermodynamics versus kinetic thermodynamics
- •3.2 Driving force
- •3.3 Heat and mass transfer
- •3.4 Examples of mass transfer
- •3.6 Nucleation
- •3.7 Lattice defects
- •3.8 Interfaces
- •3.9 Spiral growth
- •3.10 Growth mechanism and morphology of crystals
- •3.11 Morphological instability
- •3.12 Driving force and morphology of crystals
- •3.13 Morphodroms
- •3.14 Element partitioning
- •3.15 Inclusions
- •References
- •Suggested reading
- •4 Factors determining the morphology of polyhedral crystals
- •4.1 Forms of polyhedral crystals
- •4.2 Structural form
- •4.3 Equilibrium form
- •4.4 Growth forms
- •4.4.1 Logical route for analysis
- •4.4.2 Anisotropy involved in the ambient phase
- •4.4.3 Whiskers
- •MAJOR FACTORS
- •METHODOLOGY
- •IMPURITIES
- •AMBIENT PHASES AND SOLVENT COMPONENTS
- •4.4.7 Factors controlling growth forms
- •References
- •Suggested reading
- •5 Surface microtopography of crystal faces
- •5.1 The three types of crystal faces
- •5.2 Methods of observation
- •5.3 Spiral steps
- •5.4 Circular and polygonal spirals
- •5.5 Interlaced patterns
- •5.6 Step separation
- •5.7 Formation of hollow cores
- •5.8 Composite spirals
- •5.9 Bunching
- •5.10 Etching
- •References
- •Suggested reading
- •6 Perfection and homogeneity of single crystals
- •6.1 Imperfections and inhomogeneities seen in single crystals
- •6.2 Formation of growth banding and growth sectors
- •6.3 Origin and spatial distribution of dislocations
- •References
- •7 Regular intergrowth of crystals
- •7.1 Regular intergrowth relations
- •7.2 Twinning
- •7.2.1 Types of twinning
- •7.2.2 Energetic considerations
- •7.2.4 Penetration twins and contact twins
- •7.2.5 Transformation twin
- •7.2.6 Secondary twins
- •7.3 Parallel growth and other intergrowth
- •7.4 Epitaxy
- •7.5 Exsolution, precipitation, and spinodal decomposition
- •References
- •Suggested reading
- •8 Forms and textures of polycrystalline aggregates
- •8.1 Geometrical selection
- •8.2 Formation of banding
- •8.3 Spherulites
- •8.4 Framboidal polycrystalline aggregation
- •References
- •Suggested reading
- •9 Diamond
- •9.1 Structure, properties, and use
- •9.2 Growth versus dissolution
- •9.3 Single crystals and polycrystals
- •9.4 Morphology of single crystals
- •9.4.1 Structural form
- •9.4.2 Characteristics of {111}, {110}, and {100} faces
- •9.4.3 Textures seen inside a single crystal
- •9.4.4 Different solvents (synthetic diamond)
- •9.4.5 Twins
- •9.4.6 Coated diamond and cuboid form
- •9.4.7 Origin of seed crystals
- •9.4.8 Type II crystals showing irregular forms
- •References
- •Suggested reading
- •10 Rock-crystal (quartz)
- •10.1 Silica minerals
- •10.2 Structural form
- •10.3 Growth forms
- •10.4 Striated faces
- •10.5 Growth forms of single crystals
- •10.5.1 Seed crystals and forms
- •10.5.2 Effect of impurities
- •10.5.3 Tapered crystals
- •10.6 Twins
- •10.6.1 Types of twins
- •10.6.2 Japanese twins
- •10.6.3 Brazil twins
- •10.7 Scepter quartz
- •10.8 Thin platy crystals and curved crystals
- •10.9 Agate
- •References
- •11 Pyrite and calcite
- •11.1 Pyrite
- •11.1.2 Characteristics of surface microtopographs
- •11.1.4 Polycrystalline aggregates
- •11.2 Calcite
- •11.2.1 Habitus
- •11.2.2 Surface microtopography
- •References
- •12 Minerals formed by vapor growth
- •12.1 Crystal growth in pegmatite
- •12.3 Hematite and phlogopite in druses of volcanic rocks
- •References
- •13 Crystals formed by metasomatism and metamorphism
- •13.1 Kaolin group minerals formed by hydrothermal replacement (metasomatism)
- •13.2 Trapiche emerald and trapiche ruby
- •13.3 Muscovite formed by regional metamorphism
- •References
- •14 Crystals formed through biological activity
- •14.1 Crystal growth in living bodies
- •14.2 Inorganic crystals formed as indispensable components in biological activity
- •14.2.1 Hydroxyapatite
- •14.2.2 Polymorphic minerals of CaCO3
- •14.2.3 Magnetite
- •14.3 Crystals formed through excretion processes
- •14.4 Crystals acting as possible reservoirs for necessary components
- •14.5 Crystals whose functions are still unknown
- •References
- •Appendixes
- •A.1 Setting of crystallographic axes
- •A.2 The fourteen Bravais lattices and seven crystal systems
- •A.3 Indexing of crystal faces and zones
- •A.4 Symmetry elements and their symbols
- •Materials index
- •Subject index
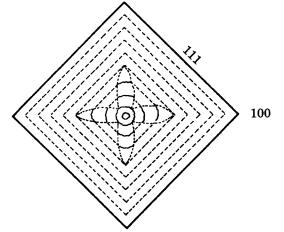
9.4 Morphology of single crystals 183
Figure 9.12. Growth sector showing a center-cross pattern formed by the growth of
smooth {111} and rough {100} interfaces.
growth, and the crystal eventually takes a simple octahedral form. Whereas the growth banding in the {111} growth sectors is straight and parallel to {111}, that in the {100} growth sectors is wavy and curved, like a hammock. This indicates that on the same crystal {111} behaves as a smooth interface (F face), whereas {100} behaves as a rough interface (K face). In natural diamond crystals, {100} behaves exclusively as a rough interface, and no evidence has been obtained so far to show that {100} may sometimes behave as a smooth interface.
From these observations, we may conclude that, in the growth of natural diamond crystals, three faces, {111}, {110}, and {100}, behave and show characteristics completely in agreement with the characteristics expected from PBC analysis. Therefore, we may conclude that, under the environmental conditions of natural diamond growth (principally in the silicate solution phase), {111} always behaves as a smooth interface under /kT * conditions, whereas the /kT ** of {110}, and particularly of {100}, stays close to the origin under any conditions, and these faces behave exclusively as rough interfaces.
Figure 9.13 shows morphological changes of diamond crystals on a diagram of growth rate versus driving force relation (see Fig. 3.15), based on the relation of the ** positions of the {111} and {100} faces. From this, it is expected that spherulitic forms and cuboids occur under higher driving force conditions, whereas octahedral crystals are expected under lower driving force conditions.
9.4.4Different solvents (synthetic diamond)
In the case of synthetic diamond, grown under high-temperature, highpressure conditions from a high-temperature solution with metal or alloy as the solvent, diamond crystals exhibit a cubo-octahedral Tracht bounded by {100} and
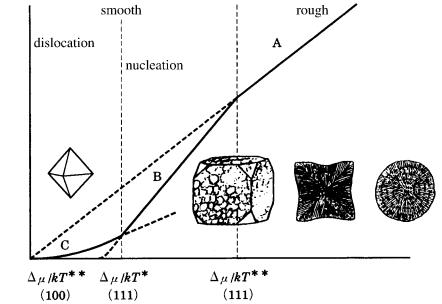
184 Diamond
growth rate R
driving force
Figure 9.13. Morphology of natural diamond crystals expected in relation to the driving force. The morphological change is as expected assuming that the positions of
/kT * and /kT ** are different for {111} and {100}.
{111}. Although the development of these two faces may vary depending on the driving force, both show evidence of spiral growth, as can be seen in Fig. 9.14. This indicates that {100} transforms from a rough K face to a smooth F face in a metallic solution. A transformation from a K face to an F face requires a change in the surface structure of the {100} face (i.e. surface reconstruction should take place), resulting in the same effect as introducing a new PBC. In the growth of natural diamond in a high-temperature solution phase with silicate as the solvent, surface reconstruction does not occur, whereas it does occur if a metal solvent is used. Surface reconstruction occurs in a solution in which the metallic element has a small ionic radius as the solvent, whereas it does not occur in a solvent with large ionic radius.
Recently, diamond synthesis has been successfully performed under hightemperature, high-pressure conditions in a system using kimberlite powder, various carbonates, sulphates or water as the solvent [13], [14]. Higher pressure and temperature conditions are required in a non-metallic solution than in a metallic solution, and the crystals obtained are mainly simple octahedral, differing from those observed in crystals grown from metallic solutions. Crystals synthesized in a non-metallic solution show the same characteristics as natural diamond Tracht. These observations indicate that the solvent components have a definitive effect upon surface reconstruction, and thus on the morphology of the crystals.
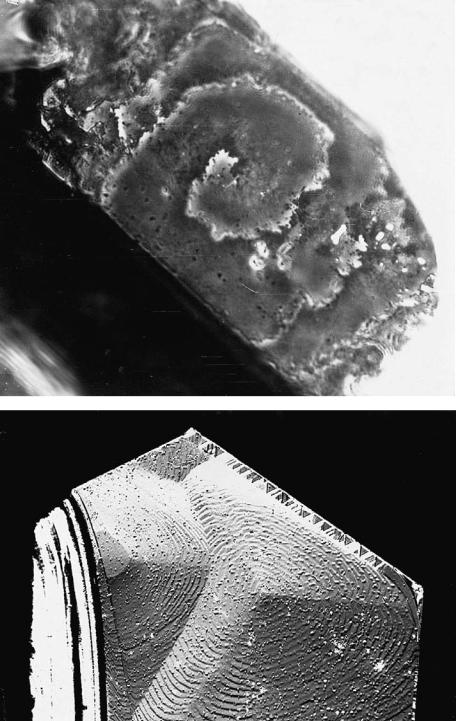
9.4 Morphology of single crystals 185
(a)
(b)
Figure 9.14. Spiral growth layers observed on (a) {100} and (b) {111} faces of diamond
crystals synthesized under high-pressure and high-temperature conditions.
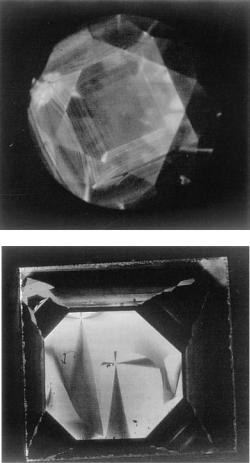
186 Diamond
(a)
(b)
Figure 9.15. Comparison of cathodoluminescence tomographs of (a) natural and
(b) synthetic diamonds. (By courtesy of All Japan Gemmological Association [15].)
The resulting differences in the morphology of natural and synthetic diamond single crystals using metal solvents is also recorded in patterns shown by growth sectors and growth banding. If we reveal inhomogeneities of this sort using such techniques as cathodoluminescence, the differences between natural and synthetic diamonds are easily distinguishable. Figure 9.15 is an example of cathodoluminescence photographs of natural and synthetic diamonds grown in metallic solution, and the difference between the two is very evident [15]. It is noted that the intensity of the cathodoluminescence is remarkably different depending on growth sector. Figure 9.15 also indicates that element partitioning is affected by crystallographic directions, and therefore by kinetics.
Vapor grown diamonds are synthesized by the CVD method under low-pressure conditions, for which diamond becomes unstable, and it is possible to obtain single crystals of micrometer size. Frequent occurrences of multiply twinned par-