
- •Contents
- •Foreword to the English translation
- •Preface
- •1 Introduction
- •1.1 Historical review
- •1.2 The birth of the concept of crystal growth
- •1.3 Morphology, perfection, and homogeneity
- •1.4 Complicated and complex systems
- •References
- •Suggested reading
- •2 Crystal forms
- •2.1 Morphology of crystals – the problems
- •References
- •Suggested reading
- •3 Crystal growth
- •3.1 Equilibrium thermodynamics versus kinetic thermodynamics
- •3.2 Driving force
- •3.3 Heat and mass transfer
- •3.4 Examples of mass transfer
- •3.6 Nucleation
- •3.7 Lattice defects
- •3.8 Interfaces
- •3.9 Spiral growth
- •3.10 Growth mechanism and morphology of crystals
- •3.11 Morphological instability
- •3.12 Driving force and morphology of crystals
- •3.13 Morphodroms
- •3.14 Element partitioning
- •3.15 Inclusions
- •References
- •Suggested reading
- •4 Factors determining the morphology of polyhedral crystals
- •4.1 Forms of polyhedral crystals
- •4.2 Structural form
- •4.3 Equilibrium form
- •4.4 Growth forms
- •4.4.1 Logical route for analysis
- •4.4.2 Anisotropy involved in the ambient phase
- •4.4.3 Whiskers
- •MAJOR FACTORS
- •METHODOLOGY
- •IMPURITIES
- •AMBIENT PHASES AND SOLVENT COMPONENTS
- •4.4.7 Factors controlling growth forms
- •References
- •Suggested reading
- •5 Surface microtopography of crystal faces
- •5.1 The three types of crystal faces
- •5.2 Methods of observation
- •5.3 Spiral steps
- •5.4 Circular and polygonal spirals
- •5.5 Interlaced patterns
- •5.6 Step separation
- •5.7 Formation of hollow cores
- •5.8 Composite spirals
- •5.9 Bunching
- •5.10 Etching
- •References
- •Suggested reading
- •6 Perfection and homogeneity of single crystals
- •6.1 Imperfections and inhomogeneities seen in single crystals
- •6.2 Formation of growth banding and growth sectors
- •6.3 Origin and spatial distribution of dislocations
- •References
- •7 Regular intergrowth of crystals
- •7.1 Regular intergrowth relations
- •7.2 Twinning
- •7.2.1 Types of twinning
- •7.2.2 Energetic considerations
- •7.2.4 Penetration twins and contact twins
- •7.2.5 Transformation twin
- •7.2.6 Secondary twins
- •7.3 Parallel growth and other intergrowth
- •7.4 Epitaxy
- •7.5 Exsolution, precipitation, and spinodal decomposition
- •References
- •Suggested reading
- •8 Forms and textures of polycrystalline aggregates
- •8.1 Geometrical selection
- •8.2 Formation of banding
- •8.3 Spherulites
- •8.4 Framboidal polycrystalline aggregation
- •References
- •Suggested reading
- •9 Diamond
- •9.1 Structure, properties, and use
- •9.2 Growth versus dissolution
- •9.3 Single crystals and polycrystals
- •9.4 Morphology of single crystals
- •9.4.1 Structural form
- •9.4.2 Characteristics of {111}, {110}, and {100} faces
- •9.4.3 Textures seen inside a single crystal
- •9.4.4 Different solvents (synthetic diamond)
- •9.4.5 Twins
- •9.4.6 Coated diamond and cuboid form
- •9.4.7 Origin of seed crystals
- •9.4.8 Type II crystals showing irregular forms
- •References
- •Suggested reading
- •10 Rock-crystal (quartz)
- •10.1 Silica minerals
- •10.2 Structural form
- •10.3 Growth forms
- •10.4 Striated faces
- •10.5 Growth forms of single crystals
- •10.5.1 Seed crystals and forms
- •10.5.2 Effect of impurities
- •10.5.3 Tapered crystals
- •10.6 Twins
- •10.6.1 Types of twins
- •10.6.2 Japanese twins
- •10.6.3 Brazil twins
- •10.7 Scepter quartz
- •10.8 Thin platy crystals and curved crystals
- •10.9 Agate
- •References
- •11 Pyrite and calcite
- •11.1 Pyrite
- •11.1.2 Characteristics of surface microtopographs
- •11.1.4 Polycrystalline aggregates
- •11.2 Calcite
- •11.2.1 Habitus
- •11.2.2 Surface microtopography
- •References
- •12 Minerals formed by vapor growth
- •12.1 Crystal growth in pegmatite
- •12.3 Hematite and phlogopite in druses of volcanic rocks
- •References
- •13 Crystals formed by metasomatism and metamorphism
- •13.1 Kaolin group minerals formed by hydrothermal replacement (metasomatism)
- •13.2 Trapiche emerald and trapiche ruby
- •13.3 Muscovite formed by regional metamorphism
- •References
- •14 Crystals formed through biological activity
- •14.1 Crystal growth in living bodies
- •14.2 Inorganic crystals formed as indispensable components in biological activity
- •14.2.1 Hydroxyapatite
- •14.2.2 Polymorphic minerals of CaCO3
- •14.2.3 Magnetite
- •14.3 Crystals formed through excretion processes
- •14.4 Crystals acting as possible reservoirs for necessary components
- •14.5 Crystals whose functions are still unknown
- •References
- •Appendixes
- •A.1 Setting of crystallographic axes
- •A.2 The fourteen Bravais lattices and seven crystal systems
- •A.3 Indexing of crystal faces and zones
- •A.4 Symmetry elements and their symbols
- •Materials index
- •Subject index
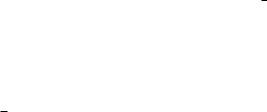
4.4 Growth forms 81
determine the dog-tooth Habitus, only striations parallel to the edges with {1011} faces are observable; step patterns due to the spreading of growth layers on the face have never been observed.
The structural form of brookite (TiO2) is expected to be bounded by {210} and {111}, both being F faces by PBC analysis, but the actual growth form observed is platy Habitus bounded by largely developed {100}, which is an S face. The misfit ratio between the PBC on the (0110) face of quartz and that on (100) of brookite is the smallest among any misfit ratios between the two crystal species. From this, it was found that the platy Habitus of brookite arose because quartz adsorbed in an epitaxial relation on {100} of brookite, thus diminishing the growth rate R of (100) [30].
In addition to the cases discussed above, in which the addition of impurities decreases the growth rate, there are also cases in which the addition of impurities accelerates the growth rate. As already described in Section 4.4.3, where SnO2 or TiO2 crystals are synthesized in the high-temperature solution phase, if trivalent ions, such as Fe3 , having a similar ionic radius to those of Sn4 and Ti4 are added, an extremely elongated prismatic or whisker morphology results. This is because {111} (corresponding to an S face by PBC analysis) transforms into a K face by the addition of the impurity ions. This is because the transformation from an S face to a K face occurs by breaking bonds in PBCs to maintain the electronic neutrality through the substitution by impurity ions with a different charge [19]. On the other hand, bonds in PBCs on {110}, an F face, will not be broken by this substitution, and the face behaves as an F face. However, the adsorption of impurity ions affects the advancing rate of growth steps on {110}, which diminishes. Thus, the same impurity ions can have opposing effects upon the growth rate.
Since the most drastic change in Habitus and Tracht is effected by the addition of impurities, the relationship between impurity addition and change in Tracht may be applied to determine the absolute structure of a crystal, such as the determination of the positive or negative axis of a polar crystal, or the right-handed or lefthanded structure of an enantiomorphic crystal. Unless applying special techniques, it is not easy to determine the polarity of a crystal by the X-ray diffraction method. Generally, the axial direction is determined using either piezoelectricity or pyroelectricity. A method called tailor-made habit control has been designed to determine the absolute structure of a crystal, by observing the Habitus change in the presence of appropriate impurities which fit either direction of a polar axis [31].
AMBIENT PHASES AND SOLVENT COMPONENTS
It is generally observed that crystals from the same species exhibit widely different Tracht and Habitus depending on whether they grow from the vapor or solution phases.
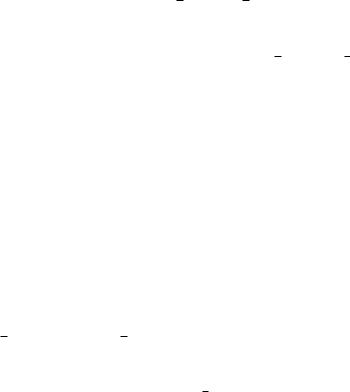
82 Morphology of polyhedral crystals
Hematite (Fe2O3) crystals grow around volcanic fumaroles that formed at the most recent stage of volcanic activity. This natural process corresponds to the growth of hematite by the chemical vapor transport (CVT) method, and the crystals of this form from all over the world show a thin platy Habitus with a largely developed {0001} face, bounded by narrow {1011} and {1010} faces. In contrast to this
Habitus, hematite crystals occurring in vein type or contact metasomatic ore deposits, which grew from a hydrothermal solution, characteristically show thick platy or nail-head Habitus bounded by well developed {1011} and {1010} faces. Corundum (Al2O3) crystals having the same crystal structure as hematite exhibit similar tendencies. Thin platy crystals grow on the wall of a vessel from vaporized phase by CVT, when crystals are synthesized by flux (high-temperature solution) method, using fluoride having high vapor pressure as flux. In contrast, corundum crystals grown in solution take on a thick platy Habitus. Natural corundum crystals formed by contact metasomatism or regional metamorphism characteristically show thick platy to prismatic Habitus, rather than a thin platy one. The big difference in Habitus observed between crystals grown from vapor and solution phases is related to the difference observed in the step separation of spiral growth layers between the two phases, which will be described in Section 5.6. Step separation observed in crystals grown from the vapor phase is up to 100 times wider than that observed in solution grown crystals.
High-temperature quartz crystals occur as phenocrysts in acidic igneous rocks with a high SiO2 content, and show hexagonal bipyramidal Habitus bounded by
only a {1011} face, with no {1010}; this Habitus has been accepted as the typical
Habitus of high-temperature quartz. However, when quartz crystals are synthesized above the transition temperature 573 °C in a hydrothermal solution, the crystals exhibit a hexagonal prismatic Habitus with {1010} associated faces. The hexagonal bipyramidal Habitus simply represents a Habitus of high-temperature quartz grown in an ambient phase of acidic silicate solution.
As will be described in Chapter 9, natural diamond crystals exhibit the same
Habitus as predicted from the structure form, on which the {111} faces always behave as smooth interfaces, whereas the {100} faces always behave as rough interfaces. Diamond crystals synthesized under high-temperature, high-pressure conditions from the solution phase, with metals or alloys as the solvent component, take cubo-octahedral Habitus. Although CVD diamonds grown from the vapor phase also take cubo-octahedral Habitus, the order of morphological importance of {111} and {100} is reversed as compared with that of diamond crystals grown under the diamond stable region, i.e. high-temperature, high-pressure conditions, in both natural and synthetic crystals (see Chapter 9).
The above examples describe the Habitus changes due to the difference between the vapor and solution phases, and between solvent components in solution
4.4 Growth forms 83
growth. Many examples are described in refs. [24] and [27], which consider different Habitus of the same crystal species grown from aqueous and organic solutions. Since controlling the morphology of tiny crystals is an important aspect in utilizing the numerous tiny crystals, how to control the Habitus of these crystals is still an important aspect in industry even today.
Even if crystals grow from the same aqueous solution, there are differences in
Habitus. NaClO3 crystals, for example, grow easily as polyhedral crystals, whereas NH4Cl crystals always grow as dendrites, and NaCl crystals appear as hopper crystals. If Pb or Mn ions are added, cubic crystals of NaCl bounded by flat {100} faces may be obtained quite easily, but if NaCl is grown in pure solution all crystals take a hopper form, unless great care is taken to keep the supersaturation very low. These differences occur because the solute–solvent interaction energies, and, as a result, the values of /kT * and /kT **, are different for different crystals.
The reasons why we have Habitus variation for vapor and solution phases, and for different solvents when the crystals grow from the solution phase, can therefore be understood in terms of the G factor of steps because of the different solute–solvent interaction energies.
TEMPER ATURE, PRESSURE, AND DRIVING FORCE
As discussed in Chapter 3, as the temperature or driving force increases a smooth interface transforms into a rough interface. When a crystal is bounded by many faces with different orders of morphological importance, it can be seen that a face with a lower order of morphological importance will show a roughening transition at a lower temperature and driving force than does a face with higher order of morphological importance. As a result, it is expected that changes in
Habitus and Tracht accompanying changes of temperature and driving force will be observed. Pressure will also affect the Tracht and Habitus, owing to its relation with the driving force, and the degree of impurity concentration will also show a temperature dependence. Changes of this type are seen not only in changes from polyhedral to hopper to dendritic shown in Fig. 3.21, but also changes in Habitus and
Tracht of polyhedral crystals.
TR ACHT CHANGES ASSOCIATED WITH GROWTH
Figure 4.13 shows statistical results on the frequency of appearance of different Tracht of a few hundred pyrite crystals occurring in approximately a handful of clay, depending on crystal size. The pyrite crystals were formed together with clay minerals by the reaction of hydrothermal solution with rocks near the sea bottom, and so can be regarded as being crystallized under nearly identical conditions of temperature, pressure, and driving force. Figure 4.13 indicates that smaller crystals show a higher frequency of appearance of simple cubic form bounded by
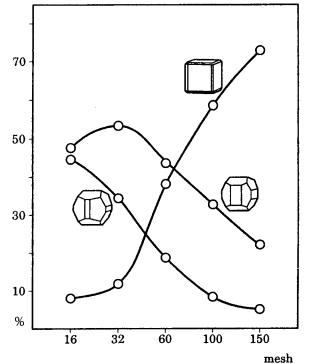
84 Morphology of polyhedral crystals
Figure 4.13. Frequency of appearance of different Tracht depending on crystal size. Statistical measurements were made on a few hundred pyrite crystals occurring in approximately a handful of clay. The mesh axis denotes the number of openings in a unit area of the mesh filter. See ref. [2], Chapter 2.
only {100} faces, and, as the grain size increases, that the frequency of appearance of pentagonal dodecahedral form mainly bounded by {210} faces increases. This implies that the Tracht changes from cubic to pentagonal dodecahedral as the crystals grow larger. This change in Tracht associated with growth is represented as striations on pentagonal dodecahedral faces. Figure 4.14 indicates that pentagonal dodecahedral faces appear by the piling up of steps of rectangular growth layers on {100} faces as the grain sizes increase. Pentagonal dodecahedral faces are characterized by the development of striations parallel to the edge with a {100} face, and also consists of not only {210} but also pentagonal dodecahedral faces such as {430}, {540}, etc. The most well developed {210} face is characterized by striations only, and does not show growth layers parallel to the face, as can be seen in Fig. 11.2. In contrast, there are cases in which {210} faces exhibit striations perpendicular to the edge; for example, in pyrite crystals from different modes of formation such as those from Elba. In this case, striations appear due to freely developed elongated growth layers on the {210} face. The same {210} faces grow differently depending
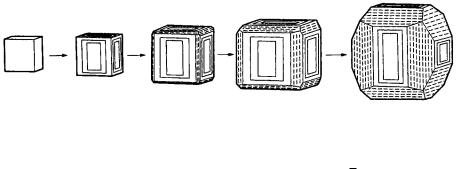
4.4 Growth forms 85
Figure 4.14. Changes observed on Tracht and surface microtopographs of {100} and
{210} faces of pyrite crystals as growth proceeds (see ref. [1], Chapter 2).
on growth conditions. Similar changes are noticed on {1010} faces between natural and synthetic quartz crystals.
Similar observations of Tracht variation as growth proceeds were also made on garnet crystals in regional metamorphic rocks (a {110} Tracht at the earlier stage changes to {211} as the crystals grow in size) [32]. Smaller crystals show a dodecahedral Tracht bounded by {110}, and as sizes increase by growth the Tracht changes to tetragonal trioctahedron bounded by {211}. In contrast to the fact that growth layers of rhombic form are universally observed on {110} faces, {211} shows only striations parallel to the edges with {110}.
4.4.6Ultra-fine particles
The discussions summarized in Sections 4.4.2–4.4.5 relate to the morphologies of crystals of size down to a few millimeters. Will extremely tiny crystals of sizes ranging from a few to 100 nm take polyhedral forms bounded by flat faces, or will they show forms bounded by curved faces due to the size effect? It was theoretically predicted that, as the size of solid materials decreases, a change is expected to occur in the physical properties. Also, considering the presence of ultra-fine particles in the cosmos, the morphology exhibited by ultra-fine particles attracts a special concern.
Investigations into ultra-fine particles may be regarded as a Japanese specialty. In Japan, there is a fifty-year-long history of investigation on the forms and structures of ultra-fine particles of metals and chemical compounds using gas evaporation techniques, electron microscopy, and selected area electron diffraction; the results are summarized in ref. [33]. In this reference, the Habitus and Tracht exhibited by single crystals, twinned, and multiply twinned particles are summarized in relation to the growth conditions on hcp metals (Mg, Zn, Cd, Be), bcc metals (Fe, V, Nb, Ta, Cr, Mo, W), group IVB elements (C, Si, Ge, Sn), and Sb and Bi. Surprisingly, ultrafine particles of nanometer size show a polyhedral Habitus bounded by flat faces in most cases, and their Habitus and Tracht change depending on growth conditions, just as in the case of larger crystals. However, there are still many unsolved problems in understanding how these Habitus and Tracht changes occur. As compared to