
Крючков Фундаменталс оф Нуцлеар Материалс Пхысицал Протецтион 2011
.pdf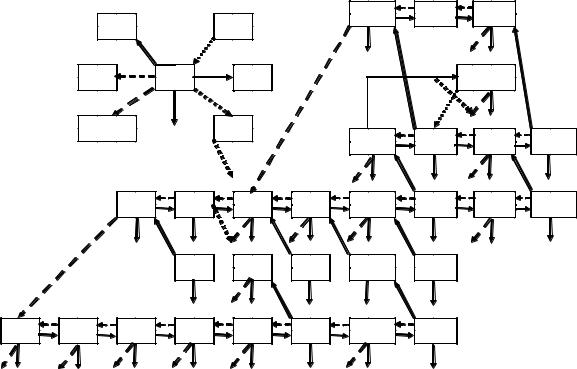
|
AZ+1 |
|
Am Z |
|
242Cm |
243Cm |
244Cm |
|
||
|
γ |
|
|
|
|
|
||||
|
|
β- |
|
|
|
|
|
|
|
|
|
A-1 |
σn,2 A |
σa |
A+1 |
|
|
|
242m |
|
|
|
Z |
α |
Z |
β+ |
Z |
|
|
|
Am |
|
|
|
|
|
|
|
|||||
|
A-4 |
|
σf |
A |
|
|
|
|
|
|
|
Z-2 |
|
|
Z-1 |
|
241Am |
242Am |
243Am |
244Am |
|
|
|
|
|
|
|
|
||||
|
|
236Pu |
237Pu |
|
238Pu |
239Pu |
240Pu |
241Pu |
242Pu |
243Pu |
|
|
|
236Np |
237Np |
238Np |
239Np |
240Np |
|
|
|
232U |
233U |
234U |
235U |
|
236U |
237U |
238U |
239U |
|
|
Fig. 5.3. Isotopic transformations in a uranium-plutonium cycle
231
Determination of NM content by measurements of intrinsic gamma radiations
Factors influencing NDA measurement results
NM solutions are normally homogeneous and one can simply get a standard for measurements using a suitable vessel. The content of NM in the sample is found from the intensity of its emission from the sample. Some of the gamma radiation is however absorbed inside the sample en route to the detector. The correction factor CF(АТ) that accounts for the self-absorption of NM radiation inside the sample can be found using an external gamma radiation source with an energy close to the NM radiation energy. To do this, the transmission T of the external source gamma ray beam through the sample is measured:
T = |
N |
= exp(- μl × x) , |
(5.1) |
|
|||
|
N0 |
|
where N0 is the number of the source-emitted gamma-quanta getting on the sample; N is the number of the source-emitted gamma-quanta transmitted through the sample; μl is the gamma-ray linear attenuation factor; and х is the sample thickness. With a plane geometry, the allowance is calculated from the formula:
CF ( AT ) = |
μl × x |
= |
- ln(T ) |
|
|
(1-T ) . |
(5.2) |
||
[1 - exp(-μl × x)] |
An extra source attached next to the detector is used to correct the counting losses caused by the measurement system’s dead time. Using a radiation source simplifies the correction circuit and makes it more reliable as compared to instrumented correction based on a pulse generator. Observations of the pulse count rate in the peak produced by this source yield data on the count losses during measurements with samples. Such source is selected such that not to hamper measurements of the NM emission from the samples.
Uranium solutions are tested via passive measurement of gamma rays with the energy of 185.7 keV of 235U, the results being corrected from the measurement results for transmission of the 75Se source radiation with the
232
energy 136.0 keV and the counting losses corrected using a (the radiation energy of 88 keV).
The range of the concentrations measured covers seven orders of magnitude. The sample dimensions are smaller than in other methods.
Waste control. Segmented gamma ray scanning
This technique is used to test containers and tanks with solid and liquid NM-containing waste. The measurement conditions in this are rather complicated: NM is present in small concentrations and has a nonuniform axial and radial distribution in the container. The volumes of the samples controlled differ greatly ranging from small vials to 200-liter metal drums. The matrix density is relatively low.
Onsite NM-containing waste is containerized in layers, NM in these being more uniform horizontally than vertically. When analyzed, the sample is rotated to mitigate the horizontal nonuniformity effects, as the vertical nonuniformity effects are mitigated by analyzing material by segments. Each segment is measured individually with all the values obtained summed up. The basic assumption is that NM is uniformly distributed inside each segment and one can determine to which extent the gamma radiations inside the segment are mitigated by measurements of the transmission.
Segmented scanning (SS) combines container movements with radiation measurements. SS is probably the most widespread ND measurement technique based on γ-radiation measurements.
Analyzed radiations
Uranium content is controlled by measuring γ-radiation of 185.7 keV. For 239Pu control, one normally measures γ-radiation of 413.7 keV.
The obtained results distorted by the absorption of γ-rays in the container are corrected with the aid of the correction factors found by measuring the transmission of the 75Se source γ-radiations with the energies of 136.0 keV, 264.6 keV, 279.5 keV and 400.6 keV through the container. The correction for the results of measuring the 185.7-keV radiation of 235U is obtained by interpolation between the transmission values for gamma ray lines with energies of 136.0 keV and 264.6 keV, while the correction for measurements of 413.7-keV 239Pu radiations is found by extrapolation.
Another source, 109Cd, (Eγ = 88.0 keV), serves to correct the pulse count errors.
233
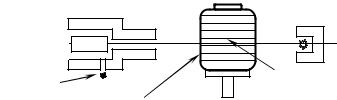
Measurements and interpretation of results
To have the result to characterize the average NM content in a container, the latter is rotated and moved vertically. The container is lifted gradually as its vertical segments are scanned, this making it possible to average the difference in attenuations of radiations in separate horizontal segments. The facility is shown schematically in Fig. 5.4.
The pulse count rate in the complete nр absorption peak is found by measurements using the formula:
n р |
= (n'р |
- RB ) × |
Rref |
, |
(5.3) |
|
|||||
|
|
|
RRL |
|
where n'р is the measured count rate in the interval that contains the desired
peak; (Rref /RRL) is the allowance for the counting loss found by measurements with 109Cd without and with a sample; and RB is the background count rate below the peak.
|
Shielding and |
|
|
lead collimator |
75Se source in |
|
|
shielding |
Detector |
G |
|
109Cd source |
|
|
|
Container with |
Rotating and |
|
NM in matrix |
lifting table |
Fig. 5.4. Schematic of a segmented scanning facility
The measured and corrected pulse count rate in the gamma-peak nр relates to the mass of the isotope determined via the calibration factor which is found with the aid of a standard. The contribution of the calibration factor to the systematic and random measurement error should be relatively small.
The results of measurements using a standard may be influenced by several factors, including homogeneity of the sample material, the
234
magnitude of transmission (should be over 10%), size of NM particles and others.
Segmented scanning is applicable to many low-density NM-containing materials: paper, sand, plastic, ash and liquids.
Control of deposits
Deposit is NM that remains inside process equipment and cannot be removed by way of conventional flushing. It settles in tanks and accumulates in process piping and ventilation systems. The deposit amount varies from 0.1 to 0.2% of the total facility capacity even after the equipment is cleaned thoroughly. The initial operation phase of a new facility may account for a deposit share of 1 to 10% of the output product.
Most of the uranium and plutonium deposit measurements are based on recording the 235U peak of 185.7 keV and the combination of 239Pu peaks with the energies of 375 keV and 414 keV. Portable scintillation NaI detectors are commonly used to measure these gamma-quanta. For measurements, the detectors have a lead shielding made around them, the latter having a collimator aperture to transmit radiation only from the desired direction.
A deposit mass measurement program has the following stages.
1.Identification of potential deposit points in equipment.
2.Rapid surveying using collimated instrumentation to detect locations with the largest NM deposit quantities.
3.Graduation of the detectors using standard samples. Each detector is graduated for the deposit location as a point, a line or a plane.
4.Selection of the model for deposit in each equipment component. Deposits are characterized as a point, a line or a plane, and are quantitatively measured.
5.Quantitative measurements. Most of the time is given to locations with most of the NM amount.
6.Deposits are measured from different directions and at various distances, using different geometry description models, to estimate the measurement result uncertainty. Corrections for the radiation attenuation thanks to the self-absorption in deposit and the absorption en route to the detector are estimated and introduced.
Graduation procedure
Graduation for a point, a line or a plane can be done using one movable point source of 1 to 5 g of 235U or 239Pu. It should be remembered that self-
235

absorption of gamma-quanta in uranium or plutonium may be highly intensive.
If a standard point source contains т0 grams of NM, the mass of the point deposit m (g) is found from the formula:
m = m0 |
× |
C |
× |
r |
2 |
, |
(5.4) |
|
|
|
|
||||||
C |
r |
2 |
||||||
|
|
|
|
|
||||
|
|
0 |
|
0 |
|
|
where С is the count rate in the deposit measurement, and r is the distance from the detector to the deposit.
Where there is a linear deposit distribution, the NM mass per the deposit length unit mL (g/m) is found from the expression:
mL |
= |
m0 |
× |
C |
× |
r |
. |
(5.5) |
LE |
|
|
||||||
|
|
|
C0 r0 |
|
If a planar deposit distribution model is chosen, the NM mass per the deposit area unit mA (g/m2) is found from the formula:
mA |
= |
m0 |
× |
C |
. |
(5.6) |
AE |
|
|||||
|
|
|
C0 |
|
Gamma ray spectrometry of uranium enrichment
There are two definitions of the uranium enrichment by 235U isotope:
·enrichment (mass percent) Е1=(mass of 235U/total mass of U) 100%;
·enrichment (atomic percent) Е2=(number of 235U atoms/total number of U atoms)×100%.
Commonly used are analyses of uranium enrichment based on the assumption that the intensity of gamma radiation of 235U from uranium
samples of a sufficient thickness is proportional to the enrichment thereof by 235U. Gamma-quanta with the energy of 185.7 keV in 235U decays are
emitted with a probability of (57.5±0.9)% (the quantum yield of radiation*, the number of quanta of the said radiation reaches 4.6×104 quantum/(s×g)).
* “Branching ratio” is the term often used in foreig n literature.
236
Mean free paths and “infinite“ thicknesses for quan ta of 185.7 keV in uranium compounds are given in Table 5.4.
Table 5.4
Mean free paths and “infinite” thicknesses for gamm a radiation of 185.7 keV in uranium compounds
|
|
|
Density, |
|
Infinite |
|
No. |
Compound |
Mean free path, cm |
thickness, |
|||
g/cm3 |
||||||
|
|
|
|
|
cm |
|
1 |
Metal |
18.7 |
0.04 |
0.26 |
||
2 |
UF6 (solid) |
4.7 |
0.20 |
1.43 |
||
3 |
UO2 |
(caked) |
10.9 |
0.07 |
0.49 |
|
4 |
UO2 |
(powder) |
2.0 |
0.39 |
2.75 |
|
5 |
Uranyl nitrate |
2.8 |
0.43 |
3.04 |
Method description
The detector (Fig. 5.5) records the radiation transmitted through the filter and the collimator. The detector-visible surface area is determined with the aid of a collimator. The filter absorbs radiation in the region of the energies below 185.7 keV, which enables the measuring path to be unloaded and the share of 185.7-keV signals in the total flow of signals via the path to be increased. The filters are made of average-weight materials (Cd, Ni and others).
The pulse count rate in the photopeak nр=Sph/t, where Sph is the count of pulses in the photopeak and t is the measurement time, is determined by the following expression:
nр = (Ωd / 4π )ελ235 (N A / AU ) ρU EIθ exp(− µf ρf df ) ×
D |
(5.7) |
|
× exp(− µc ρc dc )∫exp(−μℓ x)dx, |
||
|
||
0 |
|
where Ωd is the solid angle limited by the collimator aperture; ε is the efficiency of the detector at Еγ =185.7 keV; NA is the Avogadro number; АU is the atomic mass of uranium in the sample; ρU is the density of the uranium in the sample; Е is the enrichment; I is the quantum yield (branching ratio) for the irradiation of 185.7 keV; θ is the collimator aperture area; μf, ρf, df are the filter mass attenuation factor, density and
237
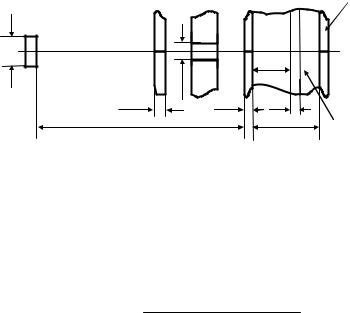
thickness; μc, ρc, dc are the container mass attenuation factor, density and thickness; μℓ is the attenuation factor for the gamma radiation in the uranium sample; and λ235 is the 235U decay constant.
Filter |
Collimator |
|
Container |
Detector |
|
|
|
2r |
|
x |
|
|
|
|
|
|
r |
|
|
d |
d |
dx |
|
l |
|
D |
Uranium sample |
|
|
|
in container |
Fig. 5.5. Schematic geometry of uranium enrichment for the sample gamma radiation
Following the calculation of the integral and transformations (and a more detailed representation of the sample composition), the formula (5.7) is reduced to the following form:
|
E ×ТC ×Тff ×(1-Тsam ) |
|
n |
р = K × [1+ (μM / μU ) ×(ρM / ρU )], |
(5.8) |
where K = [(Wd / 4π ) ×ε ×λ235 × I × AU ×Tf ]; Тsam is the transmission factor for the examined sample; Тc is the transmission factor for the container wall; Тf is the filter transmission factor; μU, ρU is the uranium mass attenuation factor and density; and μM, ρM is the matrix mass attenuation factor and density.
The term [1+ (μM M / μU ) ×(ρM / ρU )] accounts for the uranium dilution
in the sample by other materials (oxygen, fluorine, plutonium, etc.). It depends on the composition of the measured material.
K is found with the aid a physical standard and the value thereof turns into the calibration factor. Therefore, the enrichment value sought for is obtained from the formula:
238
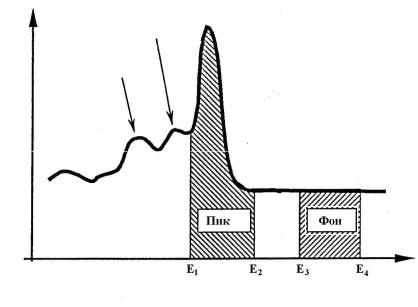
E = |
nр ×[1+ (μM / μU ) ×(ρM / ρU )] |
. |
(5.9) |
|
|||
|
K ×ТC ×Тf ×(1-Тsam ) |
|
Now we shall compare the measurements with NaIand Ge-
spectrometers. The portion of the spectrum obtained on a NaI-spectrometer in the region of the 235U peak of 185.7 keV is shown in Fig. 5.6.
The count of pulses in the photopeak Sph = p – f ×b where p is the total count of pulses in the preset range of energies Е1– Е2 that includes the photopeak of 185.7 keV; b is the total count of background pulses in the range above the peak (see Fig. 5.6); and f is the factor of scaling between the measured background in the peak region. The background is estimated via extrapolation by the numbers of counts in the channels above the peak.
In analyses of infinitely thick samples Е=nр/K=А×p+В×b, where А and В (В = –f ×А) are calibration factors found by measurements with a standard.
There is no problem with the background subtraction during measurements on semiconductor Ge-detectors. The peaks are approximately 20 times as narrow as during measurements based on NaIdetectors, so the peak/background relation is accordingly higher.
Number of counts
185.7 keV
143-164 keV
X-ray
|
|
Back- |
|
Peak |
|||
|
ground |
||
|
Energy, keV
Fig. 5.6. Gamma radiation spectrum measured by NaI-detector
239

Measurement of the 235U and 238U gamma radiation relative intensity
The major drawback of the uranium enrichment measurement method based on recording of 185.7keV radiation is the requirement to have the measuring system calibrated for each new container with the uranium sample. No such drawback is present in measurements of enrichment from the relative intensity of 235U and 238U gamma radiations.
The gamma radiation spectrum has three energy ranges that can be used for such measurements: 53–68 keV, 84–130 keV and 18 5–1001 keV.
The region of 84–130 keV includes a series of γ- and ХK-lines of uranium isotopes. Radiations of 235U and 238U in this region are very close by energy and so are detected with nearly the same efficiency.
The relation between the concentrations of isotopes in the sample is obtained from the formula:
Ni Nk = nip nkp ×Т1i / 2 Т1k/ 2 × Iγk Iγi × e kγ eiγ , |
(5.10) |
where Ni, Nk are the numbers of the atoms of respectively the i-th and k-th isotopes in the sample; Т1i/ 2 , Т1k/ 2 are the half-lives of respectively the i-th and k-th isotopes; εγi , εγk are the efficiencies of radiation detection in the
analyzed peaks of respectively the i-th and k-th isotopes, which include, in the given case, the detector efficiency, the geometry of the measurements, self-absorption of radiations in the sample and attenuation of same in the
material between the sample and the detector; and Iγi , Iγk are the quantum
yields of the radiations as detected in the analyzed peaks of respectively the i-th and k-th isotopes.
The count rate nip and the concentration of the i-th isotope relate as follows:
nip |
|
N i × ln 2 |
|
i |
|
|
|
|
|
×εγ . |
(5.11) |
i |
= |
i |
|
||
Iγ |
|
T 1/ 2 |
|
|
|
The term in brackets has the same value for all gamma radiations emitted by one isotope. So the relation ( nip / Iγi ) is proportional to the efficiency
εγi . The relative efficiency measurement procedure includes:
240