
- •6.1 Resistive Displacement Sensors
- •Types of Precision Potentiometers
- •Resistive Element
- •Electrical Characteristics
- •Mechanical Characteristics
- •Mechanical Mounting Methods
- •Implementation
- •6.2 Inductive Displacement Sensors
- •The Single-Coil Linear Variable-Reluctance Sensor
- •The Variable-Differential Reluctance Sensor
- •Variable-Reluctance Tachogenerators
- •Microsyn
- •Synchros
- •Variable-Coupling Transducers
- •Induction Potentiometer
- •Appendix to Section 6.2
- •Variable Distance Displacement Sensors
- •Variable Area Displacement Sensors
- •Variable Dielectric Displacement Sensors
- •Aluminum Type Capacitive Humidity Sensors
- •Tantalum Type Capacitive Humidity Sensors
- •Silicon Type Capacitive Humidity Sensors
- •Polymer Type Capacitive Humidity Sensors
- •Capacitive Moisture Sensors
- •Pulse Width Modulation
- •Square Wave Linearization
- •Feedback Linearization
- •Oscillator Circuits
- •Appendix to Section 6.3
- •6.4 Piezoelectric Transducers and Sensors
- •Single Crystals
- •Piezoelectric Ceramics
- •Perovskites
- •Processing of Piezoelectric Ceramics
- •Piezoelectric Polymers
- •Piezoelectric Ceramic/Polymer Composites
- •Suppliers of Piezoelectric Materials
- •6.5 Laser Interferometer Displacement Sensors
- •Longitudinal Zeeman Effect
- •Two-Frequency Heterodyne Interferometer
- •Single-Mode Homodyne Interferometer
- •6.6 Bore Gaging Displacement Sensors
- •Gages That Control Dimensions
- •Gages That Control Geometry
- •6.7 Time-of-Flight Ultrasonic Displacement Sensors
- •Ultrasound Transducers
- •6.8 Optical Encoder Displacement Sensors
- •Absolute Encoders
- •Incremental Encoders Quadrature Signals
- •Geometric Masking
- •Diffraction-Based Encoders
- •6.9 Magnetic Displacement Sensors
- •6.10 Synchro/Resolver Displacement Sensors
- •Equipment Needed for Testing Resolvers
- •Multispeed Units
- •Applications
- •Resolver-to-Digital Conversion
- •Bandwidth Optimization
- •Encoder Emulation
- •Determining Position Lag Error Due to Acceleration
- •Large Step Settling Time
- •Time Constants
- •6.11 Optical Fiber Displacement Sensors
- •Principle of Operation
- •Fabrication Techniques
- •Bragg Grating Sensors
- •Limitations of Bragg Grating Strain Sensors
- •Principle of Operation
- •Fabrication Procedure
- •Temperature Sensitivity of Long-Period Gratings
- •Knife-Edge Photodetector
- •Bicell Detector
- •Continuous Position Sensor
- •References

The right-hand side of this trigonometric identity indicates that the differencing-junction output represents a carrier-frequency sine wave with an amplitude proportional to the sine of the difference between θ (the angle to be digitized) and φ (the angle stored in digital form in the up/down counter). This point is the ac error signal brought out as (e). The demodulator is also presented with the reference voltage, which has been isolated from the reference source, and appropriately scaled, by the reference conditioner. The output of the demodulator is then, an analog dc level, proportional to sin (θ – φ), in other words, to the sine of the “error” between the actual angular position of the resolver and the digitally encoded angle, φ, which is the output of the counter. This point dc error is sometimes brought out as (D) while an addition of a threshold detector will give a built-in-test (BIT) flag. When the ac error signal exceeds 100 LSBs, the BIT flag will indicate a tracking error. This angular error signal is then fed into the error processor and VCO. This circuit consists essentially of an analog integrator whose output (the time integral to the error) controls the frequency of a voltage-controlled oscillator (VCO). The VCO produces clock pulses that are counted by the up/down counter. The “sense” of the error (φ too high or φ too low) is determined by the polarity of (φ), and is used to generate a control counter signal (U), which determines whether the counter increments upward or downward. Finally, note that the up/down counter, like any counter, is functionally an incremental integrator; therefore, the tracking converter constitutes in itself a closed-loop servomechanism (continuously attempting to null the error to zero) with two integrators in series. This called a “Type II” servo loop, which has decided advantages over Type 1 or Type 0 loops. In order to appreciate the value of a Type II servo behavior of this tracking converter, consider first the shaft is not moving. Ignoring inaccuracies, drifts, and the inevitable quantizing error, the error should be zero (θ = φ), and the digital output represents the true shaft angle of the resolver. Now, start the resolver shaft moving, and allow it to accelerate uniformly, from dθ/dt = 0 to dθ/dt = V. During the acceleration, an error will develop because the converter cannot instantaneously respond to the change of angular velocity. However, since the VCO is controlled by an integrator, the output of which is the integral of the error, the greater the lag (between θ and φ), the faster the counter will be called on to catch up. When the velocity becomes constant at V, the VCO will have settled to a rate of counting that exactly corresponds to the rate of change in θ per unit time and instantaneously θ = φ. Therefore, dφ/dt will always track dθ/dt without a velocity or position error. the only error will be momentary (transient) error, during acceleration or deceleration. Furthermore, the information produced by the tracking converter is always “fresh,” being continually updated, and always available at the output of the counter. Since dθ/dt tracks the input velocity it can be brought out as velocity, a dc voltage proportional to the rate of rotation, which is of sufficient linearity in modern converters to eliminate the need for a tachometer in many systems.
Bandwidth Optimization
When using a low-cost monolithic converter for position and velocity feedback, it is important to understand the dynamic response for a changing input. When considering what bandwidth to set the converter, several parameters must be taken into consideration. The ability to track step responses and accelerations will determine what bandwidth to select. The lower the bandwidth of the resolver-to-digital converter, the greater the noise immunity; high frequency noise will be rejected. The relationship between the maximum tracking rate and bandwidth determines the settling time for small and large steps. For a small step input, the bandwidth determines the converter settling time. When one has a large step, the maximum velocity slew rate and bandwidth together, determine the settling time.
Encoder Emulation
Today’s resolver-to-digital converters also have the ability to emulate the output of an optical incremental encoder. By providing the outputs A, B, and Zero Index, the encoder can be replaced with a resolver and resolver-to-digital converter without changing the existing interface hardware.
© 1999 by CRC Press LLC
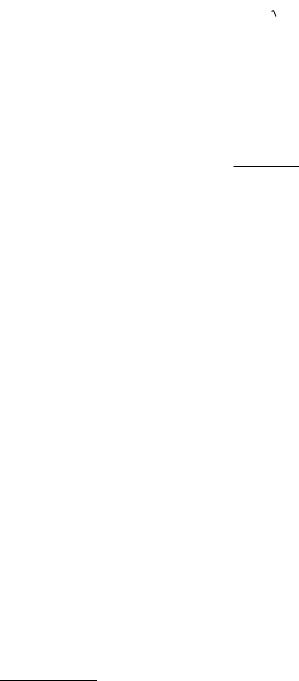
Determining Position Lag Error Due to Acceleration
As the bandwidth and the maximum tracking rate are varied, one can determine the acceleration constant (Ka) and large step settling time.
EXAMPLE: |
|
Resolution: |
16 bit |
Bandwidth: |
100 Hz |
Reference: |
1000 Hz |
Max tracking: |
10 rps |
BW = |
2A |
|
π |
||
|
If the bandwidth = 100 Hz
A = 222
Ka = A2
Ka = 49,284° s–2
The lag in degrees during an acceleration is:
Acceleration
Ka
EXAMPLE:
Acceleration = 19,000° s–2 Ka = 49,284° s–2
LAG = 19,000/49,284 = 0.38° In 16 bit 1 LSB = 0.0055°
Acceleration (1 LSB lag) = Ka × 0.0055 (16 bit) 49,284 × 0.0055 = 270° s–2
Large Step Settling Time
(6.115)
(6.116)
To determine the settling time for a large step response (179°), one must take into account the maximum tracking rate and bandwidth.
EXAMPLE:
A 179° step with 100 Hz BW and 10 rps max tracking Max tracking at 10 rps = 3600° s–1
179/3600 = 49 ms
Then one must add the settling time due to bandwidth limitations; this is approximately 11 time constants (16-bit mode).
Time Constants
|
|
Resolution |
# of counts/rotation |
# of time constants |
|
|
|
|
|
|
10 |
1024 |
7 |
|
|
12 |
4096 |
8 |
|
|
14 |
16384 |
10 |
|
|
16 |
65536 |
11 |
|
|
|
|
|
|
Time constant |
= 1/A |
|
|
|
A |
= 222 |
|
|
|
1/A |
= 4.5 ms |
|
|
|
11 time constants |
= 45 ms |
|
|
© 1999 by CRC Press LLC