
Bioregenerative Engineering Principles and Applications - Shu Q. Liu
..pdf706 VASCULAR REGENERATIVE ENGINEERING
method is superior to that with a metal band described above in terms of controlling the accuracy of arterial constriction.
Conventional Treatment of Hypertension [15.23]. The principle of hypertension treatment is to remove factors that cause hypertension and to reduce arterial blood pressure. As discussed on page 700 of this chapter, renovascular hypertension is caused by renal arterial stenosis. Surgical correction of stenosed renal arteries is usually an effective treatment. Endocrine hypertension may be caused by adrenal gland tumors, which induce excessive secretion of aldosterone (in the case of a cortical tumor) or epinephrine and norepinephrine (in the case of a medulla tumor). Surgical removal of the tumor usually results in a decrease in arterial blood pressure. For essential hypertension, since the causes are poorly understood, there are no effective approaches for the removal of the pathogenic factors.
An increase in arterial blood pressure usually stimulates mitogenic responses of vascular endothelial and smooth muscle cells and enhances atherogenesis. Thus, excessive hypertension should be controlled appropriately. There are two approaches that can be used to reduce arterial blood pressure: diuresis and vasodilation. Diuresis can be induced by agents known as diuretics, which enhance the excretion of water in the kidney. Typical diuretic agents are thiazide derivatives. These agents inhibit the reabsorption of sodium and potassium in the proximal renal tubules and stimulate the secretion of chloride, enhancing water excretion and reducing arterial blood pressure.
Vasodilators are agents that induce the relaxation of vascular smooth muscle cells and thus the reduction of blood pressure. There are several types of vasodilators: blockers of the α adrenergic receptor, suppressors of sympathetic vasomotor centers, blockers of the angiotensin II type 1 receptor, and blockers of calcium channels. The α-adrenergic receptor mediates norepinephrine-induced smooth muscle cell contraction and its activation induces an elevation in arterial blood pressure. The inhibition of the α adrenergic receptor can effectively reduce arterial blood pressure. Typical agents of α adrenergic receptor blockers include phentolamine and phenoxybenzamine. The sympathetic vasomotor centers control the basal tone of small arteries. An increase in the basal tone is a critical factor that contributes to hypertension. Several agents, such as clonidine and methyldopa, can be used to suppress the activity of the sympathetic vasomotor centers and reduce arterial blood pressure. The angiotensin II type 1 receptor mediates angiotensin II-induced contraction of smooth muscle cells. The suppression of the activity of this receptor reduces arterial blood pressure. A typical agent for such a purpose is losartan. Calcium is necessary for vascular smooth muscle contraction. The blockade of calcium channels leads to a reduction in the contractility of smooth muscle cells. Commonly used calcium blockers include nicardipine, nifedipine, nimodipine, and verapamil.
Molecular Engineering [15.24]. While hypertension is commonly treated with agents as described above, these agents are short-lived and often elicit side effects. Since the pathogenesis of hypertension, especially, essential hypertension, is related to the disorder of vascular control genes, the modulation of these genes provides a means for the treatment of hypertension. Strategies for molecular manipulation of hypertension are to enhance the expression of vasodilator genes and suppress the expression of vasoconstrictor genes. There are several basic approaches for achieving the therapeutic goals, including application of full-length DNA and antisense oligonucleotides. These genetic materials can be directly delivered into the blood. Potential genes and oligodeoxynucleotides for these purposes are described as follows.
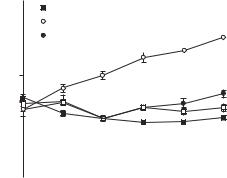

708
TABLE 15.6. Characteristics of Selected Vasodilating Molecules*
|
|
Amino |
Molecular |
|
|
Proteins |
Alternative Names |
Acids |
Weight (kDa) |
Expression |
Functions |
|
|
|
|
|
|
Atrial natriuretic |
Natriuretic peptide precursor A |
151 |
16 |
Atrium, prostate gland |
Inducing smooth muscle relaxation, |
peptide |
(NPPA), atrial natriuretic |
|
|
|
stimulating water and sodium |
|
polypeptide (ANP), |
|
|
|
excretion, enhancing endothelial |
|
prepronatriodilatin, |
|
|
|
regeneration, and regulating the |
|
cardionatrin, atrionatriuretic |
|
|
|
activity of NFκB |
|
factor (ANF), pronatriodilatin |
|
|
|
|
|
(PND), atriopeptin |
|
|
|
|
Kallikrein |
KLK1, renaL/pancreatic/salivary |
262 |
29 |
Prostate gland, uterus |
One of the 15 kallikrein subfamily |
|
kallikrein, KLKR, kallikrein |
|
|
|
members that cleaves kininogens to |
|
serine protease 1 |
|
|
|
produce bradykinin and |
|
KNG, α2-thiol proteinase |
|
|
|
lysylbradykinin |
Kininogen |
427 |
48 |
Liver, blood vessels, platelets, |
Precursor of kinins (bradykinin and |
|
|
inhibitor |
|
|
kidney, placenta |
lysylbradykinin) |
|
|
|
|
|
|
*Based on bibliography 15.26.
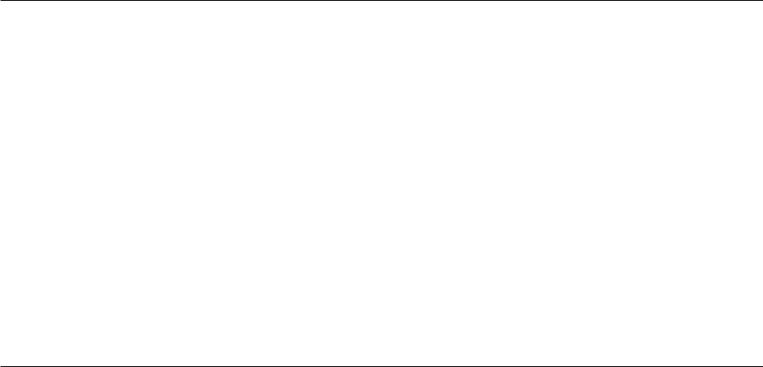
BIBLIOGRAPHY 711
Ladenvall P, Nilsson S, Jood K, Rosengren A, Blomstrand C et al: Genetic variation at the human tissue-type plasminogen activator (tPA) locus: haplotypes and analysis of association to plasma levels of tPA, Eur J Hum Genet 11:603–10, 2003.
Loscalzo J, Braunwald E: Tissue plasminogen activator, New Engl J Med 319:925–31, 1988.
Ludwig M, Wohn KD, Schleuning WD, Olek K: Allelic dimorphism in the human tissue-type plasminogen activator (TPA) gene as a result of an Alu insertion/deletion event, Hum Genet 88:388–92, 1992.
Pang PT, Teng HK, Zaitsev E, Woo NT, Sakata K et al: Cleavage of proBDNF by tPA/plasmin is essential for long-term hippocampal plasticity, Science 306:487–91, 2004.
Pennica D, Holmes WE, Kohr WJ, Harkins RN, Vehar GA et al: Cloning and expression of human tissue-type plasminogen activator cDNA in E. coli, Nature 301:214–21, 1983.
Seeds NW, Basham ME, Haffke SP: Neuronal migration is retarded in mice lacking the tissue plasminogen activator gene, Proc Natl Acad Sci USA 96:14118–23, 1999.
Seeds NW, Williams BL, Bickford PC: Tissue plasminogen activator induction in Purkinje neurons after cerebellar motor learning, Science 270:1992–4, 1996.
Tishkoff SA, Pakstis AJ, Stoneking M, Kidd JR, Destro-Bisol G et al: Short tandem-repeat polymorphism/Alu haplotype variation at the PLAT locus: Implications for modern human origins, Am J Hum Genet 67:901–25, 2000.
Wang X, Lee SR, Arai K, Lee SR, Tsuji K et al: Lipoprotein receptor-mediated induction of matrix metalloproteinase by tissue plasminogen activator, Nature Med 9:1313–7, 2003.
Plasminogen
Azuma H, Uno Y, Shigekiyo T, Saito S: Congenital plasminogen deficiency caused by a ser572-to- pro mutation, Blood 82:475–80, 1993.
Bugge TH, Flick MJ, Daugherty CC, Degen JL: Plasminogen deficiency causes severe thrombosis but is compatible with development and reproduction, Genes Dev 9:794–807, 1995.
Cao Y, Ji RW, Davidson D, Schaller J, Marti D et al: Kringle domains of human angiostatin: Characterization of the anti-proliferative activity on endothelial cells J Biol Chem 271:29461–7, 1996.
Cao Y, O’Reilly MS, Marshall B, Flynn E, Ji RW et al: Expression of angiostatin cDNA in a murine fibrosarcoma suppresses primary tumor growth and produces long-term dormancy of metastases, J Clin Invest 101:1055–63, 1998.
Drew AF, Kaufman AH, Kombrinck KW, Danton MJ, Daugherty CC et al: Ligneous conjunctivitis in plasminogen-deficient mice, Blood 91:1616–24, 1998.
Fischer MB, Roeckl C, Parizek P, Schwarz HP, Aguzzi A: Binding of disease-associated prion protein to plasminogen, Nature 408:479–83, 2000.
Ichinose A, Espling ES, Takamatsu J, Saito H, Shinmyozu K et al: Two types of abnormal genes for plasminogen in families with a predisposition for thrombosis, Proc Natl Acad Sci USA 88:115–9, 1991.
O’Reilly MS, Holmgren L, Shing Y, Chen C, Rosenthal RA et al: Angiostatin: A novel angiogenesis inhibitor that mediates the suppression of metastases by a Lewis lung carcinoma, Cell 79:315– 28, 1994.
Petersen TE, Martzen MR, Ichinose A, Davie EW: Characterization of the gene for human plasminogen, a key proenzyme in the fibrinolytic system, J Biol Chem 265:6104–11, 1990.
Romer J, Bugge TH, Pyke C, Lund LR, Flick MJ et al: Impaired wound healing in mice with a disrupted plasminogen gene, Nature Med 2:287–92, 1995.
Schott D, Dempfle CE, Beck P, Liermann A, Mohr-Pennert A et al: Therapy with a purified plasminogen concentrate in an infant with ligneous conjunctivitis and homozygous plasminogen deficiency, New Engl J Med 339:1679–86, 1998.
712 VASCULAR REGENERATIVE ENGINEERING
Swaisgood CM, Schmitt D, Eaton D, Plow EF: In vivo regulation of plasminogen function by plasma carboxypeptidase, Br J Clin Invest 110:1275–82, 2002.
Protein C
Allaart CF, Poort SR, Rosendaal FR, Reitsma PH, Bertina RM et al: Increased risk of venous thrombosis in carriers of hereditary protein C deficiency defect, Lancet 341:134–8, 1993.
Beckmann RJ, Schmidt RJ, Santerre RF, Plutzky J, Crabtree GR et al: The structure and evolution of a 461 amino acid human protein C precursor and its messenger RNA, based upon the DNA sequence of cloned human liver cDNAs, Nucleic Acids Res 13:5233–47, 1985.
Bernard GR, Vincent JL, Laterre PF, LaRosa SP, Dhainaut JF et al: Recombinant Human Activated Protein C Worldwide Evaluation in Severe Sepsis (PROWESS) Study Group Efficacy and safety of recombinant human activated protein C for severe sepsis, New Engl J Med 344:699–709, 2001.
Conard J, Horellou MH, van Dreden P, Samama M, Reitsma PH et al: Homozygous protein C deficiency with late onset and recurrent coumarin-induced skin necrosis, Lancet 339:743–4, 1992.
Drews R, Paleyanda RK, Lee TK, Chang RR, Rehemtulla A et al: Proteolytic maturation of protein C upon engineering the mouse mammary gland to express furin, Proc Natl Acad Sci USA 92:10462–6, 1995.
Dreyfus M, Magny JF, Bridey F, Schwarz HP, Planche C et al: Treatment of homozygous protein C deficiency and neonatal purpura fulminans with a purified protein C concentrate, New Engl J Med 325:1565–8, 1991.
Faust SN, Levin M, Harrison OB, Goldin RD, Lockhart MS et al: Dysfunction of endothelial protein C activation in severe meningococcal sepsis, New Engl J Med 345:408–16, 2001.
Hasstedt SJ, Bovill EG, Callas PW, Long GL: An unknown genetic defect increases venous thrombosis risk, through interaction with protein C deficiency, Am J Hum Genet 63:569–76, 1998.
Lay AJ, Liang Z, Rosen ED, Castellino FJ: Mice with a severe deficiency in protein C display prothrombotic and proinflammatory phenotypes and compromised maternal reproductive capabilities, J Clin Invest 115:1552–61, 2005.
Manns BJ, Lee H, Doig CJ, Johnson D, Donaldson C: An economic evaluation of activated protein C treatment for severe sepsis, New Engl J Med 347:993–1000, 2002.
Riewald M, Petrovan RJ, Donner A, Mueller BM, Ruf W: Activation of endothelial cell protease activated receptor 1 by the protein C pathway, Science 296:1880–2, 2002.
Siegel JP: Assessing the use of activated protein C in the treatment of severe sepsis, New Engl J Med 347:1030–4, 2002.
Warren HS, Suffredini AF, Eichacker PQ, Munford RS: Risks and benefits of activated protein C treatment for severe sepsis, New Engl J Med 347:1027–30, 2002.
Von Willebrand Factor
Mancuso DJ, Tuley EA, Westfield LA, Worrall NK, Shelton-Inloes BB et al: Structure of the gene for human von Willebrand factor, J Biol Chem 264:19514–27, 1989.
Sadler JE, Shelton-Inloes BB, Sorace JM, Harlan JM, Titani K et al: Cloning and characterization of two cDNAs coding for human von Willebrand factor, Proc Natl Acad Sci USA 82:6394–8, 1985.
Mancuso DJ, Tuley EA, Westfield LA, Lester-Mancuso TL, Le Beau MM et al: Human von Willebrand factor gene and pseudogene: structural analysis and differentiation by polymerase chain reaction, Biochemistry 30:253–69, 1991.
Ginsburg D, Handin RI, Bonthron DT, Donlon TA, Bruns GAP et al: Human von Willebrand factor (vWF): Isolation of complementary DNA (cDNA) clones and chromosomal localization, Science 228:1401–6, 1985.
BIBLIOGRAPHY 713
Lynch DC, Zimmerman TS, Collins CJ, Brown M, Morin MJ et al: Molecular cloning of cDNA for human von Willebrand factor: Authentication by a new method, Cell 41:49–56, 1985.
Emsley J, Cruz M, Handin R, Liddington R: Crystal structure of the von Willebrand factor A1 domain and implications for the binding of platelet glycoprotein Ib, J Biol Chem 273:10396–401, 1998.
Tuley EA, Gaucher C, Jorieux S, Worrall NK, Sadler JE et al: Expression of von Willebrand factor “Normandy”: an autosomal mutation that mimics hemophilia A, Proc Natl Acad Sci USA 88:6377–81, 1991.
Ribba AS, Voorberg J, Meyer D, Pannekoek H, Pietu G: Characterization of recombinant von Willebrand factor corresponding to mutations in type IIA and type IIB von Willebrand disease, J Biol Chem 267:23209–15, 1992.
Tissue Thromboplastin
Bogdanov VY, Balasubramanian V, Hathcock J, Vele O, Lieb M et al: Alternatively spliced human tissue factor: a circulating, soluble, thrombogenic protein, Nature Med 9:458–62, 2003.
Carson SD, Henry WM, Shows TB: Tissue factor gene localized to human chromosome 1 (1pter1p21), Science 229:991–3, 1985.
Davie EW, Fujikawa K, Kisiel W: The coagulation cascade: Initiation, maintenance, and regulation, Biochemistry 30:10363–70, 1991.
Erlich J, Parry GCN, Fearns C, Muller M, Carmeliet P et al: Tissue factor is required for uterine hemostasis and maintenance of the placental labyrinth during gestation, Proc Natl Acad Sci USA 96:8138–43, 1999.
Isermann B, Sood R, Pawlinski R, Zogg M, Kalloway S et al: The thrombomodulin-protein C system is essential for the maintenance of pregnancy, Nature Med 9:331–7, 2003.
Pawlinski R, Fernandes A, Kehrle B, Pedersen B, Parry G et al: Tissue factor deficiency causes cardiac fibrosis and left ventricular dysfunction, Proc Natl Acad Sci USA 99:15333–8, 2002.
Toomey JR, Kratzer KE, Lasky NM, Broze GJ, Jr: Effect of tissue factor deficiency on mouse and tumor development, Proc Natl Acad Sci USA 94:6922–6, 1997.
Prothrombin
Banfield DK, MacGillivray RTA: Partial characterization of vertebrate prothrombin cDNAs: Amplification and sequence analysis of the B chain of thrombin from nine different species,
Proc Natl Acad Sci USA 89:2779–83, 1992.
Celikel R, McClintock RA, Roberts JR, Mendolicchio GL, Ware J et al: Modulation of alphathrombin function by distinct interactions with platelet glycoprotein Ib-alpha, Science 301:218– 21, 2003.
Degen SJF, Davie EW: Nucleotide sequence of the gene for human prothrombin, Biochemistry 26:6165–77, 1987.
De Stefano V, Martinelli I, Mannucci PM, Paciaroni K, Chiusolo P et al: The risk of recurrent deep venous thrombosis among heterozygous carriers of both factor V Leiden and the G20210A prothrombin mutation, New Engl J Med 341:801–6, 1999.
Dumas JJ, Kumar R, Seehra J, Somers WS, Mosyak L: Crystal structure of the Gp1b-alpha- thrombin complex essential for platelet aggregation, Science 301:222–6, 2003.
Gehring NH, Frede U, Neu-Yilik G, Hundsdoerfer P, Vetter B et al: Increased efficiency of mRNA 3-prime end formation: a new genetic mechanism contributing to hereditary thrombophilia, Nature Genet 28:389–92, 2001.
Martinelli I, Sacchi E, Landi G, Taioli E, Duca F et al: High risk of cerebral-vein thrombosis in carriers of a prothrombin-gene mutation and in users of oral contraceptives, New Engl J Med 338:1793–7, 1998.
714 VASCULAR REGENERATIVE ENGINEERING
Sun WY, Witte DP, Degen JL, Colbert MC, Burkart MC et al: Prothrombin deficiency results in embryonic and neonatal lethality in mice, Proc Natl Acad Sci USA 95:7597–602, 1998.
Fibrinogen
Collen A, Maas A, Kooistra T, Lupu F, Grimbergen J et al: Aberrant fibrin formation and crosslinking of fibrinogen Nieuwegein, a variant with a shortened A-alpha-chain, alters endothelial capillary tube formation, Blood 97:973–80, 2001.
Crabtree GR, Kant JA: Molecular cloning of cDNA for the alpha, beta, and gamma chains of rat fibrinogen: A family of coordinately regulated genes, J Biol Chem 256:9718–23, 1981.
Crabtree GR, Kant JA: Coordinate accumulation of the mRNAs for the alpha, beta, and gamma chains of rat fibrinogen following defibrination, J Biol Chem 257:7277–9, 1982.
Drew AF, Liu H, Davidson JM, Daugherty CC, Degen JL: Wound-healing defects in mice lacking fibrinogen, Blood 97:3691–8, 2001.
Fu Y, Cao Y, Hertzberg KM, Grieninger G: Fibrinogen alpha genes: Conservation of bipartite transcripts and carboxy-terminal-extended alpha subunits in vertebrates, Genomics 30:71–6, 1995.
Kant JA, Fornace AJ Jr, Saxe D, Simon MI, McBride OW et al: Evolution and organization of the fibrinogen locus on chromosome 4: gene duplication accompanied by transposition and inversion, Proc Natl Acad Sci USA 82:2344–8, 1985.
Neerman-Arbez M, de Moerloose P, Bridel C, Honsberger A, Schonborner A et al: Mutations in the fibrinogen A-alpha gene account for the majority of cases of congenital afibrinogenemia, Blood 96:149–52, 2000.
Neerman-Arbez M, Honsberger A, Antonarakis SE, Morris MA: Deletion of the fibrogen (sic) alpha-chain gene (FGA) causes congenital afibrogenemia [sic], J Clin Invest 103:215–8, 1999.
15.3. Regulation of Vascular Contractility
Endothelin 1
Kim TH, Xiong H, Zhang Z, Ren B: beta-Catenin activates the growth factor endothelin-1 in colon cancer cells, Oncogene 24(4):597–604, 2005.
Jin JJ, Nakura J, Wu Z, Yamamoto M, Abe M et al: Association of endothelin-1 gene variant with hypertension, Hypertension 41(1):163–7, 2003.
Arinami T, Ishikawa M, Inoue A, Yanagisawa M, Masaki T et al: Chromosomal assignments of the human endothelin family genes: the endothelin-1 gene (EDN1) to 6p23–p24, the endothelin- 2 gene (EDN2) to 1p34, and the endothelin-3 gene (EDN3) to 20q13.2–q13.3, Am J Hum Genet 48:990–6, 1991.
Bloch KD, Friedrich SP, Lee ME, Eddy RL, Shows TB et al: Structural organization and chromosomal assignment of the gene encoding endothelin, J Biol Chem 264:10851–7, 1989.
Bourgeois C, Robert B, Rebourcet R, Mondon F, Mignot TM et al: Endothelin-1 and ET(A) receptor expression in vascular smooth muscle cells from human placenta: A new ET(A) receptor messenger ribonucleic acid is generated by alternative splicing of exon 3, J Clin Endocr Metab 82:3116–23, 1997.
Campia U, Cardillo C, Panza JA: Ethnic differences in the vasoconstrictor activity of endogenous endothelin-1 in hypertensive patients, Circulation 109:3191–5, 2004.
Ieda M, Fukuda K, Hisaka Y, Kimura K, Kawaguchi H et al: Endothelin-1 regulates cardiac sympathetic innervation in the rodent heart by controlling nerve growth factor expression, J Clin Invest 113:876–84, 2004.
Inoue A, Yanagisawa M, Takuwa Y, Mitsui Y, Kobayashi M et al: The human preproendothelin-1 gene: Complete nucleotide sequence and regulation of expression, J Biol Chem 264:14954–9, 1989.
BIBLIOGRAPHY 715
Khodorova A, Navarro B, Jouaville LS, Murphy JE, Rice FI et al: Endothelin-B receptor activation triggers an endogenous analgesic cascade at sites of peripheral injury, Nature Med 9:1055–61, 2003.
Kurihara Y, Kurihara H, Oda H, Maemura K, Nagai R et al: Aortic arch malformations and ventricular septal defect in mice deficient in endothelin-1, J Clin Invest 96:293–300, 1995.
Kurihara Y, Kurihara H, Suzuki H, Kodama T, Maemura K et al: Elevated blood pressure and craniofacial abnormalities in mice deficient in endothelin-1, Nature 368:703–10, 1994.
Remuzzi G, Perico N, Benigni A: New therapeutics that antagonize endothelin: Promises and frustrations, Nature Rev 1:986–1001, 2002.
Shohet RV, Kisanuki YY, Zhao XS, Siddiquee Z, Franco F et al: Mice with cardiomyocyte-specific disruption of the endothelin-1 gene are resistant to hyperthyroid cardiac hypertrophy, Proc Natl Acad Sci USA 101:2088–93, 2004.
Yanagisawa H, Hammer RE, Richardson JA, Williams SC, Clouthier DE et al: Role of endothelin- 1/endothelin-A receptor-mediated signaling pathway in the aortic arch patterning in mice, J Clin Invest 102:22–33, 1998.
Yanagisawa H, Yanagisawa M, Kapur RP, Richardson JA, Williams SC et al: Dual genetic pathways of endothelin-mediated intercellular signaling revealed by targeted disruption of endothelin converting enzyme-1 gene, Development 125:825–36, 1998.
Yang LL, Gros R, Kabir MG, Sadi A, Gotlieb AI et al: Conditional cardiac overexpression of endothelin-1 induces inflammation and dilated cardiomyopathy in mice, Circulation 109:255– 61, 2004.
Yin JJ, Mohammad KS, Kakonen SM, Harris S, Wu-Wong JR et al: A causal role for endothelin-1 in the pathogenesis of osteoblastic bone metastases, Proc Natl Acad Sci USA 100:10954–9, 2003.
Xu SW, Howat SL, Renzoni EA, Holmes A, Pearson JD et al: Endothelin-1 induces expression of matrix-associated genes in lung fibroblasts through MEK/ERK, J Biol Chem 279(22):23098– 103, 2004.
Endothelin 2
Arinami T, Ishikawa M, Inoue A, Yanagisawa M, Masaki T et al: Chromosomal assignments of the human endothelin family genes: The endothelin-1 gene (EDN1) to 6p23-p24, the endothelin- 2 gene (EDN2) to 1p34, and the endothelin-3 gene (EDN3) to 20q13.2-q13.3, Am J Hum Genet 48:990–6, 1991.
Bloch KD, Hong CC, Eddy RL, Shows TB, Quertermous T: cDNA cloning and chromosomal assignment of the endothelin 2 gene: Vasoactive intestinal contractor peptide is rat endothelin 2, Genomics 10:236–42, 1991.
Deng AY, Dene H, Pravenec M, Rapp JP: Genetic mapping of two new blood pressure quantitative trait loci in the rat by genotyping endothelin system genes, J Clin Invest 93:2701–9, 1994.
Ohkubo S, Ogi K, Hosoya M, Matsumoto H, Suzuki N et al: Specific expression of human endo- thelin-2 (ET-2) gene in a renal adenocarcinoma cell line: Molecular cloning of cDNA encoding the precursor of ET-2 and its characterization, FEBS Lett 274:136–40, 1990.
Endothelin 3
Arinami T, Ishikawa M, Inoue A, Yanagisawa M, Masaki T et al: Chromosomal assignments of the human endothelin family genes: the endothelin-1 gene (EDN1) to 6p23–p24, the endothelin- 2 gene (EDN2) to 1p34, and the endothelin-3 gene (EDN3) to 20q13.2–q13.3, Am J Hum Genet 48:990–6, 1991.
Baynash AG, Hosoda K, Giaid A, Richardson JA, Emoto N et al: Interaction of endothelin-3 with endothelin-B receptor is essential for development of epidermal melanocytes and enteric neurons, Cell 79:1277–85, 1994.