
Bioregenerative Engineering Principles and Applications - Shu Q. Liu
..pdf
666
TABLE 15.2. Characteristics of Selected Vasoconstrictors*
|
|
Amino |
Molecular |
|
|
Proteins |
Alternative Names |
Acids |
Weight (kDa) |
Expression |
Functions |
|
|
|
|
|
|
Endothelin 1 |
ET1, EDN1 |
212 |
24 |
Ubiquitous |
Stimulating smooth muscle contraction, |
|
|
|
|
|
inducing cell proliferation, causing |
|
|
|
|
|
tumorigenesis, and mediating matrix |
|
|
|
|
|
production |
Endothelin 2 |
ET2, EDN2 |
178 |
20 |
Ubiquitous |
Inducing vasoconstriction (most potent |
|
|
|
|
|
vasoconstrictor among endothelin 1,2,3), |
|
|
|
|
|
promoting tumor cell survival, and serving |
|
|
|
|
|
as a macrophage chemoattractant |
Endothelin 3 |
ET3, EDN3 |
238 |
25 |
Lung, spleen, pancreas |
Inducing smooth muscle contraction, |
|
|
|
|
|
regulating the development of neural crest- |
|
|
|
|
|
derived cells, and stimulating cell division |
Endothelin |
ETA, ETRA |
427 |
49 |
Blood vessels, placenta, |
A G-protein-coupled receptor for |
receptor type A |
|
|
|
skeletal muscle |
endothelin 1,3 |
Endothelin |
ETB, ETRB, endothelin |
442 |
50 |
Ubiquitous |
A G-protein-coupled receptor for endothelin |
receptor type B |
receptor, nonselective type |
|
|
|
1,3, regulating neural development |
|
|
|
|
|
|
*Based on bibliography 15.3.

668 VASCULAR REGENERATIVE ENGINEERING
Endothelial cells are also able to synthesize and release growth inhibitors, including transforming growth factor β (TGFβ), heparan sulfate, and nitric oxide synthase. TGFβ has been shown to inhibit the proliferation of endothelial and smooth muscle cells. Heparan sulfate is a potent inhibitor of smooth muscle proliferation as well as an inhibitor of smooth muscle phenotype modulation. Nitric oxide synthase catalyzes the formation of nitric oxide, which exerts an inhibitory effect on the proliferation of endothelial and smooth muscle cells. In a homeostatic state, a coordinated regulation of the synthesis and secretion of growth promoters and inhibitors is critical to the control of cell proliferation and vascular remodeling.
Production of Extracellular Matrix [15.1]. Endothelial cells can synthesize and release several extracellular components, including collagen type IV, fibronectin, and laminin. These components participate in the construction of the vascular basal lamina. In addition, endothelial cells can produce proteoglycans, which constitute the ground substance compartment of the intima.
Smooth Muscle Cells [15.5]. Smooth muscle cells are fiber-like muscular cells found in the tunica media of arteries and veins. These cells are primarily aligned in the circumferential direction of blood vessels. Vascular smooth muscle cells can be identified by their location; all cells localized to the media of arteries and veins are defined as smooth muscle cells. Smooth muscle cells express several distinct molecular markers, including smooth muscle α actin (see Chapter 15 opening figure), smooth myosin 1, and calponin. These markers are used in biological research to identify vascular smooth muscle cells.
Although smooth muscle cells are present in the wall of arteries and veins, the distribution of smooth muscle cells differs between the two type of blood vessels. Arteries contain multiple layers of smooth muscle cells, which are organized between elastic laminae. In contrast, veins contain a single layer of smooth muscle cells, which are found underneath the intimal layer of the venous wall. For the same generation of blood vessels, the number of smooth muscle cells in the arterial wall is considerably larger than that in the venous wall.
Smooth muscle cells possess a number of functions, including contraction and relaxation, regulation of vascular cell proliferation and migration, and synthesis of extracellular matrix constituents. These functions are discussed as follows.
Contractility [15.5]. Smooth muscle contraction and relaxation are processes induced by interaction between α-actin filaments and myosin molecules. The sliding of myosin molecules along actin filaments drives the shortening of the cells, whereas the dislodgment of the myosin molecules from the actin filaments results in cell relaxation. The interaction of myosin molecules with actin filaments is initiated by an increase in the concentration of cytoplasmic calcium, which is stored in and released from the endoplasmic reticulum. Calcium is an critical ion that participates in regulating the activities of contractility-mediating signaling molecules. While a complete signaling pathway remains to be determined, it has been demonstrated that myosin light chain kinase (MLCK) is a key downstream regulatory factor for the activation of myosin molecules. MLCK can be activated in response to an increase in the cytoplasmic calcium concentration. Activated MLCK induces phosphorylation of the myosin light chain, resulting in the activation of the myosin ATPase and the interaction of myosin molecules with actin filaments. Several signaling molecules, including Rho kinase and mitogen-activated protein kinase, have been shown to directly phosphorylate and activate MLCK.


670VASCULAR REGENERATIVE ENGINEERING
τ= 4μQ πr3
An increase in fluid shear stress, due to elevated bloodflow or reduced vessel diameter, may induce deformation or conformational changes of molecules at the cell surface. Such mechanical stimulation may activate signaling pathways, resulting activation of the vasorelaxant mechanisms, including the activation of nitric oxide synthase and the release of nitric oxide. The consequence is smooth muscle relaxation and vessel dilation. The physiological significance of these activities is that an increase in fluid shear stress, often resulting from elevated demand of regional bloodflow, stimulates the dilation of blood vessels, leading to the enhancement of regional blood supply.
Regulation of Cell Proliferation and Migration [15.5]. Smooth muscle cells play an important role in regulating the proliferation and migration of vascular cells, including themselves and fibroblasts. Smooth muscle cells exert such an effect via synthesizing and releasing growth factors, including platelet-derived growth factor (PDGF), insulin-like growth factor (ILGF), and fibroblast growth factor (FGF). These growth factors can act on vascular cells via interaction with corresponding growth factor receptor protein tyrosine kinases and activate cytoplasmic mitogenic signaling pathways, resulting in cell proliferation and migration. In particular, smooth muscle cell proliferation and migration from the media to the intima contribute to intimal hyperplasia and atherogenesis. The characteristics and activation mechanisms of the growth factors are discussed on page 151, respectively.
Regulation of Extracellular Matrix Production [15.5]. Smooth muscle cells can synthesize and release extracellular matrix constituents, including primarily collagen type III, tropoelastin, and proteoglycans. Collagen is assembled into collagen fibers, tropoelastin is organized into elastic fibers, which constitute elastic laminae in the wall of arteries, and proteoglycans constitute the ground substance compartment of blood vessels.
Fibroblasts [15.1]. Fibroblasts are cells found in the adventitia of arteries and veins. These cells are randomly organized through the adventitia. The density of fibroblasts is considerably lower than that of the medial smooth muscle cells. Fibroblasts possess two major functions: production of extracellular matrix constituents and regulation of vascular cell proliferation. Fibroblasts can synthesize and release collagen type III and proteoglycans, which constitute the extracellular matrix of the adventitia. Fibroblasts regulate the proliferation of vascular cells via production of growth factors, such as fibroblast growth factor (FGF) and epidermal growth factor (EGF). These growth factor can interact with corresponding growth factor receptors and induce proliferative activities.
Extracellular Matrix of Blood Vessels [15.1]
The extracellular matrix of blood vessels is composed of the basal lamina, collagen fibers, elastic fibers and laminae, and proteoglycans. These matrix components provide structural support, mechanical strength, and elasticity to the wall of blood vessels, and play critical roles in regulating the development, morphogenesis, and pathogenic remodeling of blood vessels. The basal lamina is a thin membrane found underneath the endothelium and serves as a substrate for the endothelial cells. This membrane consists of collagen type IV, fibronectin, and laminin. In the case of blood vessel injury and endothelial detachment,
ANATOMY AND PHYSIOLOGY OF THE VASCULAR SYSTEM |
671 |
the basal lamina initiates platelet and leukocyte adhesion and triggers blood coagulation, contributing to the repair of damaged blood vessels.
The vascular collagen matrix is composed of primarily collagen type III and determines the strength of blood vessels, especially at a high blood pressure level. This matrix also serves as a substrate for the attachment of smooth muscle cells and fibroblasts, stimulates the proliferation and migration of vascular cells, and controls the pattern formation of smooth muscle cells. Elastic fibers and laminae are primary matrix components that are responsible for the elasticity of blood vessels. In addition, these components exert an inhibitory effect on cell proliferation and migration via activation of a signaling pathway involving the inhibitory receptor signal-regulatory protein α and SH2 domain-containing protein tyrosine phosphatase (see page 201 for details). Proteoglycans are the components of ground substances, which serve as a structure for the assembly and organization of vascular cells. A detailed discussion of extracellular matrix can be found on page 102.
Regulation of Bloodflow [15.1]
The primary function of the vascular system is to conduct oxygenated bloodflow from the beating heart to peripheral tissues and return deoxygenated blood from the peripheral tissues to the heart, maintaining the circulation of bloodflow. At a given state and a given time, the rate of blood supply differs between different organ systems because of different metabolic activities. For instance, the cardiac and skeletal muscular systems demand a considerable increase in bloodflow during physical exercise, while bloodflow to other less active systems, such as the gastrointestinal system, is accordingly reduced. Such a coordinated control of regional bloodflow is achieved by regulating the contractility of local arterial smooth muscle cells. The arterial smooth muscle cells in the cardiac and skeletal muscle systems undergo relaxation, whereas the intestinal arterial smooth muscle cells undergo enhanced contraction. Thus, bloodflow is redistributed from the intestinal system to the cardiac and skeletal muscle systems during physical exercises.
The redistribution of bloodflow is regulated via several mechanisms, including regulation by local metabolites, the nervous system, and hormones. Local metabolites, including carbon dioxide, H+, lactic acid, adenosine, adenosine monophosphate, and adenosine diphosphate, play a critical role in regulating regional bloodflow. These metabolites are generated during metabolic processes and stimulate the relaxation of vascular smooth muscle cells. An increase in metabolism during physical exercises results in the elevation of these metabolites and induces the dilation of local arteries, leading to an increase in the rate of regional bloodflow. This mechanism contributes to the enhancement of bloodflow to the heart and skeletal muscle system during exercises.
Arteries are innervated with sympathetic nerves, which secret norepinephrine. The function of norepinephrine is dependent on the type of adrenergic receptors in the smooth muscle cells. There are two major types of adrenergic receptors: α- and β-adrenergic receptors. The binding of norepinephrine to the α-adrenergic receptor induces primarily smooth muscle cell contraction, whereas the binding to the β-adrenergic receptor induces smooth muscle cell relaxation. The distribution of the two types of adrenergic receptor in arteries varies between different organs. Arteries in the brain, heart, and skeletal muscle system are dominantly innervated with the β-adrenergic receptor, whereas the gastrointestines, kidney, and skin are primarily innervated with the α-adrenergic receptor. Under conditions of increased physical activities, the activation of the sympathetic nerve system induces arterial dilation in the heart, brain, and skeletal muscular systems, whereas such
672 VASCULAR REGENERATIVE ENGINEERING
a change exerts an opposite effect on the arterial system in the stomach, intestines, kidneys, and skin. This mechanism contributes to blood flow redistribution to the brain, heart, and skeletal muscle system during physical exercises. The effect of bloodborne hormones, such as epinephrine and norepinephrine produced in the adrenal gland, elicit a similar effect on the contractility of arterial smooth muscle cells. These hormones are upregulated during exercises or under stress conditions (e.g., escaping from dangers).
Regulation of Blood Pressure [15.1]
The circulation of blood flow is driven by the arterial blood pressure, which is generated by the cardiac pumping activity. Arterial blood pressure varies periodically during a cardiac cycle. It is about 120 mm Hg during the systole and about 80 mm Hg during the diastole under physiological conditions. An increase or decrease in arterial blood pressure, defined as hypertension or hypotension, respectively, may influence the function of a variety of tissues and organs. For instance, hypertension may cause cardiac hypertrophy and failure, and extreme hypotension may result in deficiency of blood and oxygen supply. Arterial blood pressure is controlled within a narrow range under physiological conditions.
There are a number of mechanisms which control the arterial blood pressure. These mechanisms include regulation by the baroreceptors, chemoreceptors, central nerve control centers, rennin–angiotensin–aldosterone system, and vasopressin system. These mechanisms are briefly discussed as follows.
Regulation by Baroreceptors [15.1]. Baroreceptors are sensory cells localized to the carotid sinus near the carotid bifurcation and to the aortic arch, and are capable of sensing mechanical stretching signals due to arterial blood pressure. An increase in arterial blood pressure stimulates the baroreceptors, enhancing the generation of action potentials. The action potentials are transmitted to the central cardiovascular control centers via the glossopharyngeal and vagus nerves. The central control centers in turn reduce the activity of the sympathetic nerve system, leading to a decrease in arterial blood pressure. A decrease in arterial blood pressure exerts an opposite effect on the central nerve control centers, resulting in an increase in arterial blood pressure. Through such a feedback system, the baroreceptors control the arterial blood pressure within a narrow range under physiological conditions.
Regulation by Chemoreceptors [15.1]. Chemoreceptors are sensory cells found in structures known as carotid and aortic bodies localized to the bifurcation of carotid arteries and aortic arch. These receptors sense chemical signals such as oxygen, carbon dioxide, and hydrogen ion and regulate arterial blood pressure based on the blood concentration of these substances. The carotid and aortic bodies receive rich blood supply. When arterial blood pressure decreases, the concentration of oxygen decreases in the carotid and aortic bodies, whereas the concentration of hydrogen and carbon dioxide increases. These changes activate the chemoreceptors, increasing the frequency of action potentials in the receptor cells. The action potentials are transmitted to the central vasomotor nerve control centers, which in turn activate the sympathetic nervous system. Activated sympathetic nerves enhance cardiac contractility and arterial contraction, resulting in an increase in arterial blood pressure. It should be noted that the chemoreceptors are usually activated in response to a rapid decrease in arterial blood pressure under emergency conditions, such as hemorrhage and shock. They are not activated under physiological conditions.
ANATOMY AND PHYSIOLOGY OF THE VASCULAR SYSTEM |
673 |
Regulation by Central Nerve Control Centers [15.1]. There are neurons which sense changes in blood pressure in the medulla oblongata of the central nervous system. A sudden decrease in arterial blood pressure stimulates these cells to activate the sympathetic nervous system, leading to an increase in arterial blood pressure by a mechanism as described above.
Regulation by the Renin–Angiotensin–Aldosterone System [15.6]. This is a hormonal system that controls the basal tone and contractility of the vascular system. Renin is an enzyme secreted by a specialized type of arterial smooth muscle cell in a structure known as the juxtaglomerular apparatus located in the wall of renal arteries. These specialized cells are capable of sensing mechanical stretching signals. A decrease in arterial blood pressure can stimulate these cells to secret renin. Renin is released into the blood and can cleave a plasma protein called angiotensinogen to produce angiotensin I. Angiotensin I can be cleaved again and converted into angiotensin II by another enzyme called angiotensin-converting enzyme, which is produced primarily in the lungs. Angiotensin II can interact with the angiotensin II type 1 receptor in the membrane of vascular smooth muscle cells, activate a G-protein signaling pathway, induce the release of calcium and activation of protein kinases (see page 217 for G-protein-related regulatory mechanisms), and enhance actin–myosin interactions and cell contraction, resulting in an increase in arterial blood pressure (see page 668 of this chapter for the regulation of actin–myosin interaction). At a resting state, angiotensin I and II are kept at a relatively constant level, which is critical to the maintenance of the physiological arterial blood pressure.
Angiotensin II can stimulate the adrenal cortical cells to secret a hormone known as aldosterone. This hormone can act on the tubular system of the kidney to increase the absorption and retention of sodium and water, resulting in an increase in blood volume. The activation of the renin–angiotensin–aldosterone system is a major mechanism that regulates the basal level of the arterial blood pressure. A disorder of this system contributes to the pathogenesis of arterial hypertension (see page 699 of this chapter for pathogenesis of arterial hypertension).
The production of renin is regulated by hormones. A typical hormone is vitamin D. This molecule can difuse through the cell membrane and interact with the nuclear receptor vitamin D receptor. The complex of vitamin D and vitamin D receptor serves as a transcriptional factor and binds to the renin gene, suppressing its expression. Such a mechanism results in a decrease in the level of angitesin II. In experimental models, knockout of the vitamin D receptor is associated with a significant increase in the lelve of serum renin and angiotensin II, which is associated with an increase in the arterial blood pressure (Fig. 15.2).
Characteristics of renin–angiotensin system molecules are presented in Table 15.4.
Regulation by the Vasopressin System [15.1]. Vasopressin is also known as antidiuretic hormone (ADH) and is produced in the posterior pituitary. The release of vasopressin is regulated by baroreceptors and the central vasomotor control system. A decrease in arterial blood pressure stimulates the baroreceptors and central vasomotor system, which in turn activates the posterior pituitary to release vasopressin. Released vasopressin can enhance the contractility of vascular smooth muscle cells and thus increase the arterial blood pressure.
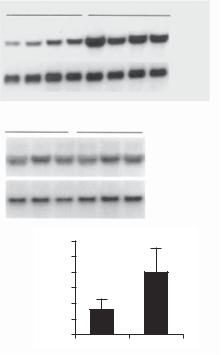
674 VASCULAR REGENERATIVE ENGINEERING
A
+/+ |
–/– |
Renin
35B4
B +/+ |
–/– |
Angiotensinogen
36B4
C |
600 |
* |
|
||
(pg/ml) |
500 |
|
400 |
|
|
300 |
|
|
II |
|
|
200 |
|
|
Ang |
|
|
100 |
|
|
|
|
|
|
0 |
|
|
+/+ |
–/– |
Figure 15.2. Effect of vitamin D receptor (VDR) inactivation on renin expression and plasma Angiotensin II (Ang II) production. (A) Renin mRNA expression in the kidney. Kidney total RNAs were isolated from wild-type (+/+) and VDR−/− (−/−) mice and analyzed by Northern blot. The same membrane was sequentially hybridized with mouse renin and 36B4 (control) cDNA probes. Each lane represents an individual animal. (B) Liver angiotensinogen mRNA expression in wildtype and VDR−/− mice, determined by Northern blot. The membrane was sequentially hybridized with mouse angiotensinogen and 36B4 cDNA probes. Each lane represents an individual mouse.
(C) Plasma Ang II concentrations in wild-type and VDR−/− mice. *P < 0.001 vs. wild-type mice; n = 15 in each group. Reprinted with permission from Li Y C, et al. 1,25-Dihydroxyvitamin D3 is a negative endocrine regulator of the renin-angiotensin system, J Clin Invest 110:229–238, copyright 2002.
VASCULAR DISORDERS
Atherosclerosis
Pathogenesis, Pathology, and Clinical Features [15.7]. Atherosclerosis is a disorder found in the lumen of primarily large and medium-sized arteries and characterized by the progressive development of focal atheroma, a structure containing extracellular matrix, cholesterol, calcium, smooth muscle cells, and macrophages, which are covered with a layer of endothelial cells (Fig. 15.3). Atherosclerosis may be initiated at any age. The formation of atheroma may begin during the childhood. As the development of atheroma
