
Engineering and Manufacturing for Biotechnology - Marcel Hofman & Philippe Thonart
.pdfInitiation, growth and immobilisation of cell cultures of taxus spp.
Murashige, T. and Skoog, F. (1962) A revised medium for rapid growth and bioassays with tobacco tissue cultures, Physiologia Plantarium 15, 473-497.
Nicolaou, H. C., Yang, Z., Liu, J. J., Ueno, H., Nantermet, P. G., Guy, R. K.., Claiborne, C. F., Renaud, J.,
Couladouros, E. A., Paulvannan, K. and Sorensen, E. J. (1994b) Total synthesis of Taxol, Nature 367,
630-634.
Nicolaou, K. C. and Sorensen, E. J. (1996) Classics in Total Synthesis: Targets, Strategies, Methods, VCH,
Weinheim.
Nicolaou, K.C., Dai, W.M. and Guy, R.K. (1994a) Chemistry and biology of Taxol, Angew Chem Int Ed
Engl 33,15-44.
Owen, M.R.L. and Pen, J. (1996) Transgenic Plants: A Production System for Industrial and
Pharmaceutical Proteins, John Wiley & Son Ltd, New York.
Penso, G. (1983) Index plantarum medicinalium totius mundi eurumque synonymorum, Organizzazione
Editorale Medico-Farmaceutica, Milan.
Pestchanker, L.J., Roberts, S.C. and Shuler, M.L. (1996) Kinetics of Taxol production and nutrient use in suspension cultures of Taxus cuspidata in shake flasks and a Wilson-type bioreactor, Enzyme and Microbial Technology 19, 256-260.
Pezzuto, J. M. (1996) Commentary: Plant derived anticancer agents, Biochem. Pharmacol, 53, 121-133. Phillipson, J . D . (1997) Medicinal plants, J. Biol. Edu. 31 (2), 109115.
Pierik, R. L. M. (1987) In Vitro Culture of Higher Plants, Martinus Nijhoff Publishers, Dordrecht.
Principe, P. P. (1996) Monetizing the pharmacological benefits of plants, in M. J. Balick, E. Elisabetsky and S. A. Laird (eds.) Medicinal Plant Resources of the Tropical Forest, Columbia University Press, New York, pp. 191-218.
Seki, M. and Furusaki, S. (1996) An immobilised cell system for Taxol production, Chemitech 26, 41-45. Seki, M., Ohzora, C., Takeda, M. and Furusaki, S. (1997) Taxol (Paclitaxel) production using free and
immobilised cells of Taxus cuspidata, Biotechnology and Bioengineering 53, 214-219.
Shahidi, F., Kolodziejczyk, P. and Whitaker, J.R. (1999) Chemicals Via Higher Plant Bioengineering
(Advances in Experimental Medicine and Biology, 464), Kluwer Academic Publishers, Dordrecht, The Netherlands.
Srinivasan, V., Pestchanker, L., Moser, S., Hirasuna, T.J., Taticek, R.A. and Shulet, M.L. (1995) Taxol production in bioreactors: kinetics of biomass accumulation, nutrient uptake, and Taxol production by cell suspensions of Taxus baccata, Biotechnology and Bioengineering 47, 666-676.
Stephanopoulos, G. N., Aristidou, A. A., and Nielsen, J. (1998) Metabolic Engineering: Principles and Methodologies, Academic Press, San Diego.
Street, H. E. (ed.) (1977) Plant Tissue and Cell Culture, 2nd edition, Blackwell, Oxford.
Strobel, G.A., Stierle, A., and Hess, W.M. (1993) Taxol formation in Yew–Taxus, Plant Science 92, 1-12. Suffness M (ed.) (1995) Taxol Science and Applications, CRC Press Inc. Boca Raton, Florida, USA. Suffness, M. and Pezzuto, J. M. (1991) Assays for cytotoxicity and antitumor activity, in K. Hostettmann
(ed), Methods in Plant Biochemistry, Academic Press, London, Vol. 6, Chap. 4, pp. 71-133.
Vasil, I. K. (1994) Automation of plant propagation, Plant Cell, Tissue and Organ Culture 39, 105-108.
Vidensek, N., Lim, P., Campbell A. and Carlson C. (1990) Taxol content in bark, wood, root, leaf, twig and seedling from several Taxus species, Journal of Natural Products 53, 1609-1610.
Wani, M.C., Taylor, H.L., Wall, M.E., Coggon, P. and McPhail, A.T. (1971) Plant antitumor agents. VI. The isolation and structure of Taxol, a novel antileukemic and antitumor agent from Taxus brevifolia, Journal of America Chemistry Society 93, 2325-2327.
Wheeler, N.C., Jech, K.., Master's S., Bronst, S.W., Alvarado, A.B., Hoover, A.J. and Sander, K..M. (1992) Effects of genetic, epigenetic, and environmental factors on Taxol content in Taxus brevifolia and related species, Journal of Natural Products 55, 432-440.
Wickremesinhe, E.R.M. and Arteca, R.N. (1991) Production of Taxol in callus and cell suspension cultures of Taxus x media Hicksii, Journal of Plant Physiology 96, 96.
Wickremesinhe, E.R.M. and Arteca, R.N. (1993) Establishment of Taxus callus cultures, optimisation of growth and confirmation of Taxol production, Plant Cell Tissue and Organ Culture 35, 181-193.
Wickremesinhe, E.R.M. and Arteca, R.N. (1994) Taxus cell suspension culture s – optimising growth and production of Taxol, Journal of Plant Physiology 144, 183-188.
Widholm, J.M. (1972) The use of fluorescein diacetate and phenosofranine for determining viability of cultured plant cell, Slain Technology 474, 189-194.
447
Chi Wai Tang, Eman Zalat and Ferda Mavituna
Williams, P. D. and Mavituna, F. (1992) Immobilised Plant Cells, in W. F. Fowler and G. S. Warren (eds.)
M. M. Moo-Young (Ed. in Chief) Plant Biotechnology: Comprehensive Biotechnology Second
Supplement, Pergamon Press, Oxford, Chap. 5, pp. 6378.
Witherup, K.M., Look, S.A., Stasko, M.W., Ghiorzi, T.J., Muschik, G.M. and Cragg, G.M. (1990) Taxus spp. Needles contain amounts of Taxol comparable to the bark of Taxus brevifolia – analysis and isolation, Journal of Natural Products 53, 1249-1255.
Wright, C. W. (1995) Natural products in the fight against AIDS, Pharmaceutical Journal 254, 583-587.
Zhiri, A., Jaziri, M., Homes, J., Vanhaelen, M. and Shimomura, K. (1994) Factors affecting the in vitro- rapid germination of Taxus embryos and the evaluation of Taxol content in the plantlets, Plant Cell
Tissue and Organ Culture 39, 261-263.
448
EFFECTIVE BIOFUEL PRODUCTION BY AN INTELLIGENT BIOREACTOR
HIDEKI FUKUDA1, AKIHIKO KONDO2, AND HIDEO NODA3
1Division of Molecular Science, Graduate School of Science and
Technology, Kobe University, Japan
2Department of Chemical Science and Engineering, Faculty of Engineering, Kobe University, Japan
3Kansai Chemical Engineering Co., Ltd., Japan
Abstract
With the aim of contributing to efforts to solve global energy and environmental problems, a joint research project— Effective Biofuel Production by an Intelligent
Bioreactor — has been set up with participants representing several universities, research institute, and industrial companies. Biofuel obtained from biomass resources is seen as an important source of ‘clean energy’ by virtue of features such as its biodegradability and low carbon and sulphur dioxide contents. By utilising an
‘intelligent’ bioreactor containing immobilised ‘arming cells’, it is expected that practical biofuel production can be achieved at a considerably lower cost than with conventional processes.
1. Introduction
The growing seriousness of the global energy problem and environmental pollution are substantially increasing the importance of using value-added products from biomass resources as biofuel . Biofuel produced from biomass, such as biodiesel or ethanol, have two significant advantages:
•biodegradability,
•better-quality exhaust gas emissions.
In addition, the atmospheric levels of carbon and sulphur dioxide are not raised because the organic carbon of biofuel is produced by photosynthesis in plants.
In current research on biodiesel production, rapeseed esters are being investigated in Europe [1] and palm oil esters in Malaysia [2]. Soybean oil esters also feature prominently as a potential diesel fuel alternative [3], and there is a wide range of ongoing research in this area. In fact, in recent years biodiesel has been produced from waste edible oil on a pilot scale in Japan.
449
M. Hofman and P. Thonart (eds.), Engineering and Manufacturing for Biotechnology, 449–455. © 2001 Kluwer Academic Publishers. Printed in the Netherlands.
Hideki Fukuda, Akihiko Kondo, and Hideo Noda
Though efficient in terms of reaction yield and time, the chemical approach to synthesising alkyl esters [4-6] from triglycerides has several drawbacks, including difficulties in the recovery of glycerol, the need for removal of salt residue, and the energy-intensive nature of the process. On the other hand, the use of biocatalysts allows for the synthesis of specific alkyl esters, easy recovery of glycerol, and transesterification of glycerides with high amounts of free fatty acids. In addition, this process can further be used to synthesise other value-added products, including biodegradable lubricants and additives for fuels and lubricants. However, it has not thus far been adopted industrially because of its high cost.
Over the past two decades, there has been considerable interest and activity in the production of ethanol for use as a fuel by fermentation. Sugar materials such as molasses, sugar cane, and sulphite waste liquor have been mainly utilised for ethanol production, since complicated saccharifying or lignin degrading pretreatment processes are required when starch or cellulosic materials are used. There is thus a need for a novel bioprocess by which ethanol can be produced directly from starch or cellulolytic materials without the necessity for any pretreatment.
The purpose of the project described here, which has been realised with the support of the New Energy and Industrial Technology Development Organisation (NEDO) of Japan, is to establish a practical bioprocess for biodiesel and/or ethanol production from biomass resources based on two key technologies as elucidated below.
2. Key technologies for biofuel production
2.1 INTELLIGENT BIOREACTOR USING IMMOBILIZED YEAST CELLS
The past two decades have seen rapid developments in the use of enzymes as catalysts for industrial, analytical, and medical purposes, leading to the appearance of a new field of research known as enzyme technology. With the aim of making their use more convenient, there is now considerable interest in the direct utilisation of immobilised cells as a means of catalysis.
Passive immobilisation using porous biomass support particles (BSPs) has been successfully applied in fundamental research involving a wide variety of microbial, animal, and plant cell systems [7].
Recently, the author's laboratory succeeded in effective enzyme production using cells of a flocculent yeast immobilised within BSPs [8], as well as in the development of a novel bioconversion process using immobilised recombinant flocculent yeast cells carrying an enzyme fusion gene between rat P4501A1 and yeast NADPH-P450 reductase [9]. Of particular interest was the finding that recombinant cells immobilised within the BSPs not only exhibited significant expression of the fused enzyme, but a high proportion of plasmid-carrying cells was maintained. This contrasted with a much lower proportion among freely suspended cells released from the BSPs, in which no expression of the enzyme could be detected. It was thus apparent, as illustrated in Figure 1, that only highly expressing yeast cells were spontaneously immobilised within the BSPs. A bioreactor packed with such cells, which we have termed an ‘intelligent’
450
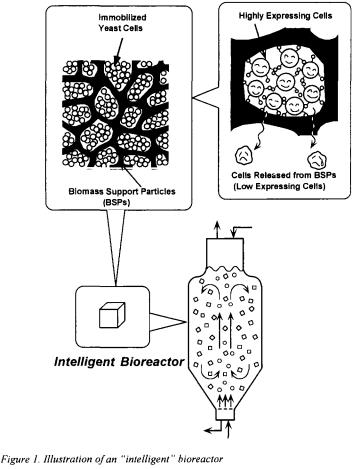
Effective biofuel production by an intelligent bioreactor
bioreactor, possess excellent potential either for the production of a wide variety of useful compounds or for use in various bioconversion reactions.
2.2 IMMOBILIZING PROTEINS ON THE SURFACE OF YEAST CELLS
The display of heterologous proteins on the cell surface of microorganisms is an important objective for many applications in microand molecular biology. In contrast to existing examples of surface expression on cells of eukaryotes, in the case of yeast cells, i.e. Saccharomyces sp., surface-expressed proteins become covalently linked to glucan in the cell wall instead of being linked to the plasma membrane, thereby rendering them resistant to extraction.
Recently, one group involved in the current project succeeded in constructing of a novel starch-utilising ‘cell surface-engineered’ yeast by displaying an amylolytic enzyme on the cell wall of S. cerevisiae [10]. Thus, the cell surface can be regarded as a
451
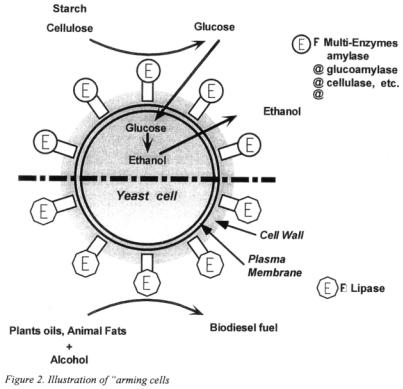
Hideki Fukuda, Akihiko Kondo, and Hideo Noda
new target for bestowing additional characteristics of metabolic reactions, and effective multipurpose yeast cells suitable for biofuel production, which have been termed ‘arming” cells’, can be created (illustrated in Figure 2).
3. Outline of ongoing research
To establish a practical process for biodiesel or ethanol production, research efforts will mainly focus on the development of (i) highly functional yeast cells, (ii) an intelligent immobilised bioreactor system, and (iii) an optimal control system in conjunction with an efficient monitoring system.
3.1 DEVELOPMENT OF HIGHLY FUNCTIONAL YEAST CELLS
The development of highly functional yeast cells that are effective for the production of biofuel should be able to realised using genetic engineering. Possible techniques include the introduction of enzyme-producing genes into flocculent yeast strains and/or of flocculation genes cloned from Saccharomyces strains [11] into enzyme-producing nonflocculent yeast strains both with and without cell-surface engineering.
452
Effective biofuel production by an intelligent bioreactor
In addition, techniques suitable for the screening and cloning of effective enzyme genes and protein conformation stabilisation are required. To stabilise the conformation of biofuel-producing enzymes and to generate strains that have higher activities than native enzymes, our laboratory will try to reveal the factors that affect the stability of the secondary structure, such as the alpha-helix, by structural analysis of synthetic model peptides and theoretical calculation. We will also endeavour to isolate thermostable proteins. The following researches are currently in progress.
•Construction of arming yeast cells
• Screening and cloning of lipase enzyme for biodiesel production [12-13]
•Stabilisation of protein conformation
3.2 DEVELOPMENT OF AN INTELLIGENT BIOREACTOR SYSTEM
Optimising the design of bioreactors for use in immobilised cell processes depends on several factors. The nature of the mass transfer requirement plays an important part, while the immobilisation method and particle characteristics must also be considered. In addition, the type of substrate, operational requirements, and hydraulic and economic considerations will also influence the design. Thus, the application of an intelligent bioreactor system suitable for biofuel production in industrial processes requires— mainly involving cell physiology, BSP materials and mass transfer within the bioreactor—to elucidate such properties. Researches in the following areas are now ongoing.
•Development of novel BSPs for engineered yeast cells
•Analysis of the suitability of bioreactors
•Construction of a novel intelligent bioreactor
3.3 DEVELOPMENT OF AN OPTIMAL CONTROL SYSTEM I N CONJUNCTION WITH EFFICIENT MONITORING
To achieve efficient online monitoring, a micro-sampling device, advanced measurement methods based on immunotechnology, and a novel flow injection analysis
(FIA) system will be developed. We will also endeavour to formulate a model of mass transfer in immobilised cells and of the related biochemical reaction in order to develop an optimal control system for a bioreactor with immobilised cells. A simulator will be constructed to examine the responses of state variables to several kinds of control action. Researches are at present under way on the following topics.
•Modelling of the mass transfer and biochemical reaction in a bioreactor
• Construction of a simulator for use in the study of responses to control actions
•Construction of a novel intelligent control system as illustrated in Figure 3.
453
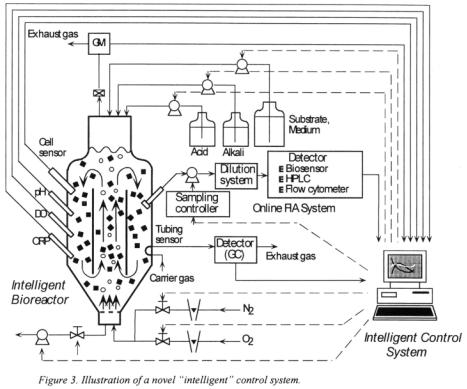
Hideki Fukuda, Akihiko Kondo, and Hideo Noda
4. Conclusion
The joint research project—Effective Biofuel Production by an Intelligent Bio-reactor— which has been set up with the support of the New Energy and Industrial Technology Development Organisation (NEDO) of Japan, aims to establish a practical bioprocess for biofuel production based on two key technologies: an intelligent bioreactor and arming cells. Twenty-two scientists with backgrounds in biochemical engineering, enzyme engineering, biology, chemistry, and other relevant fields, working in three universities, two research institutes, and four industrial companies, are participating in the research. It is expected that the project will run for three years from 1998 to 2001, and if successful could significantly contribute to alleviating global energy and environmental problems.
References
1. Cvengros, J., and Cvengrosova, Z. (1994), J. Am. Oil Chem. Soc. 71, 1349-1352 2. Masjuki, H. H, and Sapuan, A.M. (1995) J. Am. Oil Chem. Soc.72, 609-612
3.Goering, C.E., and Fry, B.G. (1984) J. Am. Oil Chem. Soc.61, 1627-1632
454
Effective biofuel production by an intelligent bioreactor
4 Ali, Y, and Hanna, M.A. (1994) Bioresource Technol. 50, 153-163
5.Freedmann, B., Pryde, E.H., and Mounts, T.L. (1984) J. Am. Oil Chem. Soc. 61, 1638-1643
6.Lee, I.Johnson, L.A., and Hammound, E.G. (1995) J. Am. Oil Chem. Soc. 72, 1155-1160
7.Fukuda, H. (1995) BIOREACTOR SYSTEM DESIGN, Marcel Dekker, Inc.,New York, pp.339-375
8.Furuta, H., Arai, T., Hama, N., Shiomi, N., Kondo, A., and Fukuda, H. (1997) J. Ferment Bioeng. 84, 169-171
9.Liu, Y., Kondo, A., Ohkawa, H., Shiota, N., and Fukuda, H. (1998) Biochem. Eng. J. 2, 229-235
10.Murai, T., Ueda, M., Yamamura, M., Atomi, H., Shibasaki, Y., Kamasawa, N., Osumi, M., Amachi, T., and Tanaka, A. (1997) Appl. Microbiol. Biotechnol., 63,1362-1366
11.Watari, J., Takata, Y., Ogawa, M., Murakami, J., and Koshino, S. (1991) Agric. Biol. Chem. 55, 15471552
12.Shimada, Y., Watanabe, Y., Samukawa, T., Sugihara, A., Noda, H., Fukuda, H., Tominaga, Y. (1999) J.
Am. Oil Chem. Soc. 76, 789-793
13.Kaieda, M., Samukawa, T., Matsumoto, T., Ban, K., Kondo, A., Shimada, Y., Noda, H., Nomoto, F.,
Ohtsuka, K., Izumoto, E., and Fukuda, H. (1999) J. Biosci. Bioeng. 88, 627-631
455
PART IX
PATENTS AND LICENSES