
The Physics of Coronory Blood Flow - M. Zamir
.pdf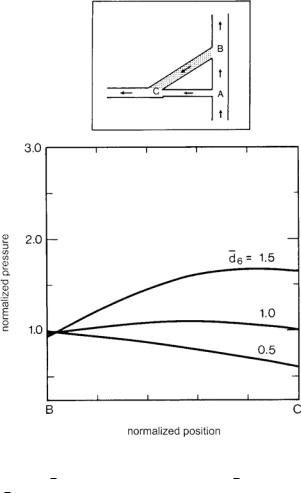
380 10 Dynamic Pathologies
Fig. 10.4.3. Pressure distribution in a coronary bypass graft, created by wave reflections when the diameter of the graft is the same as that of the native coronary artery (curve labeled d6 = 1), when it is 50% larger (d6 = 1.5), and when it is
50% smaller (d6 = 0.5). A graft of smaller diameter produces a pressure di erence between the two ends of the graft that is more favorable to flow from the aorta to the coronary artery. From [1].
If the elasticity of the bypass graft is the same as that of the native coronary arteries, it is found that wave reflections from the three vascular junctions involved have the net e ect of creating a pressure di erence within the bypass graft which is positive in the desired flow direction, as shown in Fig. 10.4.2. In other words, as a result of wave reflections the pressure at junction C in that figure is higher than the pressure at junction B. This pressure di erence by itself would cause the flow to go from the coronary network to the aorta instead of the reverse, for which the bypass is intended. But, of course, this pressure di erence is not present by itself, it represents merely a perturbation
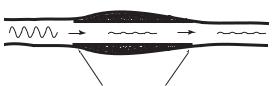
10.5 Wave Propagation Through a Coronary Stent |
381 |
on the main pressure di erence between the aorta and the coronary network. Nevertheless, it is a perturbation with an adverse e ect on the main pressure di erence driving the flow. The analysis shows that if the bypass graft is chosen to be sti er (less elastic) than the native coronary artery, this adverse e ect can in fact be diminished as shown in Fig. 10.4.2. In the clinical setting the choice of a bypass graft is usually between a saphenous vein graft and a mammary or other arterial graft [57, 82, 30], and it is usually made on surgical grounds. From the perspective of the dynamics of the flow, the results suggest that an arterial or a synthetic graft that is sti er than a saphenous vein would be preferable. Arteries generally have a higher modulus of elasticity than do veins [34, 70, 71], and arterial grafts are reported to have better performance than vein grafts [30].
Similarly, the pressure di erence between the two ends of the bypass graft, created by wave reflections from all junctions, is found to be more favorable to flow from the aorta to the coronary vessel when the diameter of the bypass graft is smaller than the native coronary artery, and more adverse when the reverse is true, as shown in Fig. 10.4.3. Common wisdom in coronary bypass surgery is to use a graft of larger diameter than that of the native coronary artery [57, 82, 72, 58], clearly, that wisdom is being based on considerations of steady flow through the bypass system.
10.5 Wave Propagation Through a Coronary Stent
The placement of a coronary stent within a diseased coronary artery, like the placement of a coronary bypass graft, is a practice based largely on considerations of steady flow. The stent functions by sca olding a diseased coronary artery to compress any lesions protruding into the lumen, thus keeping the vessel open for blood flow as illustrated schematically in Fig. 10.5.1. The basis of this practice is perfectly valid in the context of steady flow where an
rigid
elastic |
elastic |
wave reflection sites
Fig. 10.5.1. From the standpoint of the dynamics of pulsatile flow, a coronary stent represents a rigid tube segment in an otherwise elastic vessel. Because the impedance of the rigid segment is much higher than that of the elastic segments to which it is attached, the propagating wave is blunted within the stent as illustrated schematically, and because of impedance mismatch at the two ends of the stent, two wave reflection sites are created there.

382 10 Dynamic Pathologies
|
1.1 |
|
|
|
|
|
|
1.05 |
|
|
|
|
a |
|
|
|
|
|
|
|
|
1 |
|
|
|
|
|
|
1.05 |
|
|
|
|
|
|
1 |
|
|
|
|
b |
|
|
|
|
|
|
|
|
1.05 |
|
|
|
|
c |
|
1 |
|
|
|
|
|
|
|
|
|
|
|
|
| |
1.05 |
|
|
|
|
|
amplitude |
|
|
|
|
|
|
1 |
|
|
|
|
d |
|
|
|
|
|
|
||
1.05 |
|
|
|
|
e |
|
pressure |
1 |
|
|
|
|
|
|
|
|
|
|
||
1.05 |
|
|
|
|
|
|
| |
|
|
|
|
|
|
|
|
|
|
|
f |
|
|
1 |
|
|
|
|
|
|
|
|
|
|
|
|
|
1.05 |
|
|
|
|
g |
|
|
|
|
|
|
|
|
1 |
|
|
|
|
|
|
1.05 |
|
|
|
|
h |
|
|
|
|
|
|
|
|
1 |
|
|
|
|
|
|
0.95 |
|
|
|
|
|
|
0.9 |
2 |
4 |
6 |
8 |
10 |
|
0 |
|||||
|
|
|
|
position |
|
|
Fig. 10.5.2. Normalized pressure distribution along a coronary artery of length 10 cm, with a stent, shown in bold, of length 1 cm and placed at di erent position along the vessel, position ‘0’ being the entrance to and ‘10’ being the exit from the artery. When the stent is placed near the entrance of the native vessel it has the e ect of producing a pressure distribution (curve a) with a small favorable pressure di erence between entrance and exit from the vessel, but this pressure di erence becomes increasingly larger as the position of the stent is moved closer to the exit end of the vessel (curves b − h). From [2].
open vessel is clearly better than an obstructed one, and several studies have examined various mechanical and other aspects of coronary stents within this context [67, 176, 138, 232, 62, 89, 56, 149, 200, 35, 140].
But in the context of pulsatile flow in elastic tubes a number of additional issues must be addressed. The stent is usually made of a steel mesh with high radial strength [171, 154, 50], and it is found that soon after the stent is implanted the open mesh fills with growing tissue and the stent fuses with the vessel wall [150]. Thus, the combination of stent and vessel wall together become a rigid segment within an otherwise elastic vessel, which presents a considerable change in local impedance within the vessel. Because the stented segment is considerably more rigid than the upstream (proximal) and down-
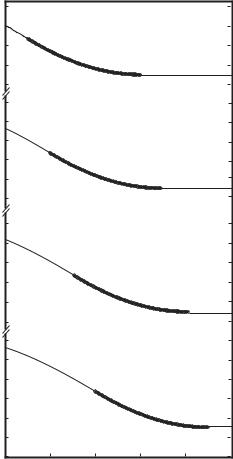
10.5 Wave Propagation Through a Coronary Stent |
383 |
|
1.15 |
|
|
|
|
|
|
1.1 |
|
|
|
|
|
|
1.05 |
|
|
|
|
|
|
1 |
|
|
|
|
a |
|
|
|
|
|
|
|
|
0.95 |
|
|
|
|
|
|
1.2 |
|
|
|
|
|
|
1.15 |
|
|
|
|
|
|
1.1 |
|
|
|
|
|
|
1.05 |
|
|
|
|
|
|
1 |
|
|
|
|
b |
| |
|
|
|
|
|
|
|
|
|
|
|
|
|
amplitude |
0.95 |
|
|
|
|
|
1.2 |
|
|
|
|
|
|
|
|
|
|
|
|
|
| pressure |
1.15 |
|
|
|
|
|
1.1 |
|
|
|
|
|
|
1.05 |
|
|
|
|
|
|
|
|
|
|
|
|
|
|
1 |
|
|
|
|
c |
|
|
|
|
|
|
|
|
0.95 |
|
|
|
|
|
|
1.2 |
|
|
|
|
|
|
1.15 |
|
|
|
|
|
|
1.1 |
|
|
|
|
|
|
1.05 |
|
|
|
|
|
|
1 |
|
|
|
|
d |
|
|
|
|
|
|
|
|
0.95 |
|
|
|
|
|
|
0.9 |
2 |
4 |
6 |
8 |
10 |
|
0 |
|||||
|
|
|
position |
|
|
|
Fig. 10.5.3. Normalized pressure distribution along a coronary artery of length 10 cm, with a stent, shown in bold, of length 5 cm and placed at di erent position along the vessel, position ‘0’ being the entrance to and ‘10’ being the exit from the artery. When the stent is placed near the entrance of the native vessel it has the e ect of producing a pressure distribution (curve a) with a significant favorable pressure di erence between entrance and exit from the native vessel, and this di erence becomes yet larger as the position of the stent is moved closer to the exit end of the vessel (curves b − d). From [2].
384 10 Dynamic Pathologies
stream (distal) segments to which it is attached, there is a change in impedance at the two junctions between the stented and non-stented segments and hence the two junctions will act as wave reflection sites (Fig. 10.5.1). These issues have only recently been examined in full elsewhere [2], and the essence of the results is presented below, omitting the analytical details.
Of particular interest is the e ect of a coronary stent on the pressure distribution within the vessel in which it is placed. As in the previous section, the pressure distribution in question is only that arising as a result of wave reflections, and it is normalized such that in the absence of wave reflections, which means in the absence of the stent, the pressure distribution would be uniform at a normalized value of 1.0. Any departure from this uniform distribution can thus be attributed entirely to the presence of the stent.
In general it is found that a stent has the e ect of producing a pressure distribution that is actually favourable for flow in the native vessel, with the pressure being higher at entrance to the vessel than it is at exit. The extent of this e ect depends on two main parameters: the length of the stent in relation to the length of the native vessel, and the position of the stent within the length of the native vessel. The results show that a relatively short stent placed near the entrance of the native vessel has the smallest e ect and produces a pressure distribution with the smallest pressure di erence between the two ends of the vessel. This e ect becomes increasingly more significant, however, as the stent is moved closer to the exit end of the native vessel, as shown in Fig. 10.5.2.
In the case of a relatively long stent it is found that the pressure distribution it produces and the associated pressure di erence are fairly significant even when the stent is placed near the entrance of the native vessel, and it becomes considerably more so as the stent is moved closer to the exit end of the vessel, as shown in Fig. 10.5.3.
10.6 Sudden Cardiac Death
A principal theme of this book has been to highlight the dynamics of the coronary circulation and to show the central role that these dynamics can play in coronary blood flow. Nowhere does this theme find a better application than in the phenomenon of sudden cardiac death, and it is therefore appropriate that this subject be discussed in the last chapter of the book. The material to be presented is indeed no more than a brief discussion, based on an extensive review by Osborn [158].
Sudden cardiac death is death due to heart failure in which the timing of the failure is sudden or unexpected [158]. It is a major public health problem, being the leading cause of death in the industrialized world, claiming more than one thousand lives per day in the United States alone [158].
The cause of sudden cardiac death in all cases is a precipitous fall in cardiac output to levels that can no longer sustain cerebral or cardiac function. The
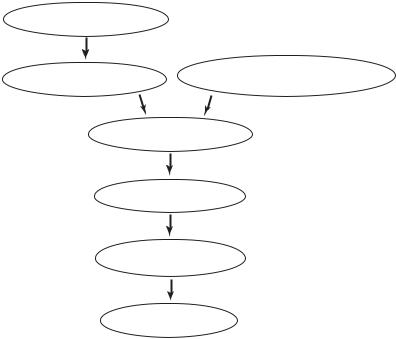
10.6 Sudden Cardiac Death |
385 |
fall in cardiac output is usually associated with a disruption in heart rhythm in one form or another: ventricular fibrillation, tachycardia, bradycardia, and the like. Common causes of disruption in heart rhythm include myocardial infarction or ischemia and cardiomyopathy, all of which act to disrupt the “electrical uniformity” of the myocardium. Other causes include disruptions in neural activity and environmental or psychological stresses such as startle, fright, anger, and excitement. Coronary artery disease, which is found in most cases of sudden cardiac death in adults, is generally seen to be a precursor to conditions of myocardial ischemia and infarction leading to a disruption in heart rhythm.
Thus, the sequence of events leading to sudden cardiac death according to current theory is as shown schematically in the form of a flow chart in Fig. 10.6.1.
coronary artery disease
myocardial |
disruption in neural activity, |
|
environmental/psychological stress |
||
ischemia/infarction |
||
|
disruption in heart rhythm
fall in cardiac output
fall in coronary blood supply
HEART FAILURE
Fig. 10.6.1. A summary of current theory on the sequence of events leading to heart failure in sudden cardiac death.
A di culty with this sequence of events is that it proposes that a fall in cardiac output is followed by a fall in coronary blood flow. The mechanism for this sequence is not clear, if indeed possible. If the fall in cardiac output leads to a drop in aortic pressure, then it is well known that autoregulation of coronary blood flow ensures that this does not lead to a fall in coronary
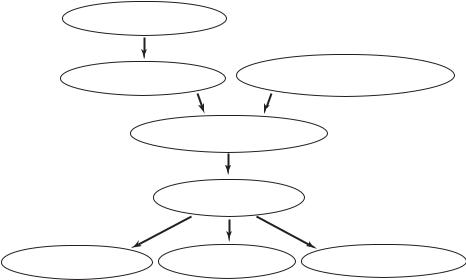
386 10 Dynamic Pathologies
blood flow. As discussed briefly in Section 1.2, in the absence of this protective regulatory feature of the coronary circulation the system would behave as an unstable “positive feedback” system in which a fall in cardiac output would lead to a fall in coronary blood flow and to a further fall in cardiac output and a further fall in coronary blood flow, etc. It is known that the coronary circulation as a feedback system does not behave in this way, as discussed in Section 1.2.
Thus, a fall or disruption in coronary blood flow is unlikely to be the result of a fall in cardiac output because this is inconsistent with the regulatory mechanisms of the system. Indeed, the reverse is more consistent with the regulatory mechanisms of the system and is therefore more likely to be the case. A fall in cardiac output is more likely to be the consequence rather than the cause of a fall in coronary blood flow in the sequence of events leading to sudden cardiac death.
We have seen throughout this book that a fall in coronary blood flow may result from a disruption in the dynamics of the coronary circulation, which in turn can result from coronary artery disease, cardiac damage, or a disruption in neural activity. The fall in coronary blood flow then leads to a fall in cardiac output, to possible disruptions in heart rhythm, and to heart failure. This sequence of events is shown schematically in Fig. 10.6.2.
coronary artery disease
myocardial |
disruption in neural activity, |
|
environmental/psychological stress |
||
ischemia/infarction |
||
|
disruption in the dynamics of the coronary circulation
fall in coronary blood supply
fall in |
HEART FAILURE |
disruption in |
|
cardiac output |
heart rhythm |
||
|
Fig. 10.6.2. A summary of proposed theory on the sequence of events leading to heart failure in sudden cardiac death.
10.7 Broken Heart Syndrome |
387 |
10.7 Broken Heart Syndrome
As this book goes to press, an issue of The New England Journal of Medicine flags one of its leading articles [207] with the banner “Broken Heart Syndrome” and a brief news item to the e ect that “Sudden emotional distress
... can sometimes produce severe transient left ventricular dysfunction. This stress-induced cardiomyopathy appears to be a form of myocardial stunning associated with marked sympathetic stimulation.” Both the banner and news item provide a particularly fitting epilogue to this chapter, and, indeed, to the principal tenet of this book that dynamic pathologies in the coronary circulation can produce calamities equal to those produced by structural pathologies.
The authors of the article report in more detail that “.. a unique pattern of transient myocardial dysfunction can occur after severe emotional stress. Patients with this syndrome have evidence of exaggerated sympathetic activation, with plasma catecholamine levels several times those in ageand sexmatched patients with Killip class III myocardial infarction. Although our data suggest that catecholamines may be central to the mechanism of stressrelated myocardial stunning, a more complete understanding of the pathogenesis of this syndrome awaits further research.” Three possible mechanisms are discussed: epicardial coronary arterial spasm, microvascular spasm, and direct myocyte injury. It is concluded that “Emotional stress can precipitate severe, reversible left ventricular dysfunction in patients without coronary disease. Exaggerated sympathetic stimulation is probably central to the cause of this syndrome.”
As mentioned in Section 10.6, psychological stresses such as startle, fright, anger and excitement, have been identified to cause disruptions in heart rhythm associated with sudden cardiac death [158]. Emotional stresses that have been identified in conjunction with the broken heart syndrome include death in a family, accident, fear and surprise [207]. These emotional stresses may clearly have some features in common with the above psychological stresses, but the key to the new findings is that the broken heart syndrome is marked by highly elevated plasma levels of catecholamines and “stress-related neuropeptides”. These agents are known to control parameters that a ect the dynamics of the coronary circulation as they produce changes in vascular calibers and vascular resistance. The broken heart syndrome may therefore be considered another example of a disruption in, indeed an insult to, the dynamics of the coronary circulation leading to a fall in coronary blood supply and to a fall in cardiac output, as outlined schematically in Fig. 10.6.2. Thus, both the sudden cardiac death syndrome and the broken heart syndrome o er examples of dynamic pathologies in the coronary circulation, although an important distinction of the latter is that it is found to be transient and reversible [207].
One of the most important aspects of dynamic pathologies is that, unlike structural pathologies, they do not leave a “footprint” after they have resolved or produced their damage. Following sudden cardiac death, indeed following
388 10 Dynamic Pathologies
any death attributed to heart disease, only structural pathologies can usually be found and they become the focus of attention. Any dynamic pathologies involved are not in evidence because they are no longer at play. The broken heart syndrome on the other hand, with the distinction of being transient, reversible and free from coronary artery disease, o ers an opportunity to detect dynamic pathologies while they are at play and in “pure form” because the syndrom is not associated with coronary artery disease.
10.8 Summary
Coronary blood flow can be disrupted by dynamic pathology in the same way that it can be disrupted by vascular pathology. Any disruption in the exquisite harmony between the pulsatile rhythm of the input pressure driving the flow, the pulsatile rhythm of the contracting myocardium muscle and its e ect on coronary vessels imbedded within the muscle tissue, and the combination of wave propagation and wave reflections within the coronary vascular tree, can produce dynamic pathology that disrupts coronary blood flow.
Changes in the architecture or mechanical properties of coronary vasculature have significant dynamical consequences and may move the system away from its magic norm or norms, which are modes of operations in which the dynamics of the system appear particularly simplified or optimal in some sense. Every aspect of the distribution and propagation of pressure and flow within the tree model is a ected. And while the dynamics of the coronary circulation are far more complicated than those of the 11-level tree model, it is reasonable to speculate that changes in the architecture or mechanical properties of the coronary arteries, whether brought on by neural or humoral agents, under normal or pathological conditions, will have significant e ects on the dynamics of coronary blood flow.
Coronary flow reserve and vascular restructuring are two mechanisms by which the coronary circulation can increase coronary blood flow. The trigger for the first is an increase in oxygen consumption by the heart muscle and its response time is of the order of seconds, while the trigger for the second is repeated episodes of hypoxia and momentary ischemia and its response time is at best of the order of weeks but more likely months or years. It would thus seem that coronary flow reserve is designed to deal with acute shortfalls in coronary blood flow, as at the onset of increased physical activity, while vascular restructuring is designed to deal with chronic shortfalls in coronary blood flow, as under the progression of obstructive coronary artery disease. The conundrum of coronary flow reserve is that it may “mask” the gradual shortfall in coronary blood flow at the initial stages of coronary heart disease and thereby prevent the mechanism of vascular restructuring from being triggered. At the latter stages of the disease the capacity of coronary flow reserve is largely depleted and the mechanism of vascular restructuring may not have the time required for its slow course of action. Physical conditioning
10.8 Summary |
389 |
may forestall this course of events by deliberately and regularly triggering the mechanism of vascular restructuring while coronary flow reserve is still intact.
The placement of a coronary bypass graft is usually viewed in terms of providing a conduit for steady flow through the graft. If the pulsatile nature of the flow and the elasticity of the vessels are considered, the e ects of wave propagation and wave reflections produce a pressure di erence between the two ends of the graft that is more favorable to flow in the desired direction through the graft when the graft is sti er than the native coronary artery and when the diameter of the graft is smaller than that of the native coronary artery.
Because of impedance mismatch and consequent wave reflections, the placement of a stent within a coronary artery has the e ect of producing a pressure distribution with a favorable pressure di erence within the native coronary artery such that the pressure at entrance to is higher than that at exit from the artery. This e ect is least significant when the stent is small and is placed near the entrance end of the coronary artery, and most significant when the stent is large and is placed near the exit end. From a clinical standpoint the first of these scenarios produces the least disturbance to flow and possibly a reduced chance of restenosis, while the reverse is true in the second scenario.
In the course of events leading to sudden cardiac death a fall in coronary blood supply is more likely to be the cause rather than the consequence of a fall in cardiac output. Because of the sudden and unexpected timing of the phenomenon, the fall in coronary blood supply may be caused by a disruption in the dynamics of coronary blood flow as discussed in this and previous chapters.
Broken heart syndrome, where transient and reversible ventricular dysfunction is observed in patients without coronary artery disease, may be another example of a disruption in the dynamics of the coronary circulation, that is, another example of a dynamic pathology. Furthermore, while in sudden cardiac death the dynamic pathology is usually coupled with structural pathologies, in broken heart syndrome, where the e ects are transient, reversible and free from coronary artery disease, the dynamic pathology is in “pure form”.