
Biomedical EPR Part-B Methodology Instrumentation and Dynamics - Sandra R. Eaton
.pdf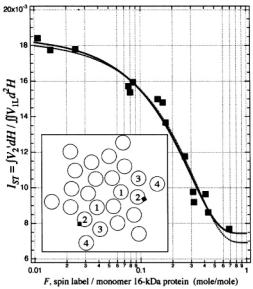
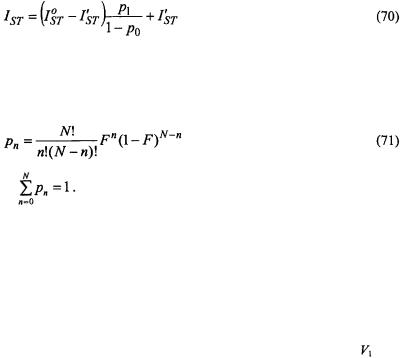
SATURATION TRANSFER SPECTROSCOPY |
353 |
Figure 14 gives the integrated ST-EPR intensity (normalised to number of spins) of the vacuolar ATPase proteolipid subunit, as a function of fractional spin labelling with maleimide on the single cysteine (Páli et al., 1999). Alleviation of saturation by spin-spin interaction causes the progressively decreasing intensity with increasing labelling level. If it is assumed that oligomers containing more than one spin-labelled monomer contribute an intensity
that is reduced relative to the value
for an isolated monomer, then the net normalised ST-EPR intensity is:
where is the probability that n monomers are labelled in an oligomer composed of N monomers. For a statistical distribution of labelling, the binomial distribution holds and the labelling probabilities are:
where
The experimental dependence in Fig. 14 is reasonably well described by Eqs. 70, 71 with N = 6. Using N as a non-linear fitting parameter results in a value of N= 6.6 ± 0.7, consistent with a hexameric assembly of the 16-kDa proteolipid subunit. This is the state of oligomerisation that is found in twodimensional crystalline preparations of the 16-kDa protein (Holzenburg et al., 1993).
In the case of trimer formation in the membrane assembly of annexin XII, spin labels on adjacent monomers are sufficiently close that a binomial
distribution is observed |
in the |
broadening of the conventional |
EPR |
lineshapes (Langen et al., |
1998). |
Non-linear rapid-passage EPR approaches, |
as described here, are more versatile in so far as they are sensitive to much weaker spin-spin interactions. This is likely to be the usual case with large integral oligomeric proteins, hence requiring progressive saturation or out- of-phase methods rather than conventional in-phase EPR.
9.2.3Spin-spin interactions: double labelling
Spin-spin interactions between different spin-labelled species in a double-labelled system are detected as a decrease in the out-of-phase spectral intensity below that predicted from the weighted sum of the two
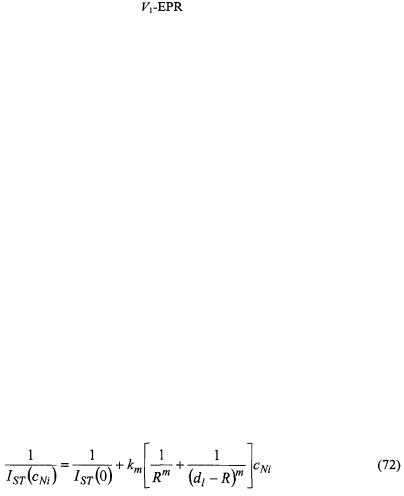
354 DEREK MARSH ET AL.
single-labelled systems. The strength of the interaction is a measure of the mutual accessibility of the two labelled species. The technique is most usefully applied to spin-labelled lipids and spin-labelled proteins in membranes. The vertical location in the membrane of the spin-labelled protein residue is determined relative to spin labels systematically stepped down the lipid chain. The depth in the membrane of the DCCD-reactive glutamate that is essential for proton translocation by the 16-kDa V-ATPase proteolipid was mapped in this way (Páli et al., 1999). More importantly, the double-labelling ST-EPR experiment demonstrates that the essential glutamate is exposed to the lipid, as is required by the rotary mechanism proposed for proton transport by stepwise interaction with the Vph1p subunit
of the V-ATPase. |
|
Progressive saturation |
was used with double-labelling to |
demonstrate the deep penetration of the N-terminal section of the haem-less cytochrome c precursor, apocytochrome c, into negatively charged lipid bilayer membranes (Snel and Marsh, 1994). The spin-labelled holoprotein, cytochrome c, by contrast, showed strongest spin-spin interaction with a spin label attached to the phospholipid headgroup, at the membrane surface, as expected for a classical peripheral membrane protein.
9.3Paramagnetic Relaxation Enhancements
Paramagnetic species used to induce spin-label relaxation enhancement are mostly paramagnetic ions, their complexes and molecular oxygen. Generally, such measurements yield details on spin-label accessibility, penetration profiles of relaxants and, in the case of purely dipolar interactions, molecular separations.
9.3.1Dipolar relaxation: distance measurements
Figure 15 gives the dependence of the paramagnetic relaxation enhancement by aqueous ions on position, n, of the spin label in the lipid chain in gel-phase bilayer membranes (Páli et al., 1992). Because the lipid chains are frozen, the
ions do not penetrate the gel-phase membranes and spin-label relaxation is by distance-dependent magnetic dipole-dipole interaction. As seen from the inset, the reciprocal ST-EPR integral intensity depends linearly on concentration,
of
ions. For a static dipolar mechanism (see Eqs. 56, 57), we have:
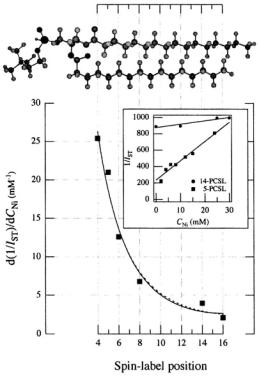
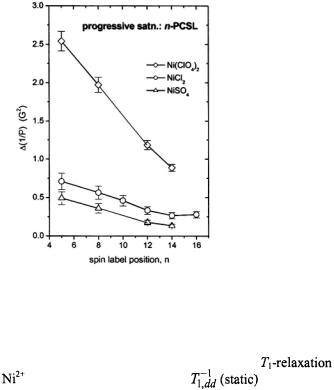
356 |
DEREK MARSH ET AL. |
9.3.2Penetration profiles of paramagnetic ions and relaxation mechanisms
The extent of penetration of paramagnetic ions into fluid lipid membranes depends strongly on the anionic counterion, or complexing ligand. Fig. 16 shows membrane penetration profiles of the sulphate, chloride and perchlorate salts of divalent nickel. The spin-lattice relaxation enhancements of spin labels in the lipid chains that are obtained from progressive saturation are in the order
This is the order of the octanol partition coefficients multiplied by the spin exchange rate constants determined in methanol (Livshits et al., 2001). Heisenberg exchange with ion pairs dissolved in the fluid membrane therefore makes a major contribution to the relaxation enhancement of the spin-labelled lipids.
Figure 16. Dependence of the relaxation enhancement, in progressive saturation EPR (see Eq. 37) on spin label position, n, in the sn-2 chain of phosphatidylcholine for fluid dimyristoyl phosphatidylcholine membranes (T= 39°C) in the presence of 30 mM
or
(Livshits et al., 2001).
Estimation of the possible dipolar contribution to |
|
|
enhancement by |
ions requires integration of |
over the ion |
distribution in the membrane (Livshits et al., 2001). Relative to Heisenberg exchange, it is estimated that

SATURATION TRANSFER SPECTROSCOPY |
357 |
and spin exchange therefore dominates for translational diffusion coefficients of the ion pairs with values
depending on the spin label position. Comparison with linebroadenings (that are equal to the spin-lattice relaxation enhancements, for Heisenberg exchange – see section 8.2), and examination of temperature dependences, confirms that the spin-label relaxation enhancements for
salts are dominated by spin exchange with paramagnetic ions dissolved in the fluid membranes. The relaxation enhancements in Fig. 16 therefore rather directly reflect the penetration profile of the
ions into the membranes. The strong dependence on the anionic counterion arises because partitioning into the membrane takes place as electroneutral ion pairs which minimises the
Born energy penalty for burying a charge in a low-dielectric medium. |
|
|
For ions with longer spin-lattice relaxation times, |
and |
dipolar |
relaxation also contributes in addition to spin exchange (Livshits et al., 2001). The net result is a greater relaxation enhancement, but this is less straightforward to interpret in terms of a simple penetration profile because of the different relaxation mechanisms. For salts, additional relaxation enhancement arises from dipolar interactions with ions within the membrane. This is analysed in terms of the enhancement in
which is bigger than that in
and therefore is diagnostic of a dipolar contribution (see sections 8.2 and 8.3). Again integrating the static dipolar contribution over the
ion distribution in the membrane, it is estimated that
depending on spin label position (Livshits et al., 2001). Thus, the dynamic dipolar mechanism dominates over the static contribution for translational diffusion coefficients of the
ion pairs with values
Most probably both dipolar mechanisms make appreciable contributions to
the relaxation induced by intramembranous |
ions. |
|
|
Only for |
ions do dynamic dipolar interactions make an appreciable |
||
contribution to |
enhancements. From Eqs. 53, 54 and 65, the |
||
enhancement, |
relative to that from |
Heisenberg |
exchange, is: |
|
|
(Livshits |
et al., 2001). |
Therefore spin exchange dominates only for diffusion coefficients of the ion pair with values
In the case of
ions, however, static dipolar enhancements are contributed not only by ions in the membrane, but also by those in the aqueous phase.
These results have considerable implications for the use of paramagnetic ions as relaxants for determining residue accessibilities in site-directed spin-
labelling. |
ions have |
the advantage |
of a purely contact spin-exchange |
mechanism for relaxation |
enhancement. |
ions potentially can give larger |
enhancements, but some selectivity may be lost as a result of the longerrange dipolar contributions. ions may be exploited to obtain distance
358 |
DEREK MARSH ET AL. |
information from the additional contribution from ions in the aqueous phase. This may be an alternative to using gel-phase membranes (as in the previous section), particularly with the less membrane-soluble sulphate salt. The results with other anions illustrate how membrane penetration of nickel salts may be enhanced. In applications of site-directed spin labelling, this is frequently achieved by using electroneutral chelate complexes.
10.OUTLOOK
The two sections, 6 and 9, on applications have illustrated something of the breadth of possibilities available for ST-EPR methodologies, in both their traditional and nonstandard forms. For rotational diffusion measurements, an imminent development will be the implementation of STEPR on high-field spectrometers. The ability to resolve the three canonical and
spectral regions at high-field will give sensitivity to both axial and off-axis rotations (see e.g., Kurad et al., 2001; Marsh et al., 2002). This enhanced orientational selection should help immediately to resolve some of the ambiguities associated with slow anisotropic rotational diffusion that were mentioned in Section 6.4.
The nonstandard forms of ST-EPR, particularly first-harmonic out-of- phase measurements, are still comparatively in their infancy. On the other hand, determination of relaxation enhancements is now a standard part of site-directed spin-labelling methodology, although it relies almost exclusively on progressive saturation experiments. Quite apart from a more unique sensitivity to the rapid-passage experiment has the advantage relative to conventional progressive saturation studies in consisting of a single measurement. It thus can be more readily automated, and is therefore anticipated as a possible method for high-throughput screening of spin-labelled cysteine mutants. Such developments seem to show promise for the application of spin-labelling to proteomics. In short, this aspect of the work of Jim Hyde has a very bright future.
ACKNOWLEDGEMENTS
Our studies on V-ATPases and the 16-kDa Nephrops protein are supported by the European Union (Contract No. QLGI-CT 2000-01801). Work is also supported by joint travel grants between Germany and Hungary (DAAD-MÖB) and between Germany and the Russian Federation (Deutsche Forschungsgemeinschaft-Russian Academy of Sciences). DM and TP are members of the European COST D22 Action.
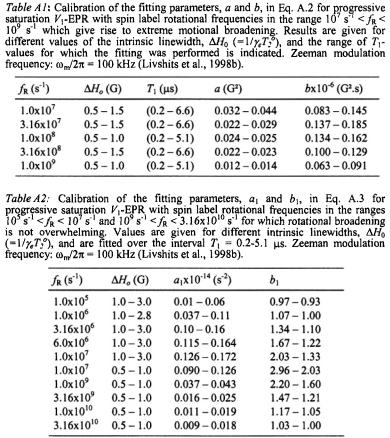
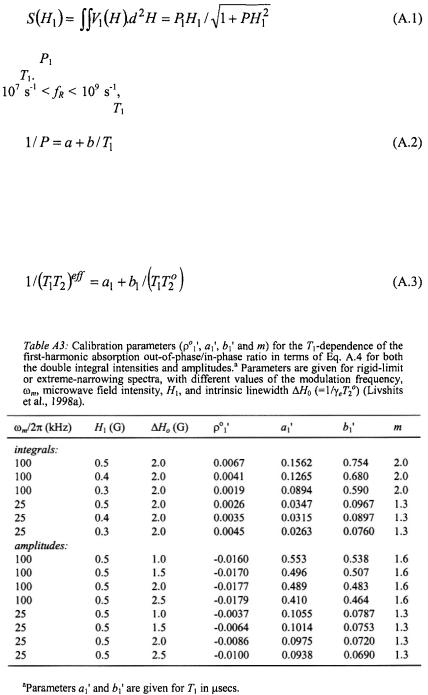
360 |
DEREK MARSH ET AL. |
where |
is a scaling parameter and P is the fitting parameter that depends |
on |
In the extreme motional broadening region, for rotational frequencies |
|
the parameter P is independent of intrinsic linewidth. |
The dependence on is then given by (Livshits et al., 1998b):
where the calibration parameters a and b are given in Table A1. For rotational frequencies in the fast (motional narrowing) and slow regimes, and
is a saturation parameter that depends directly on
In these regimes, the effective
relaxation time product is given by (Livshits et al., 1998b):
where the calibration parameters and
are given in Table A.2.
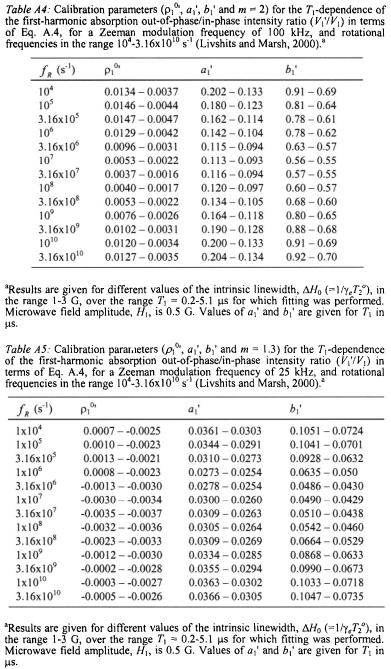
SATURATION TRANSFER SPECTROSCOPY |
361 |