
Micro-Nano Technology for Genomics and Proteomics BioMEMs - Ozkan
.pdf496 |
YAN MEI WANG, SHIRLEY S. CHAN AND ROBERT H. AUSTIN |
[41]K. Wilma Olson, A. Andrey Gorin, Lu, Xiang-Jun, M. Lynette Hock, and B. Victor Zhurkin. DNA Sequencedependent deformability deduced from protein-DNA crystal complexes. Proc. Natl. Acad. Sci., U.S.A., 95:11163, 1998.
[42]K. Wilma Olson and B. Victor Zhurkin. Modeling DNA deformations. Curr. Opin. Struct. Biol., 10:286, 2000.
[43]Y.W. Park and K.J. Breslauer, Drug binding to higher ordered DNA structures: netropsin complexation with a nuclei acid triple helix. Proc. Natl. Acad. Sci. U.S.A., 89:6653, 1992.
[44]G.A. Pedersen, L.J. Jensen, K.E. Nelson, S. Brunak, and D.W. Usssery. A DNA structural atlas for escherichia coli. J. Molec. Biol., 299:706–709, 2000.
[45]G.B. Koudelka, S.C. Harrison, and M. Ptashne. Effect of noncontacted bases on the affinity of 434 operator for 434 repressor and Cro. Nature, 326:886, 1987.
[46]M. Ptashne. Genetic Switch: Phage Lambda and Higher Organisms. Blackwell Science, Malden, MA USA, 1992.
[47]H.L. Puhl, S.R. Gudibande, and M.J. Behe. Poly[d(A·T)] and other syntheitc polydeoxynucleotides containing oligoadenosine tracts form nucleosomes easily. J. Mol. Biol., 222:1149, 1991.
[48]D. Rhodes. Nucleosome cores reconstituted from poly(dA-dT) and the octamer of histones. Nucleic Acids Res., 6:1805, 1979.
[49]A. Scipioni, C. Anselmi, G. Zuccheri, B. Samori, and P.D. Santis. Sequence-dependent DNA curvature and flexibility from scanning force microscopy images. Biophys. J., 83:2408, 2002.
[50]A. Yildiz et al. Myosin V Walks hand-over-hand: Single fluorophore imaging with 1.5-nm localization. Science, 300:2061, 2003.
[51]R.T. Simpson and P. Kunzler. Chromatin and core particles formed from the inner histones and synthetic polydeoxyribonucleotides of defined sequence. Nucleic Acids Res., 6:1387, 1979.
[52]K. Steinmetzer, J. Behlke, S. Brantl, and M. Lorenz. CopR binds and bends its target DNA: a footprinting and fluorescence resonance energy transfer study. Nucleic Acids Res., 30:2052, 2002.
[53]D. Strahs and M. Brenowitz. DNA conformational-changes associated with the cooperative binding of Cirepressor of bacteriophage-lambda to O-R. J. Mol. Biol., 244:494, 1994.
[54]D. Szwajkajzer and K. Breslauer. The influence of sequence on the thermodynamics of DNA melting and ligand-binding properties for a family of octameric duplexes. Biophys. J., 64:A281, 1993.
[55]R.H. Austin, J. Tegenfeldt, H. Cao, S. Chu, and E.C. Cox. Scanning the controls: genomics and nanotechnology. IEEE Transac. Nanotechnol., 1:12, 2002.
[56]O. Tegenfeldt Jonas, Prinz Christelle, Cao Han, Chou Steven, W. Reisner Walter, Riehn Robert, Mei Wang Yan, C. Cox Edward. C. Sturm James, Silberzan Pascal, and H. Austin Robert. The dynamics of genomiclength DNA molecules in 100-nm channels. Proc. Natl. Acad. Sc. U.S.A., 101:10979, 2004.
[57]J.D. Watson and F.H.C. Crick. Molecular structure of nucleic acids: a structure for deoxyribose nucleid acid. Nature, 171:737, 1953.
17
Engineered Ribozymes: Efficient Tools for Molecular Gene Therapy and Gene Discovery
Maki Shiota1, Makoto Miyagishi1,2, and Kazunari Taira1,2
1Department of Chemistry and Biotechnology, School of Engineering, The University of Tokyo, 7-3-1 Hongo, Tokyo 113-8656, Japan
2Gene Function Research Center, National Institute of Advanced Industrial Science and Technology (AIST), Central 4, 1-1-1 Higashi, Tsukuba Science City 305-8562, Japan
17.1. INTRODUCTION
Ribozymes are catalytic RNA molecules that cleave RNAs with high specificity. Hammerhead ribozymes are small and particularly versatile catalytic RNA molecules that cleave RNAs at specific sites (Figure 17.1A, left). The rapidly developing field of RNA catalysis is of particular current interest not only because of the intrinsic catalytic properties of ribozymes but also because of the potential utility of ribozymes as therapeutic agents and specific regulators of gene expression [16, 17, 45, 88, 89, 90, 105]. However, despite extensive efforts, the efficiency of ribozyme in vivo has generally been too low to achieve the desired biological effects. Unlike in vitro, conditions in vivo are very complex and many parameters must be taken into account, in particular conditions, the interactions of a ribozyme or its gene with intracellular proteins, which seem to be significant. Many modifications of and improvements in ribozymes, as well as methods for the introduction of ribozymes into cells, have been developed in attempts to exploit ribozymes in vivo.
Our group developed an efficient ribozyme-expression system that allows the efficient inactivation by a ribozyme of a specific gene in vivo, and we have applied this system to efforts at gene therapy and the functional analysis of genes. In this review, we describe our efforts to generate two novel ribozymes, namely, an allosterically controllable ribozyme and
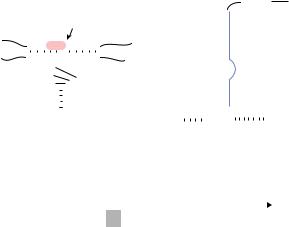
498 |
|
|
|
|
|
|
MAKI SHIOTA, MAKOTO MIYAGISHI AND KAZUNARI TAIRA |
|||||||||||||||||||||
|
|
|
|
|
|
|
|
|
|
|
|
5' |
acc |
|
|
|
|
|
|
3' |
||||||||
|
|
|
|
|
|
|
|
|
|
|
|
|
|
|
|
|
Ribozyme |
|
|
UUUUU |
|
|
||||||
|
|
|
|
|
|
|
|
|
|
|
|
|
g |
|
|
|
|
|
c |
|
|
|
|
Terminator |
||||
|
|
|
|
|
|
|
|
|
|
|
|
|
|
|
|
|
|
|
|
|||||||||
|
|
|
|
|
|
|
|
|
|
|
|
|
u |
|
|
a |
|
|
|
|
||||||||
|
|
|
|
Cleavage site |
|
|
|
|
|
u |
|
|
a |
|
|
|
|
|
|
|
|
|
||||||
|
|
|
|
|
|
|
|
|
|
|
|
|
|
|
|
|
|
|||||||||||
|
|
|
|
|
|
|
|
|
|
|
|
|
g |
|
|
c |
|
|
|
|
|
|
|
|
|
|||
|
|
|
|
|
|
|
|
|
|
|
|
|
|
|
|
|
|
|
|
|
|
|
|
|||||
5' |
|
Stem III NUX site |
|
|
Stem I |
3' |
|
|
G |
|
|
c |
|
|
|
|
|
|
|
|
|
|||||||
|
|
|
|
|
|
|
|
|
|
|
|
|
|
|
||||||||||||||
Substrate |
G C CG U C C C C C G |
|
|
|
|
|
U |
|
|
a |
|
|
|
|
|
|
|
|
|
|||||||||
|
|
C G G C A G G G G G |
5' |
|
|
U |
|
|
a |
|
|
|
|
|
|
|
|
|
||||||||||
|
|
|
|
|
|
|
|
|
|
|
|
|
|
|
||||||||||||||
3' |
|
|
|
U |
|
a |
|
|
|
|
|
|
|
|
|
|||||||||||||
|
|
|
|
|
|
|
|
Linker |
|
|
|
|
|
|
||||||||||||||
|
|
A |
C |
U GA |
|
|
|
|
|
C |
|
a |
|
|
|
|
|
|
||||||||||
|
|
A |
|
|
|
|
|
|
CG |
|
|
ca sequence |
|
|
|
|
|
|
||||||||||
|
|
G |
AGU |
|
|
|
|
|
U |
|
|
a |
|
|
|
|
|
|
|
|
|
|||||||
|
|
|
|
|
|
|
|
|
|
|
|
|
|
|
|
|
|
|||||||||||
|
|
C |
G |
|
|
|
|
|
A |
|
|
u |
|
|
|
|
|
|
|
|
|
|||||||
|
|
C |
C |
|
|
|
|
A box |
G |
|
|
c |
|
|
B box |
|||||||||||||
|
|
|
|
|
|
|
|
|
||||||||||||||||||||
|
|
G |
C Stem II |
|
|
|
|
U |
|
a |
|
|
||||||||||||||||
|
|
G |
C |
|
|
|
|
|
AUG |
|
C |
GGGCCAAA |
||||||||||||||||
|
|
A |
G |
|
|
|
|
U GGUG |
|
|
||||||||||||||||||
|
|
|
|
A |
A |
|
|
|
|
|
|
U UCAC |
|
|
CCCGGUU G |
|||||||||||||
|
|
|
|
|
|
|
|
|
|
|
|
A |
G U |
|
A |
|
C |
C |
||||||||||
|
|
Hammerhead ribozyme |
|
|
U |
|
AAGU |
|
|
|
|
|
|
|||||||||||||||
|
|
|
|
|
|
|
|
|
|
|
|
|
C |
|
|
G |
|
G |
|
|
|
|
|
|
||||
|
|
|
|
|
|
|
|
|
|
|
|
|
|
|
|
|
|
|
|
|
|
|
|
|||||
|
|
|
|
|
|
|
|
|
|
|
|
|
G |
|
C |
|
|
|
|
|
|
|
|
|
||||
|
|
|
|
|
|
|
|
|
|
|
|
|
|
|
|
|
|
|
|
|
|
|
||||||
|
|
|
|
|
|
|
|
|
|
|
|
|
C |
|
G |
|
|
|
|
|
|
|
|
|
||||
|
|
|
|
|
|
|
|
|
|
|
|
|
|
|
|
|
|
|
|
|
|
|
||||||
|
|
|
|
|
|
|
|
|
|
|
|
|
C |
|
C |
|
|
|
|
|
|
|
|
|
||||
|
|
|
|
|
|
|
|
|
|
|
|
|
U |
|
A |
|
|
|
|
|
|
|
|
|||||
|
|
|
|
|
|
|
|
|
|
|
|
|
AAC |
|
|
|
|
|
|
|
|
|
||||||
|
|
|
|
|
|
|
|
|
tRNA Val- driven transcript |
|
|
|
|
|
|
|
|
|
|
|
|
|
|
|
|
|||
|
|
|
|
|
|
|
|
|
|
|
|
|
|
|
|
|
|
|
|
|
|
|
|
|
||||
|
|
|
|
|
|
|
|
|
|
|
|
|
|
|
|
|
|
|
|
|
|
|
|
|
|
|
|
|
|
|
Upstream |
|
|
tRNA |
Val promotor |
|
Linker |
Ribozyme |
|
Terminator |
|
|
|
|
|
|
|||||||||||
|
|
sequence |
|
|
|
sequence |
|
|
|
|
|
|
|
|||||||||||||||
|
|
|
|
|
A box |
B box |
|
|
|
|
|
|
|
|
|
|
|
|
|
|
|
|
|
|
FIGURE 17.1. Secondary structure of the hammerhead ribozyme (on the left). The hammerhead ribozyme consists of a substrate-binding region (stems I and III) and a catalytic core with a stem-loop II region. Using a construct in which the substrate-binding region of the ribozyme is complementary to the target RNA, we can create molecular
scissors that cleave RNA in a site-specific manner. When the catalytic core captures the catalytically indispensable Mg2+ ions, cleavage occurs only at a NUX triplet (Ncan be any base; X can be A, C or U). tRNAVal-driven
transcript (on the right). RNA polymerase III recognizes a promoter that is located within the gene for the tRNA sequence being transcribed. The ribozyme is linked downstream of the partially modified human tRNAVal through a linker, as shown below the secondary structures.
an RNA-protein hybrid ribozyme. We chose the name “maxizyme” for the allosterically controllable ribozyme, which has sensor arms that recognize sequences in its target mRNA. In the presence of such target sequences exclusively, it forms a cavity that can capture the catalytically indispensable Mg2+ ions that allow it to cleave the target mRNA [49–51, 101, 102]. In the RNA-protein hybrid ribozyme, the cleavage activity of a hammerhead ribozyme is coupled with the unwinding activity of an RNA helicase. This hybrid ribozyme can cleave its target mRNA extremely efficiently, regardless of the secondary structure of the target RNA [107, 113].
17.2. METHODS FOR THE INTRODUCTION OF RIBOZYMES INTO CELLS
If synthetic ribozymes are to work inside cells in the human body, they must be delivered to individual cells and access their targets without degradation. However, ribozymes are rapidly destroyed in the gastrointestinal tract and in the blood. In addition, ribozymes are nucleic acids with high molecular weights, and it is unlikely that ribozymes themselves can be taken up directly by cells.
For the administration of ribozymes in a therapeutic setting in vivo, ribozymes must be carried by vectors, which can efficiently infiltrate the phospholipid bilayer of cell membranes. Many virus-based vectors have been shown to have potential as vehicles for gene delivery in vivo, and these viruses include adenovirus, herpesvirus, retrovirus,
ENGINEERED RIBOZYMES: EFFICIENT TOOLS FOR MOLECULAR GENE THERAPY |
499 |
adeno-associated virus, and lentivirus [24, 43, 57, 70, 74, 87, 116]. Rapid advances in viral vector technology have led not only to improved efficiency of introduction of ribozymes into cells but also to more precise control of transduction, such as tissue-specific transduction. The various viral vectors are derived from detoxified viruses, but their exploitation has been fraught with problems related to production, immunogenicity and safety [27, 40, 117]. In particular, it is difficult to prepare and purify many viral vectors.
Artificial non-viral vectors tha are currently being developed involve naked nucleic acids, either alone or in a variety of molecular conjugates with, for example, liposomes, polymers, and polypeptides [1, 10, 72, 86]. Compared to the use of viral vectors, the use of non-viral vectors is cost-effective and their synthesis is straightforward and safe. However, the efficiency of introduction of these vectors is sometimes relative low. It is unclear whether viral vectors or non-viral vectors are the best carriers of ribozymes into cells and different goals might be better met with one system or the other.
Available methods for the administration of ribozymes in vivo include direct administration of chemically synthesized ribozymes and the introduction of ribozymes via plasmids that carry a gene for the ribozymes, which is transcribed inside cells. In the former method, it is necessary to stabilize ribozymes against nucleolytic degradation by chemical modification, such as thio modification. However, such modifications can increase both toxicity and cost, and large amounts of ribozyme are needed to ensure that sufficient numbers of ribozymes reach the target cells. Thus, methods using plasmids have received the most attention because a stable form of DNA can be administered and the ribozyme can be synthesized constitutively inside cells.
Once a ribozyme has been introduced ito cells, the environment within the cells must be taken into account to ensure that the ribozyme will function effectively.
17.3. RIBOZYME EXPRESSION SYSTEMS
17.3.1. The pol III System
The efficacy of a ribozyme after it has been introduced via a plasmid into cells, depends on five factors: (1) the amount of ribozyme that is transcribed; (2) the stability of the ribozyme after transcription; (3) the subcellular localization of the ribozyme; (4) the activity of the ribozyme itself; and (5) the accessibility to the ribozyme of the target mRNA. Among these factors, the first three are critically controlled by the expression system.
When the gene for a ribozyme is introduced into a cell, it can be transcribed by the transcriptional machinery to produce active ribozymes. Initially, the RNA polymerase II system (pol II) was usually used for the expression of ribozymes. In this system, transcripts are automatically modified, and a cap and a poly(A) tail are added at the 5’ end and the 3’ end, respectively. Thus, the stability of transcripts is guaranteed and, moreover, the transcripts are exported from the nucleus to the cytoplasm as mature mRNAs. However, while this pol II expression system is suitable for the transcription of long RNAs (several hundred to several thousand bases), it is not particularly suitable for the transcription of short RNAs, such as ribozymes. Moreover, for accurate transcription by the pol II system, extra sequences must be added to an otherwise compact ribozyme. These extra sequences might decrease the ribozyme’s activity by forcing it to assume a higher-order structure or to bind to cellular
500 |
MAKI SHIOTA, MAKOTO MIYAGISHI AND KAZUNARI TAIRA |
proteins. Thus, we have focused our attention on the RNA polymerase III (pol III) system, which is involved mainly in the transcription of short RNAs, such as tRNA [20]. The rate of transcription by pol III is two to three orders of magnitude higher than that by pol II [12]. In addition, shorter extra sequences are needed. Therefore, the pol III system is ideal for the expression of ribozymes. Among available pol III systems, we chose to focus on the tRNA transcription system. In the pol III system, the promoter is located within the tRNA sequence that is transcribed and, thus, it is inevitable that a portion of the tRNA becomes incorporated into the ribozyme. Since tRNAs are exported to the cytoplasm from the nucleus as mature tRNAs with trimmed 5’ and 3’ ends, it was postulated that tRNA-attached ribozymes would not be exported to the cytoplasm. However, as noted below, tRNA-attached ribozymes are exported efficiently to the cytoplasm in mammalian cells. Moreover, the additional tRNA sequence does not cause any loss of ribozyme activity but, rather, appears to have a positive effect on ribozyme activity [34, 35, 42].
17.3.2. Relationship Between the Higher-Order Structure of Ribozymes and their Activity
In our ribozyme-expression system, the ribozyme is linked downstream of a partially modified human tRNAVal via a linker (Figure 17.2). The higher order structure of ribozymes obviously affects their stability and ribozymes with fewer exposed regions in a single-strand structure are more stable than others against intracellular nucleolytic degradation. In our system, we include a small stem-loop structure at the 3’ end to promote stability. By contrast, if a double-stranded region is too long, it might be recognized by other nucleases. To prevent such problems, the double-stranded region corresponding to the linker is designed with a bulge.
In our ribozyme-expression system, the higher-order structure of the ribozyme is strongly affected by the length of the linker. To determine how the higher-order structure might affect the activity and stability of our ribozyme [42], we constructed three different types of expression system, in which ribozymes targeted to the same sequence (a sequence that is relatively strongly conserved in HIV-1) were linked to the promoter of the gene for the tRNA via linkers with different sequences (Figure 17.2A). When we predicted secondary structures by Zucker’s method [120], we found that the ribozymes had secondary structures that were similar to those of tRNAs but that they had different structures in the substrate-binding region.
In ribozymes (Rz) 1 through 3 in Figure 17.2A, the degree of freedom of the substratebinding region increases in that order (a single strand has a higher degree of freedom than a double strand and, thus, binds more efficiently to the substrate). For ribozymes, which are RNA enzymes, ease of binding to the substrate is an important determinant of activity, and we can predict that a higher degree of freedom of the substrate-binding region should result in higher activity. In experiments designed to test this prediction, the differents in activity in vitro against a short substrate were confirmed. We also examined the stability of the ribozymes in cells. Plasmids encoding each ribozyme were introduced into cells, and levels of ribozymes in cells were monitored by Northern hybridization. The most stable ribozyme, Rz2, was 26-fold more abundant than the most unstable ribozyme, Rz1, and also 5-fold more abundant than Rz3 (Figure 17.3A). It is unclear why these structures, which are so similar overall, have such very different stabilities. The differences might be due to differences in the extend to which each ribozyme in the cell is recognized by a degradative nuclease.
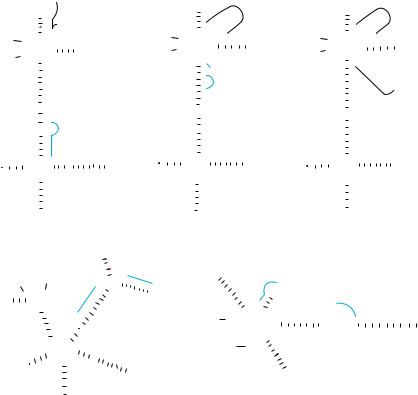


504 |
MAKI SHIOTA, MAKOTO MIYAGISHI AND KAZUNARI TAIRA |
17.3.4. Mechanism of the Export of tRNA-Ribozymes from the Nucleus to the Cytoplasm
In our hands, all tRNAVal-ribozymes transcribed by our pol III expression system were located in the cytoplasm. However, there are reports that ribozymes transcribed by a similar system that also exploits a tRNA promoter accumulate in the nucleus [6, 23]. A ribozyme that was designed to target the same HIV sequence as ours ([6]; Figure 17.2B, right) was reported to be localized in the nucleus. In addition, the extend of inhibition of expression of the target mRNA by the ribozyme was substantially lower than we observed. We have also been able to design tRNA-type ribozymes (for a different application) that accumulate in the nucleus without being transported into the cytoplasm; the secondary structures of such a ribozyme is shown in Figure 17.2B (left).
When we compare the secondary structures of the ribozymes that are transported to the cytoplasm with those of ribozymes that accumulate in the nucleus, we can easily see that the structures are different (Figures 17.2A and 2B). In particular, the ribozyme that is transported to the cytoplasm (Figure 17.2A) assumes a cloverleaf structure that is similar but not identical to that of a tRNA, while the ribozyme that accumulate in the nucleus (Figure 17.2B) has a very different structure. These results suggest that a tRNAVal-ribozyme can be transported to the cytoplasm only when the structure of the tRNAVal-ribozyme resembles that of a tRNA.
Rapid progress has been made in efforts to understand the mechanism involved in the export of tRNAs to the cytoplasm [3, 4, 25, 46, 63, 65]. The transport of tRNAs requires a tRNA-binding protein called exportin-t (Xpo-t) and the Ran GTPase, and rapid transport requires the hydrolysis of GTP. It appears that only mature tRNAs, with accurately trimmed 5’ and 3’ ends and an attached 3’ CCA end are recognized by Xpo-t [3, 4, 46, 63, 65]. Aminoacylation of each tRNA also appears to be critical for the export of tRNAs from the nucleus to the cytoplasm in Xenopus oocytes [65] and in yeast [25]. In Xenopus oocytes, immature tRNAs with several extra nucleotides at the 3’ end are not recognized by Xpo-t and, as a result, they are not exported to the cytoplasm. This phenomenon suggests the existence of a proofreading mechanism in cells whereby only tRNAs that are usable in the cytoplasm, with mature 5’ and 3’ ends, can be exported to the cytoplasm. However, in our studies of tRNAVal-ribozymes, which might be considered equivalent to a kind of immature tRNA because of the extra sequences at their 3’ ends, we found that such ribozymes were efficiently exported to the cytoplasm in mammalian cells [49–54, 42, 33, 35, 111].
We investigated the discrepancy between the reported observations that led to the proposal of the existence of a proofreading mechanism and our own observations of the efficient export to the cytoplasm of tRNAVal-ribozymes [55]. We first considered the possible existence of an alternative pathway for the export of tRNAs. The existence of an additional pathway for tRNA export had already been suggested in yeast and we can assume that tRNA export is essential for cell survival. However, contrary to our expectations, we found evidence to suggest that Xpo-t was probably involved in the transport of tRNAVal-ribozymes in somatic cells and, moreover, that a mechanism similar to that for the recognition of the tertiary structure of tRNAs was involved in the interaction of Xpo-t with tRNAVal- ribozymes [54]. Nevertheless, in Xenopus oocytes, tRNA-attached ribozymes were not exported to the cytoplasm, as we might have predicted from the proofreading hypothesis. Further investigations revealed that the Xpo-t/RanGTP complex did not interact with tRNAattached ribozymes in oocytes even though we detected such an interaction in vitro and,
ENGINEERED RIBOZYMES: EFFICIENT TOOLS FOR MOLECULAR GENE THERAPY |
505 |
more importantly, in several lines of somatic cells. These findings hinted at the presence of some kind of inhibitor in Xenopus oocytes rather than suggesting the involvement of an alternative pathway in somatic cells. We found subsequently that a nuclear extract prepared from Xenopus oocytes did, indeed, strongly inhibit the export of tRNA-attached ribozymes in somatic cells, and this observation suggests the existence of a strong inhibitor(s) in Xenopus oocytes specifically.
It seems likely that the export of tRNAs in Xenopus oocytes is subject to a special kind of regulation. Moreover, the proofreading mechanism that is operative in Xenopus oocytes seems to involve a specific inhibitor(s) that appears specifically to recognize immature tRNAs and, thus, structures such as tRNA-attached ribozyme. In somatic cells, when the choice of linker and ribozyme sequence is made appropriately, a tRNA-attached ribozyme seems to be recognized as a mature tRNA by Xpo-t and to be exported by Xpo-t to the cytoplasm.
17.4. RNA-PROTEIN HYBRID RIBOZYMES
17.4.1. Accessibility to Ribozymes of their Target mRNAs
If ribozymes are to function effectively inside cells, high-level expression, intracellular stability, and efficient export to the cytoplasm are essential. However, even ribozymes that have been improved in each of these respects are sometimes ineffective, probably because they are unable to reach their target. It is likely that the rate-limiting step in vivo for the cleavage by a ribozyme of a phosphodiester bond is the association and annealing of the ribozyme with its target site [33].
To overcome the problem of accessibility, computer-generated predictions of secondary structure are typically used to identify targets that are most likely to have an open conformation [120]. However, these predictions are often inaccurate because of unpredictable RNAprotein interactions that change the structures of RNAs in cells. To circumvent this problem in a similar system, some researchers have applied an unwieldy systematic approach that involves huge numbers of candidate molecules [68, 84]. Such an approach tends to be both expensive and laborious. To avoid dependence on either of these approaches, we attempted to develop a ribozyme that would be able to access any chosen target site regardless of local secondary structure.
17.4.2. Hybrid Ribozymes that Efficiently Cleave their Target mRNAs, Regardless of Secondary Structure
We postulated that it might be useful to design a ribozyme that could recruit a protein that could, in turn, eliminate any interfering secondary structure in the target mRNA, thereby making any site in the target mRNA accessible to the ribozyme. To create such a ribozyme, we tried to link a ribozyme to an RNA helicase, a member of a class of proteins with nonspecific RNA-binding, sliding, and unwinding activities [13, 30, 59, 108]. We introduced an RNA motif, the constitutive transport element (CTE). The CTE appears to interact with RNA helicases both in vitro and in vivo [8, 26, 28, 32, 60, 92, 103, 104, 115] and was discovered as a cytoplasmic transport signal for D-type retroviral RNA. We postulated that