
- •Preface
- •Acknowledgments
- •Reviewers
- •Contents
- •CHAPTER OUTLINE
- •CYTOPLASM
- •Plasmalemma
- •Mitochondria
- •Ribosomes
- •Endoplasmic Reticulum
- •Golgi Apparatus, cis-Golgi Network, and the trans-Golgi Network
- •Endosomes
- •Lysosomes
- •Peroxisomes
- •Proteasomes
- •Cytoskeleton
- •Inclusions
- •NUCLEUS
- •CELL CYCLE
- •CHAPTER OUTLINE
- •EPITHELIUM
- •Epithelial Membranes
- •GLANDS
- •Chapter Summary
- •CHAPTER OUTLINE
- •EXTRACELLULAR MATRIX
- •Fibers
- •Amorphous Ground Substance
- •Extracellular Fluid
- •CELLS
- •CONNECTIVE TISSUE TYPES
- •Chapter Summary
- •CHAPTER OUTLINE
- •CARTILAGE
- •BONE
- •Cells of Bone
- •Osteogenesis
- •Bone Remodeling
- •Chapter Summary
- •CHAPTER OUTLINE
- •FORMED ELEMENTS OF BLOOD
- •Lymphocytes
- •Neutrophils
- •PLASMA
- •COAGULATION
- •HEMOPOIESIS
- •Erythrocytic Series
- •Granulocytic Series
- •Chapter Summary
- •CHAPTER OUTLINE
- •SKELETAL MUSCLE
- •Sliding Filament Model of Muscle Contraction
- •CARDIAC MUSCLE
- •SMOOTH MUSCLE
- •Chapter Summary
- •CHAPTER OUTLINE
- •BLOOD-BRAIN BARRIER
- •NEURONS
- •Membrane Resting Potential
- •Action Potential
- •Myoneural Junctions
- •Neurotransmitter Substances
- •SUPPORTING CELLS
- •PERIPHERAL NERVES
- •Chapter Summary
- •CHAPTER OUTLINE
- •BLOOD VASCULAR SYSTEM
- •HEART
- •ARTERIES
- •Capillary Permeability
- •Endothelial Cell Functions
- •VEINS
- •LYMPH VASCULAR SYSTEM
- •Chapter Summary
- •CHAPTER OUTLINE
- •CELLS OF THE IMMUNE SYSTEM
- •Antigen-Presenting Cells
- •DIFFUSE LYMPHOID TISSUE
- •LYMPH NODES
- •TONSILS
- •SPLEEN
- •THYMUS
- •Chapter Summary
- •CHAPTER OUTLINE
- •PITUITARY GLAND
- •Pars Intermedia
- •Pars Nervosa and Infundibular Stalk
- •Pars Tuberalis
- •THYROID GLAND
- •Parathyroid Glands
- •Suprarenal Glands
- •Cortex
- •Medulla
- •Pineal Body
- •Chapter Summary
- •CHAPTER OUTLINE
- •SKIN
- •Epidermis of Thick Skin
- •Dermis
- •DERIVATIVES OF SKIN
- •Chapter Summary
- •CHAPTER OUTLINE
- •CONDUCTING PORTION OF THE RESPIRATORY SYSTEM
- •Extrapulmonary Region
- •Intrapulmonary Region
- •RESPIRATORY PORTION OF THE RESPIRATORY SYSTEM
- •MECHANISM OF RESPIRATION
- •Chapter Summary
- •CHAPTER OUTLINE
- •ORAL CAVITY AND ORAL MUCOSA
- •Oral Mucosa
- •Tongue
- •Teeth
- •Odontogenesis (See Graphic 13-2)
- •Chapter Summary
- •CHAPTER OUTLINE
- •REGIONS OF THE DIGESTIVE TRACT
- •Esophagus
- •Stomach
- •Small Intestine
- •Large Intestine
- •GUT-ASSOCIATED LYMPHOID TISSUE
- •DIGESTION AND ABSORPTION
- •Carbohydrates
- •Proteins
- •Lipids
- •Water and Ions
- •Chapter Summary
- •CHAPTER OUTLINE
- •MAJOR SALIVARY GLANDS
- •PANCREAS
- •LIVER
- •Exocrine Function of the Liver
- •Endocrine and Other Functions of the Liver
- •GALLBLADDER
- •Chapter Summary
- •CHAPTER OUTLINE
- •KIDNEY
- •Uriniferous Tubule
- •Nephron
- •Collecting Tubules
- •FORMATION OF URINE FROM ULTRAFILTRATE
- •EXTRARENAL EXCRETORY PASSAGES
- •Chapter Summary
- •CHAPTER OUTLINE
- •OVARY
- •Ovarian Follicles
- •Regulation of Follicle Maturation and Ovulation
- •Corpus Luteum and Corpus Albicans
- •GENITAL DUCTS
- •Oviduct
- •Uterus
- •FERTILIZATION, IMPLANTATION, AND THE PLACENTA
- •Fertilization and Implantation
- •Placenta
- •VAGINA
- •EXTERNAL GENITALIA
- •MAMMARY GLANDS
- •Chapter Summary
- •CHAPTER OUTLINE
- •TESTES
- •Spermatogenesis
- •GENITAL DUCTS
- •ACCESSORY GLANDS
- •PENIS
- •Erection and Ejaculation
- •Chapter Summary
- •CHAPTER OUTLINE
- •SENSORY ENDINGS
- •Chapter Summary
- •Terminology of Staining
- •Common Stains Used in Histology
- •Hematoxylin and Eosin
- •Wright Stain
- •Weigert Method for Elastic Fibers and Elastic van Gieson Stain
- •Silver Stain
- •Iron Hematoxylin
- •Bielschowsky Silver Stain
- •Masson Trichrome
- •Periodic Acid-Schiff Reaction (PAS)
- •Alcian Blue
- •von Kossa Stain
- •Sudan Red
- •Mucicarmine Stain
- •Safranin-O
- •Toluidine Blue

386 U R I N A R Y S Y S T E M
Descending Thin Limb
¥As the ultraÞltrate passes down the descending thin limb of Henle’s loop, it reacts to the increasing gradient of osmotic concentration in the interstitium.
¥Water leaves, and a limited amount of salts enters the lumen, reducing the volume and increasing the salt concentration of the ultraÞltrate (which becomes hypertonic).
Ascending Thin Limb
¥In the ascending thin limb of Henle’s loop, water is conserved, but salts are permitted to leave the ultraÞltrate, decreasing its osmolarity and contributing to the maintenance of the osmotic concentration gradient.
Concentration of Urine in the Collecting Tubule
The ultraÞltrate that enters the collecting tubule is hypoosmotic. As it passes down the collecting tubule, it is subject to the increasing osmotic gradient of the renal interstitium.
¥If antidiuretic hormone (ADH) is released from the pars nervosa of the pituitary, the cells of the collecting tubules become permeable to water, which leaves the lumen of the collecting tubule, increasing the concentration of the urine.
¥In the absence of ADH, the cells of the collecting tubule are impermeable to water, and the urine remains hypotonic.
¥The collecting tubule is also responsible for permitting urea to diffuse into the interstitium of the inner medulla. The high interstitial osmolarity of this region is attributed to the urea concentration.
Role of the Vasa Recta in Urine Concentration (Countercurrent Exchange System)
The vasa recta assists in the maintenance of the osmotic concentration gradient of the renal medulla, since these capillary loops are completely permeable to salts and water.
¥Thus, as the blood descends in the arteria recta, it becomes hyperosmotic, but as it ascends in the vena recta, its osmolarity returns to normal.
¥It is also important to realize that the arteria recta carries a smaller volume than the vena recta, permitting
the removal of the ßuid and salts transported into the renal interstitium by the uriniferous tubules.
EXTRARENAL EXCRETORY PASSAGES
The extrarenal excretory passages consist of the ureters, urinary bladder, and urethra.
¥The ureters and bladder are lined by transitional epithelia.
¥The ureters possess a Þbroelastic lamina propria and two to three layers of smooth muscle arranged in an inner longitudinal and an outer circular fashion. The third muscle layer, the outermost longitudinal layer, appears in the lower one-third of the ureter.
¥The transitional epithelial lining of the bladder and of the other urinary passages offers an impermeable barrier to urine.
¥The plasma membrane of the surface-most cells of a transitional epithelium is thicker than the average plasma membrane and is composed of a lattice structure consisting of hexagonally arrayed elements.
¥Furthermore, since cells of the transitional epithelium must line an ever larger surface as the urinary bladder distends, the plasma membrane is folded in a mosaiclike fashion.
Folding occurs at the interplaque regions, whereas the thickened plaque regions present vesicular profiles, which probably become unfolded as urine accumulates in the bladder.
¥The subepithelial connective tissue of the bladder is composed, according to most, of a lamina propria and a submucosa.
¥The three smooth muscle layers of the muscularis are extensively interlaced, making them indistinguishable in some areas.
¥The urethra of the male differs from that of the female not only in its length but also in its function and epithelial lining.
The lamina propria of both sexes contains mucous glands of Littré and intraepithelial glands, which lubricate the lining of the urethra, facilitating the passage of urine to the outside.
The urethra is described in Chapter 17, ÒFemale Reproductive System,Ó and Chapter 18, ÒMale Reproductive System.Ó

U R I N A R Y S Y S T E M 387
CLINICAL CONSIDERATIONS
Odor and Color of Urine
The odor and color of urine may provide clues to the individual’s disease state. Normal urine is either colorless or has a yellow color if the urine is concentrated. Similarly, dilute urine has very little odor, whereas concentrated urine has a pungent smell. If the color of urine is reddish, the individual may have porphyria or there is fresh blood in the urine; if the color is brown, the possibility is that breakdown byproducts of damaged muscle or breakdown by-products of hemoglobin are in the urine. Black discoloration could be due to the presence of melanin pigment in the urine, whereas cloudy urine could be an indication of the presence of acidic crystals or the presence of pus derived from urinary tract infection. Additionally, certain medications can discolor the urine, and the patient should be warned in advance about the color change. Changes in the odor of urine can be due to diabetes that is not being controlled (a sweet odor); fetid odor could indicate the presence of a urinary tract infection; and a musty odor of urine in a young patient may suggest phenylketonuria.
Tubular Necrosis
Tubular necrosis may result in acute renal failure. Cells of the renal tubules die either by being poisoned due to exposure to toxic chemicals, such as mercury or carbon tetrachloride, or die because of severe cardiovascular shock that reduces blood flow to the kidneys. The dead cells become sloughed off and occlude the lumina of their tubules. If the basal laminae remain intact, epithelial cell division may be able to repair the damage in less than 3 weeks.
Acute Glomerulonephritis
Acute glomerulonephritis is usually the result of a localized beta streptococcal infection in a region of the body other than the kidney (e.g., strep throat). Plasma cells secrete antibodies that complex with streptococcal antigens, forming an insoluble antigen-antibody complex that is filtered by the basal lamina between the podocytes and the endothelial cells of the glomerulus. As the immune complex builds up in the glomerular basal lamina, the epithelial cells and mesangial cells proliferate. Additionally, leukocytes accumulate in the glomerulus, congesting and blocking it. Moreover, pharmacologic agents released at the site of damage cause the glomerulus to become leaky, and proteins, platelets, and erythrocytes may enter the glomerular filtrate. Usually after the acute inflammation abates, the glomeruli repair themselves and the normal kidney function returns. Occasionally, however, the damage is extensive and kidney function becomes permanently impaired.
Diabetes Insipidus
Diabetes insipidus occurs because of damage to the cells of the hypothalamus that manufacture ADH (antidiuretic hormone). The low levels of ADH interfere with the ability of the collecting tubules of the kidney to concentrate urine. The excess fluid loss in the formation of copious quantities of dilute urine results in polydipsia (excessive thirst) and dehydration.
Diabetic Glomerulosclerosis
Diabetes mellitus causes vascular pathologies that involve blood vessels throughout the body, including those of the glomerular capillary network where synthesis of the basement membrane components increases to such an extent that it interferes with normal filtration. Additionally, hypercellularity of the mesangial cell population also interferes with the function of the normal
This figure is from the kidney of a patient with end-stage renal disease as a result of diabetes mellitus. Note that the glomerular capillaries are engorged with blood, the intraglomerular cell population is increased, and the glomerular basement membrane displays evidence of being thickened. (Reprinted with permission from Rubin R, Strayer D, et al., eds. Rubin’s Pathology. Clinicopathologic Foundations of Medicine, 5th ed., Baltimore: Lippincott Williams & Wilkins, 2008, p. 709.)
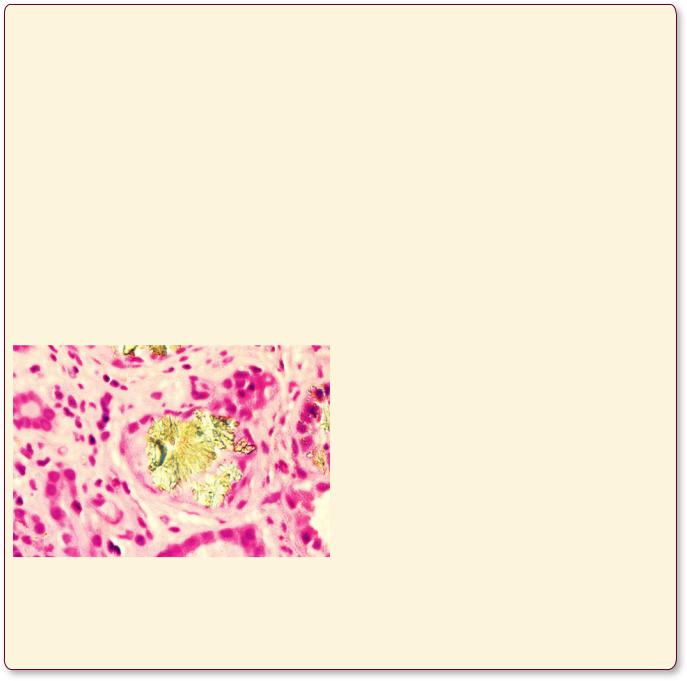
388 U R I N A R Y S Y S T E M
filtration barrier and sclerosis ensues. Electron micrography demonstrates that the lamina densa of the glomerular basal membrane may increase as much as 10-fold, which becomes engorged with various plasma proteins. In the United States, approximately 35% of patients in endstage renal disease suffer from diabetic glomerulosclerosis caused by both type I and type II diabetes mellitus.
Urate Nephropathy
Urate nephropathy is the deposition of uric acid crystals in the kidney tubules or in the renal interstitium as a result of elevated levels of uric acid in the blood. In most cases this condition is due to the patient suffering from primary gout; however, high uric acid blood levels also occur in cases of chemotherapy in cancer treatment as well as in patients who have reduced excretions of uric acid, such as in cases of lead poisoning. Although in most patients urate nephropathy is not life threatening, it may result in acute renal failure with fatal consequences.
This figure is from the kidney of a patient demonstrating the deposition of uric acid crystals in the collecting tubule, indicating that the individual is suffering from urate nephropathy. (Reprinted with permission from Rubin R, Strayer D, et al., eds. Rubin’s Pathology. Clinicopathologic Foundations of Medicine, 5th ed., Baltimore: Lippincott Williams & Wilkins, 2008, p. 736.)
Kidney Stones
Kidney stones usually form due to the condition known as hyperparathyroidism, in which the formation of excess parathyroid hormone (PTH) by the parathyroid glands results in an increased level of osteoclastic activity. The resorption of bone, as well as the increased absorption of calcium and phosphates from the gastrointestinal tract, eventuates higher than normal blood calcium levels. As the kidneys excrete higher than normal concentrations of calcium and phosphates, their presence in the urine, especially under alkaline conditions, causes their precipitation in the kidney tubules. Continued accretion of these ions onto the crystal surface causes an increase in the size of the crystals, and they become known as kidney stones.
Cancers of the Kidney
Cancers of the kidney are usually solid tumors, whereas cysts of the kidney are usually benign. The most common symptom of kidney cancer is blood in the urine, although the amount of blood may be undetectable without a microscopic examination of the urine. Usually, kidney cancers are accompanied by pain and fever, but frequently, they are discovered by abdominal palpation during routine physicals when the physician detects a lump in the region of the kidney. Since kidney cancers spread early and usually to the lung, the prognosis is poor.
Bladder Cancer
Annually, there are more than 50,000 new cases of transitional cell carcinomas of the bladder in the United States. Interestingly, almost 65% of the affected individuals are male, and about half of these patients smoke cigarettes. The most prominent symptom of bladder cancer is blood in the urine, followed by burning sensation and pain on urination, as well as an increased frequency of the urge to urinate. Although these symptoms are frequently
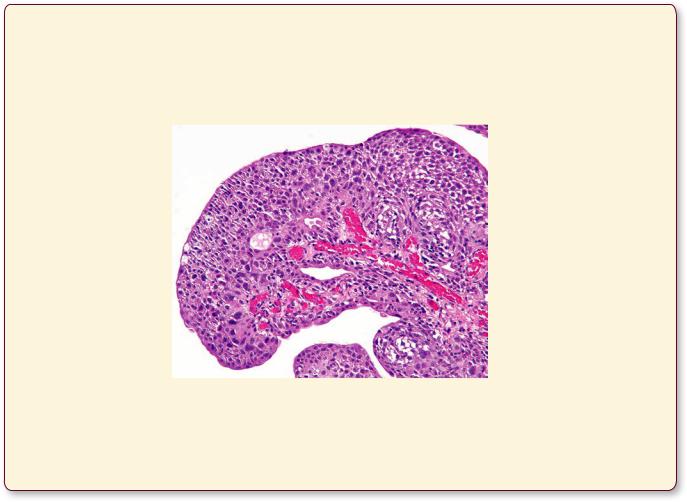
U R I N A R Y S Y S T E M 389
confused with cystitis, the condition becomes suspicious once the antibiotics fail to alleviate the problem, and cytology of the urine demonstrates the presence of cancerous transitional cells. If caught early, before the carcinoma invades the deeper tissues, the survival rate is as
great as 95%; however, if the tumor is a rapidly dividing one that invades the muscular layers of the bladder and reaches the lymph nodes, the 5-year survival rate drops to less than 45%.
This figure is from a urinary bladder with high-grade papillary urothelial carcinoma. Note that the transitional epithelium is disorganized and the individual epithelial cells display dense, pleomorphic nuclei. (Reprinted with permission from Rubin R, Strayer D, et al., eds. Rubin’s Pathology. Clinicopathologic Foundations of Medicine, 5th ed., Baltimore: Lippincott Williams & Wilkins, 2008, p. 757.)
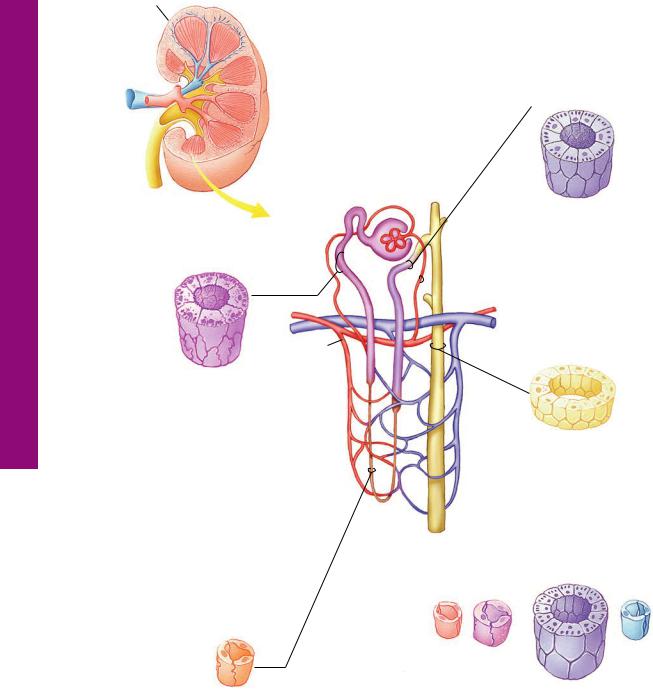
Tubules Uriniferous • 1-16 GRAPHIC
390 |
U R I N A R Y S Y S T E M |
|
|
Kidney |
The renal artery enters the renal vein and ureter leave at the |
|
|
|
|
|
hilus. The medulla, composed of 10–18 renal pyramids is |
|
|
surrounded by the cortex, housing the renal corpuscles, |
|
|
the distal and the proximal convoluted tubules, and |
|
|
medullary rays. |
Distal convoluted tuble
|
|
|
Medulla |
|
|
|
|
|
|
|
|
|
|
|
|
|
Cortex |
Afferent |
||
|
|
|
||||
|
|
|
|
|||
|
|
|
|
glomerular |
||
|
|
|
|
arteriole |
|
(Cuboidal epithelial cells |
|
|
|
|
|
||
|
|
|
|
|
|
|
|
|
|
|
|
|
with short microvilli) |
Proximal convoluted tubule |
|
|
The distal tubule, |
|||
|
|
composed of low |
||||
|
|
|
|
|
|
cuboidal cells with |
|
|
|
|
|
|
short, sparse microvilli, |
|
|
|
|
|
|
begins deeper in the |
|
|
|
|
|
|
medulla than the |
|
|
|
|
|
|
proximal tubule ends. |
|
|
|
Efferent |
|
|
Collecting tubule |
|
|
|
glomerular |
|
|
|
|
|
|
arteriole |
|
|
|
(Cuboidal epithelial cells with |
|
|
|
|||
long dense microvilli) |
|
|
|
The proximal tubule is composed of cuboidal cells whose basal plasma membrane displays deep infoldings (striations) housing mitochondria, indicative of active transport. Its apical plasma membrane displays numerous long microvilli, denoting absorption and secretion.
(Cuboidal epithelial cells)
The collecting ducts, possessing cuboidal cells, begin in the medullary rays of the cortex and end at the area cribrosa.
Thick segment of loop of Henle
Artery |
Vein |
Thin segment of loop of Henle
(Squamous epithelial cells) |
(Cuboidal epithelial cells) |
The thin limbs Henle’s loop, composed of |
The arteria recta of the vasa recta originates as |
squamous cells, are long in juxtamedullary |
a branch of the efferent glomerular arteriole of |
and extremely short in cortical nephrons. |
juxtamedullary nephrons. Its counterpart from |
|
cortical nephrons establishes the peritubular |
|
capillary network of the cortex. |
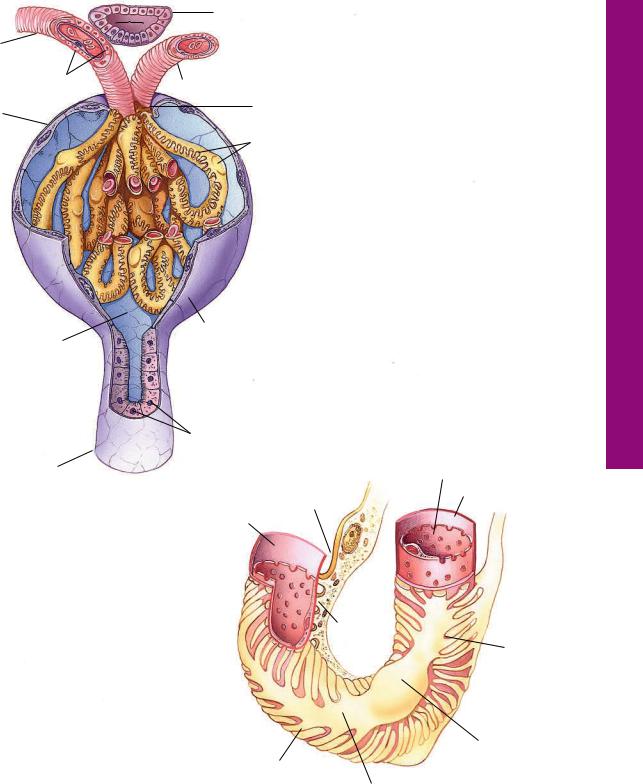
U R I N A R Y S Y S T E M 391
Afferent arteriole
Juxtaglomerular cells
Bowman’s capsule (parietal layer)
Urinary space
Distal tubule
Macula densa
Juxtaglomerular cells (modified smooth muscle)
Efferent arteriole
Vascular pole
Bowman’s capsule (visceral layer podocytes)
Parietal layer
Urinary pole
The parietal layer of Bowman’s capsule is composed of simple squamous epithelium, whereas its visceral layer is modified to form podocytes. The ultrafiltrate enters Bowman’s (urinary) space and leaves the renal corpuscle at its urinary pole, via the proximal convoluted tubule. The afferent glomerular arteriole enters and the efferent glomerular arteriole leaves the renal corpuscle at its vascular pole, the former supplying and the latter draining the glomerulus. The macula densa component of the distal tubule comes in close proximity to the juxtaglomerular cells of the afferent (and efferent) glomerular arterioles.
Brush border (microvilli)
Proximal convoluted tubule
Primary process
Basal lamina
Endothelium
The fenestrated capillaries constituting the glomerulus are invested by pedicels arising from the primary processes of podocytes. Filtration slits between adjoining pedicles are bridged by thin diaphragms that, in association with the fused basal laminae of the capillary endothelium and podocyte, contribute to the formation of the filtration barrier.
Secondary process
(pedicel)
Endothelium
Podocyte
Basal lamina
Secondary process
(pedicel) Filtration slit
Podocyte cell body
Primary process
Corpuscle Renal• 2-16 GRAPHIC
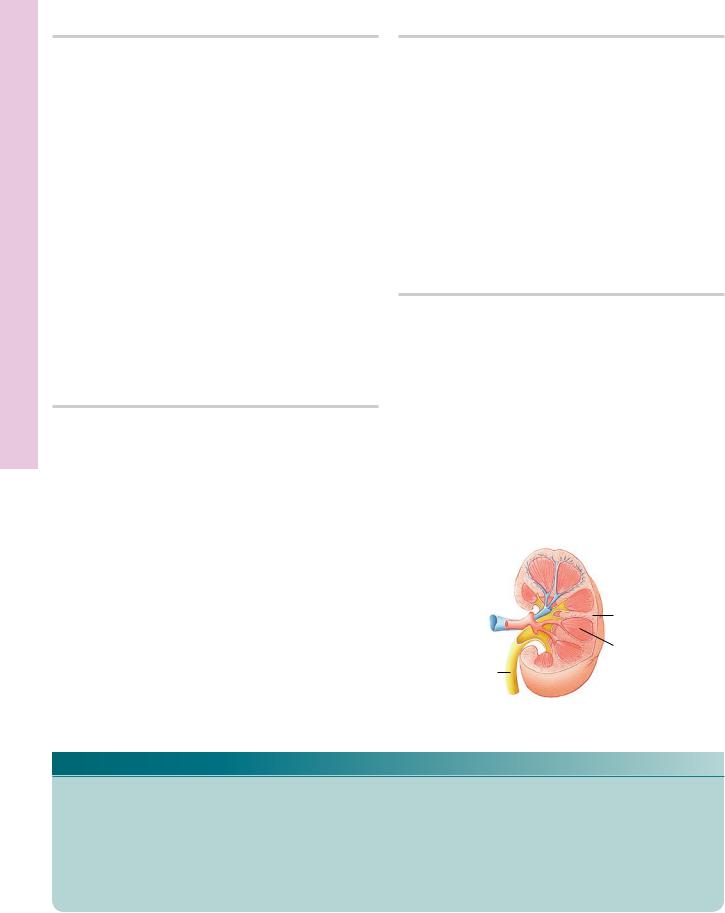
Morphology General and Survey idney, • K1-16 PLATE
392 U R I N A R Y S Y S T E M
FIGURE 1. Kidney cortex and medulla. Human. Paraffin section. ×14.
The kidney cortex and part of the medulla are presented at a low magnification to provide an insight into the cortical architecture. The capsule (Ca) appears as a thin, light line at the top of the photomicrograph. The darker area below it, occupying the top half of the photomicrograph, is the cortex (C); the lower lighter region is the medulla (M). Note that longitudinal rays of the medulla appear to invade the cortex; these are known as medullary rays (MR). The tissue between medullary rays appears convoluted and is referred to as the cortical labyrinth (CL). It is occupied by dense, round structures, the renal corpuscles (RC). These are the first part of the nephrons, and their location in the cortex is indicative of their time of development as well as of their function. They are referred to as superficial (1), midcortical (2), or juxtamedullary nephrons (3). Each medullary ray and one-half of the cortical labyrinth on either side of it constitutes a lobule of the kidney. The lobule extends into the medulla, but its borders are undefinable histologically (approximated by vertical lines). The large vessels at the corticomedullary junction are arcuate vessels (AV); those in the cortical labyrinth are interlobular vessels (IV).
FIGURE 3. Kidney cortex. Human. Paraffin section. ×132.
The various components of the cortical labyrinth and portions of two medullary rays are evident. The orientation of this photomicrograph is perpendicular to that of Figure 1. Note that two renal corpuscles (RC) in the center of the photomicrograph display a slight shrinkage artifact and thus clearly demonstrate Bowman’s space (BS). The renal corpuscles are surrounded by cross sections of proximal convoluted tubules (PT), distal convoluted tubules
(DT), and macula densa (MD). Since the proximal convoluted tubule is much longer than the convoluted portion of the distal tubule, the number of proximal convoluted tubule profiles around a renal corpuscle outnumbers the distal convoluted tubule profiles by approximately 7 to 1. The medullary rays contain the pars recta (PR) of the proximal tubule, the ascending thick limbs of Henle’s loop (AT), and collecting tubules (CT).
FIGURE 2. Kidney capsule. Monkey. Plastic section. ×540.
The kidney is invested by a capsule (Ca) composed of dense collagenous connective tissue. The two layers of the capsule are clearly evident, in that the outer layer is paler and houses occasional fibroblasts (Fb); the inner layer is thinner and darker in color, and instead of fibroblasts, it has myofibroblasts whose nuclei are plumper than those of fibroblasts. Although this structure is not highly vascular, it does possess some capsular vessels (CV). Observe the numerous red blood cells in the lumina of these vessels. The deeper aspect of the capsule possesses a rich capillary network (CN) that is supplied by the terminal branches of the interlobular arteries and is drained by the stellate veins, tributaries of the interlobular veins. Note the cross sections of the proximal convoluted tubules (PT).
FIGURE 4. Colored colloidin-injected kidney. Paraffin section. ×132.
This specimen was prepared by injecting the renal artery with colored colloidin, and a thick section was taken to demonstrate the vascular supply of the renal corpuscle. Each renal corpuscle contains tufts of capillaries, the glomerulus (G), which is supplied by the afferent glomerular arteriole (AA) and drained by the efferent glomerular arteriole (EA). Note that the outer diameter of the afferent glomerular arteriole is greater than that of the efferent glomerular arteriole; however, the diameters of the two lumina are about equal. It is important to realize that the glomerulus is an arterial capillary network; therefore, the pressure within these vessels is greater than that of normal capillary beds. This results in more effective filtration pressure. The large vessel on the lower right is an interlobular artery (IA), and it is the parent vessel of the afferent glomerular arterioles.
Capsule
Cortex
Medulla
Collecting
tubules
Kidney
KEY
AA |
afferent arteriole |
CN |
capillary network |
IV |
interlobular vessel |
AT |
ascending thick limb of |
CT |
collecting tubule |
M |
medulla |
|
Henle’s loop |
CV |
capsular vessel |
MD |
macula densa |
AV |
arcuate vessel |
DT |
distal convoluted tubule |
MR |
medullary ray |
BS |
Bowman’s space |
EA |
efferent arteriole |
PR |
pars recta |
C |
cortex |
Fb |
fibroblast |
PT |
proximal convoluted tubule |
Ca |
capsule |
G |
glomerulus |
RC |
renal corpuscle |
CL |
cortical labyrinth |
IA |
interlobular artery |
|
|
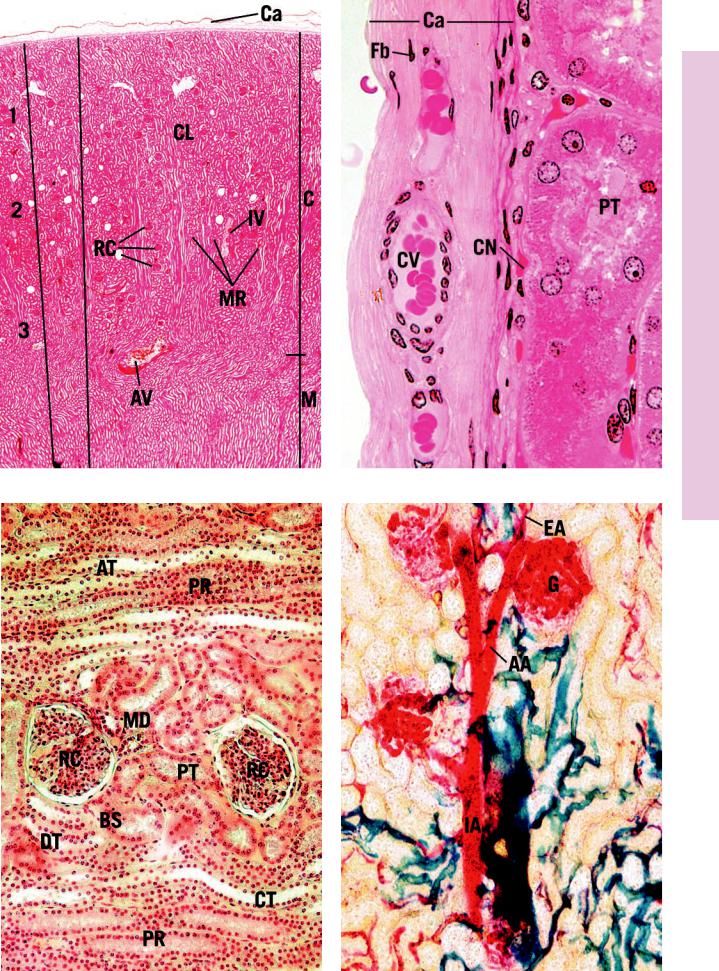
Morphology General and Survey idney, • K1-16 PLATE
FIGURE 1 |
FIGURE 2 |
FIGURE 3 |
FIGURE 4 |
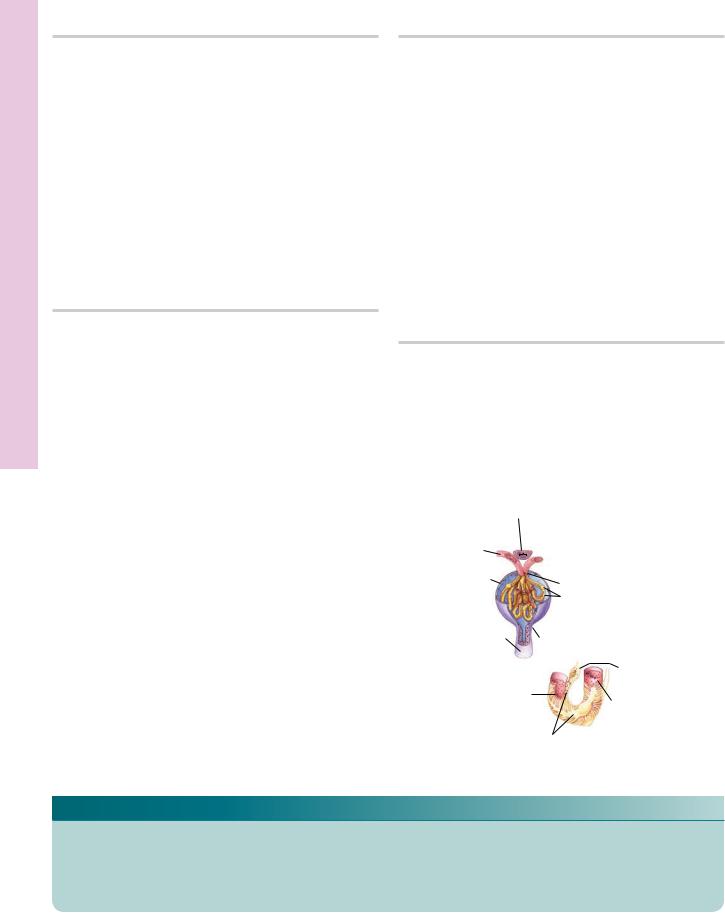
ortex C Renal• 2-16 PLATE
394 U R I N A R Y S Y S T E M
FIGURE 1. Kidney cortical labyrinth. Monkey. Plastic section. ×270.
The center of this photomicrograph is occupied by a renal corpuscle. The urinary pole is evident as the short neck empties into the convoluted portion of the proximal tubule (PT). The renal corpuscle is composed of the glomerulus (G), tufts of capillaries, the visceral layer of Bowman’s capsule (podocytes) that is intimately associated with the glomerulus, Bowman’s space (BS) into which the ultrafiltrate is expressed from the capillaries, and the parietal layer (PL) of Bowman’s capsule, consisting of a simple squamous epithelium. Additionally, mesangial cells are also present in the renal corpuscle. Most of the tubular profiles surrounding the renal corpuscle are transverse sections of the darker-staining proximal tubules (PT), which outnumber the cross sections of the lighterstaining distal tubules (DT).
FIGURE 3. Kidney cortical labyrinth. Monkey. Plastic section. ×270.
The vascular pole of this renal corpuscle is very clearly represented. It is in this region that the afferent glomerular arteriole (AA) enters the renal corpuscle and the efferent glomerular arteriole (EA) leaves, draining the glomerulus. Observe that these two vessels and their capillaries are supported by mesangial cells (Mg). Note that although the outer diameter of the afferent glomerular arteriole is greater than that of the efferent glomerular arteriole, their luminal diameters are approximately the same. The renal corpuscle is surrounded by cross-sectional profiles of distal (DT) and proximal (PT) tubules. The boxed area is presented at a higher magnification in Figure 4. Inset. Glomerulus. Kidney. Monkey. Plastic section. ×720. The glomerulus is composed of capillaries whose endothelial cell (En) nuclei bulge into the lumen. The endothelial cells are separated from podocytes (P), modified visceral cell layer of Bowman’s capsule, by a thick basal lamina (arrows). Mesangial cells (Mg) form both supporting and phagocytic elements of the renal corpuscle. Note that major processes (asterisks) of the podocytes are also distinguishable in this photomicrograph.
FIGURE 2. Kidney cortical labyrinth. Monkey. Plastic section. ×270.
The renal corpuscle in the center of the photomicrograph displays all of the characteristics identified in Figure 1, except that instead of the urinary pole, the vascular pole (VP) is presented. That is the region where the afferent and efferent glomerular arterioles enter and leave the renal corpuscle, respectively. Some of the smooth muscle cells of the afferent (and sometimes efferent) glomerular arterioles are modified in that they contain renin granules. These modified cells are known as juxtaglomerular cells (JC). They are closely associated with the macula densa (MD) region of the distal tubule. Again, note that most of the cross-sectional profiles of tubules surrounding the renal corpuscle belong to the convoluted portion of the proximal tubules (PT), whereas only one or two are distal tubules. Observe the rich vascularity (BV) of the renal cortex as well as the scant amount of connective tissue elements (arrows) associated with these vessels.
FIGURE 4. Juxtaglomerular apparatus. Kidney. Monkey. Plastic section. ×1,325.
The boxed area of Figure 3 is magnified to present the juxtaglomerular apparatus. This is composed of the macula densa (MD) region of the distal tubule and apparent juxtaglomerular cells (JC), modified smooth muscle cells of the afferent glomerular arteriole (AA). Observe the granules (arrowheads) in the juxtaglomerular cells, which are believed to be the enzyme renin. Note the nuclei (asterisks) of the endothelial cells lining the afferent glomerular arteriole.
Distal tubule
Afferent |
|
|
||
arteriole |
|
Efferent arteriole |
||
Bowman’s |
|
|||
|
Vascular pole |
|||
capsule |
|
|||
|
Bowman’s capsule |
|||
(parietal layer) |
|
|||
|
(visceral layer podocytes) |
|||
|
|
|
|
|
Urinary space |
|
|
|
|
|
|
|
||
Proximal |
Urinary pole |
|||
convoluted tubule |
|
|
||
|
|
|
|
Podocyte |
Endothelium
Endothelium
Secondary process (pedicel)
Primary process
Renal corpuscle
KEY
AA |
afferent arteriole |
En |
endothelial cell |
P |
podocyte |
BS |
Bowman’s space |
G |
glomerulus |
PL |
parietal layer |
BV |
blood vessel |
JC |
juxtaglomerular cell |
PT |
proximal tubule |
DT |
distal tubule |
MD |
macula densa |
VP |
vascular pole |
EA |
efferent arteriole |
Mg |
mesangial cell |
|
|
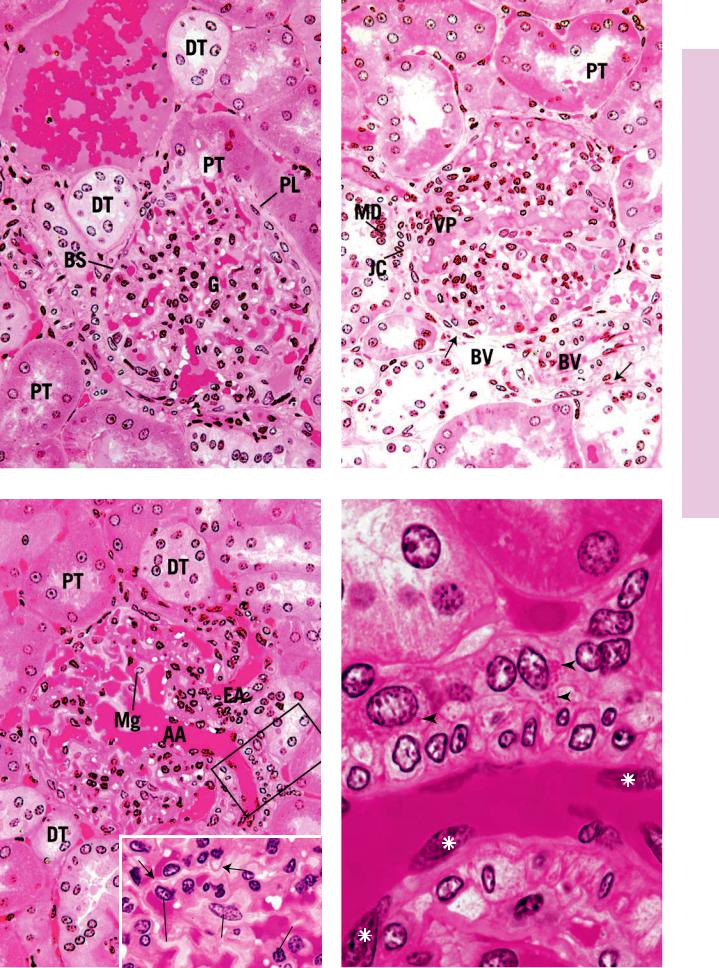
ortex C Renal• 2-16 PLATE
FIGURE 1 |
FIGURE 2 |
MD
JC
AA
Mg
En P
FIGURE 3 |
FIGURE 4 |
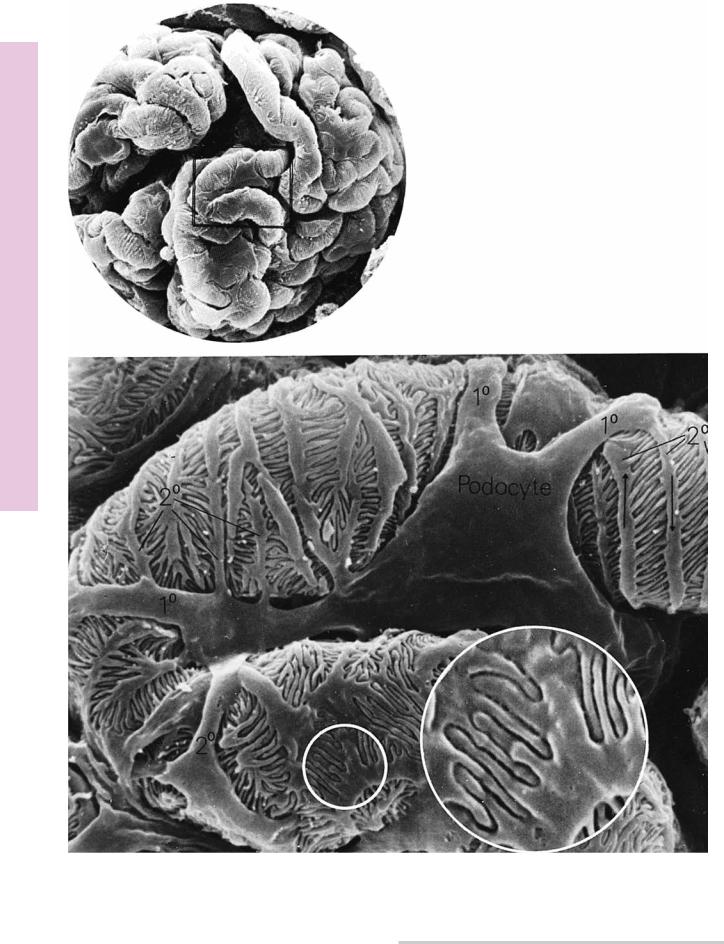
Microscopy Electron Scanning , Glomerulus• 3-16 PLATE
FIGURE 1
FIGURE 1. Scanning electron micrograph of a glomerulus, displaying the primary and secondary processes and pedicels of podocytes. Top, ×700; bottom, ×4,000; and inset, ×6,000. (From Ross MH,
Reith EJ, Romrell LJ. Histology: A Text and Atlas. 2nd ed., Baltimore: Williams & Wilkins,
1989, p. 536.)
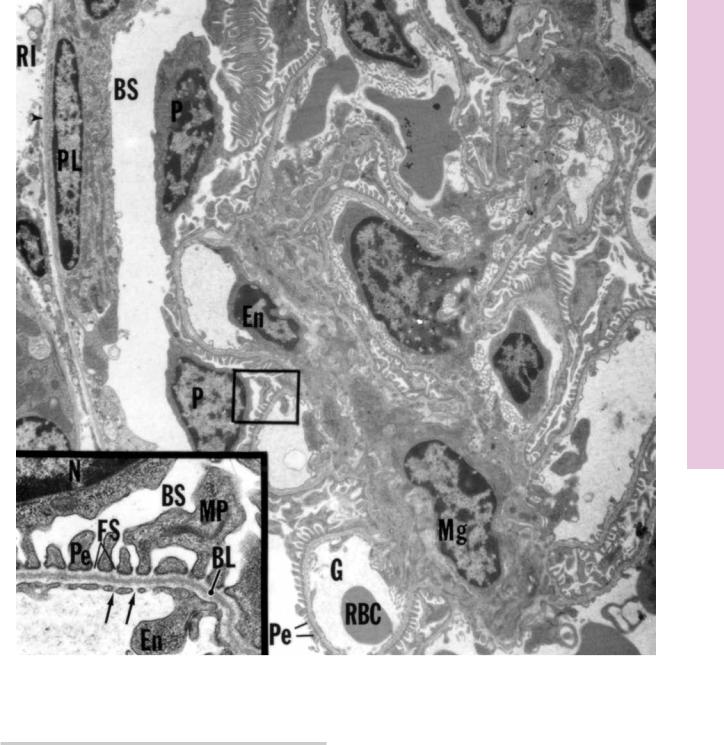
U R I N A R Y S Y S T E M 397
Microscopy Electron orpuscle, C Renal• 4-16 PLATE
FIGURE 1
FIGURE 1. Kidney cortex. Renal corpuscle. Mouse. Electron microscopy. ×3,780.
Various components of the renal corpuscle are displayed in this electron micrograph. Note the basal lamina (arrowhead) separating the simple squamous cells of the parietal layer (PL) of Bowman’s capsule from the renal interstitium (RI). Bowman’s space (BS) and the podocytes (P) are shown to advantage, as are the glomeruli (G) and surrounding pedicels (Pe). Mesangial cells (Mg) occupy the space between capillary loops, and several
red blood cells (RBC) and endothelial cells (En) are also evident. Inset. Podocyte and glomerulus. Mouse. Electron microscopy.
×6,300. This is a higher magnification of the boxed area, presenting a portion of a podocyte. Observe its nucleus (N), major process (MP), and pedicels (Pe). Note that the pedicels lie on a basal lamina (BL) that is composed of a lamina rara externa, lamina densa, and lamina rara interna. Observe the fenestrations (arrows) in the endothelial lining (En) of the glomerulus. The spaces between the pedicels, known as filtration slits (FS), lead into Bowman’s space (BS).
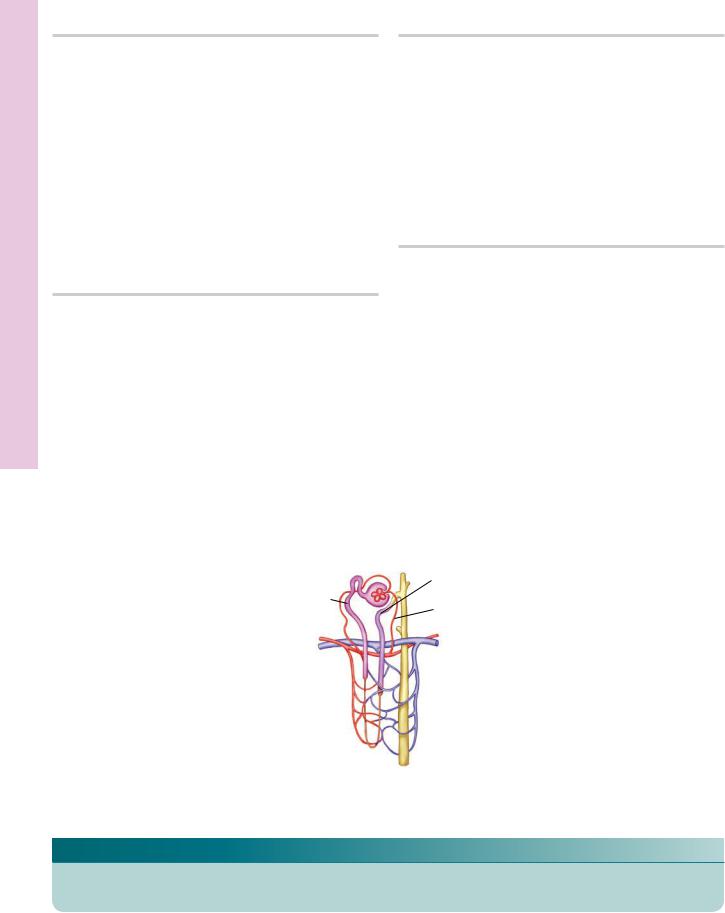
Medulla Renal• 5-16 PLATE
398 U R I N A R Y S Y S T E M
FIGURE 1. Renal medulla. Monkey. Plastic section. ×270.
This photomicrograph of the renal medulla demonstrates the arrangement of the various tubular and vascular structures. The formed connective tissue elements among the tubules and vessels are very sparse and constitute mainly fibroblasts, macrophages, and fibers (asterisks). The major tubular elements in evidence are the collecting tubules (CT), recognizable by the conspicuous lateral plasma membranes of their tall cuboidal (or low columnar) cells, thick limbs of Henle’s loop (TH), and occasional thin limbs of Henle’s loop (TL). Many vascular elements are noted; these are the vasa recta spuria, whose thicker-walled descending limbs are the arteriolae rectae spuriae (AR) and thinner-walled ascending limbs are the venulae rectae spuriae (VR).
FIGURE 3. Renal papilla. x.s. Monkey. Plastic section. ×540.
In the deeper aspect of the medulla, collecting tubules merge with each other, forming larger and larger structures. The largest of these ducts are known as papillary ducts (PD), or ducts of Bellini, which may be recognized by their tall, pale columnar cells and their easily discernible lateral plasma membranes (arrows). These ducts open at the apex of the renal papilla, in the region known as the area cribrosa. The thin limbs of Henle’s loop (TL) are evident. These structures form the hairpin-like loops of Henle in this region, where the ascending thin limbs recur to ascend in the medulla, eventually to become thicker, forming the straight portion of the distal tubule. Note that the arteriolae rectae spuriae (AR) and the venulae rectae spuriae (VR) follow the thin limbs of Henle’s loop deep into the renal papilla. Some of the connective tissue elements are marked by asterisks.
FIGURE 2. Renal papilla. x.s. Human. Paraffin section. ×270.
The most conspicuous tubular elements of the renal papilla are the collecting tubules (CT), with their cuboidal cells, whose lateral plasma membranes are evident. The numerous thin-walled structures are the thin limbs of Henle’s loop (TL) as well as the arteriolae rectae spuriae (AR) and venulae rectae spuriae (VR) that may be identified by the presence of blood in their lumina. The formed connective tissue elements (asterisks) may be discerned in the interstitium among the various tubules of the kidney. An occasional thick limb of Henle’s loop (TH) may also be observed.
FIGURE 4. Renal medulla. l.s. Monkey. Plastic section. ×270.
This photomicrograph is similar to Figure 1, except that it is a longitudinal rather than a transverse section of the renal medulla. The center is occupied by a collecting tubule (CT), as is distinguished by the tall cuboidal cells whose lateral plasma membranes are evident. The collecting tubule is flanked by thick limbs of Henle’s loop (TH). The vasa recta are filled with blood, and the thickness of their walls identifies whether they are arteriolae rectae spuriae
(AR) or venulae rectae spuriae (VR). A thin limb of Henle’s loop
(TL) is also identifiable.
|
|
|
|
|
Distal convoluted |
Proximal |
|
|
|
|
tubule |
|
|
|
|
Afferent glomerular |
|
convoluted |
|
|
|
|
|
tubule |
|
|
|
|
arteriole |
|
|
|
|
|
Thick limb of |
|
|
|
|
|
|
|
|
|
|
|
loop of Henle |
|
|
|
|
|
Collecting |
|
|
|
|
|
|
Thin segment |
|
|
|
|
tubule |
|
|
|
|
|
|
of loop of Henle |
|
|
|
|
|
Uriniferous tubule
KEY
AR |
arteriolae rectae spuriae |
PD |
papillary duct |
TL |
thin limb of Henle’s loop |
CT |
collecting tubule |
TH |
thick limb of Henle’s loop |
VR |
venulae rectae spuriae |
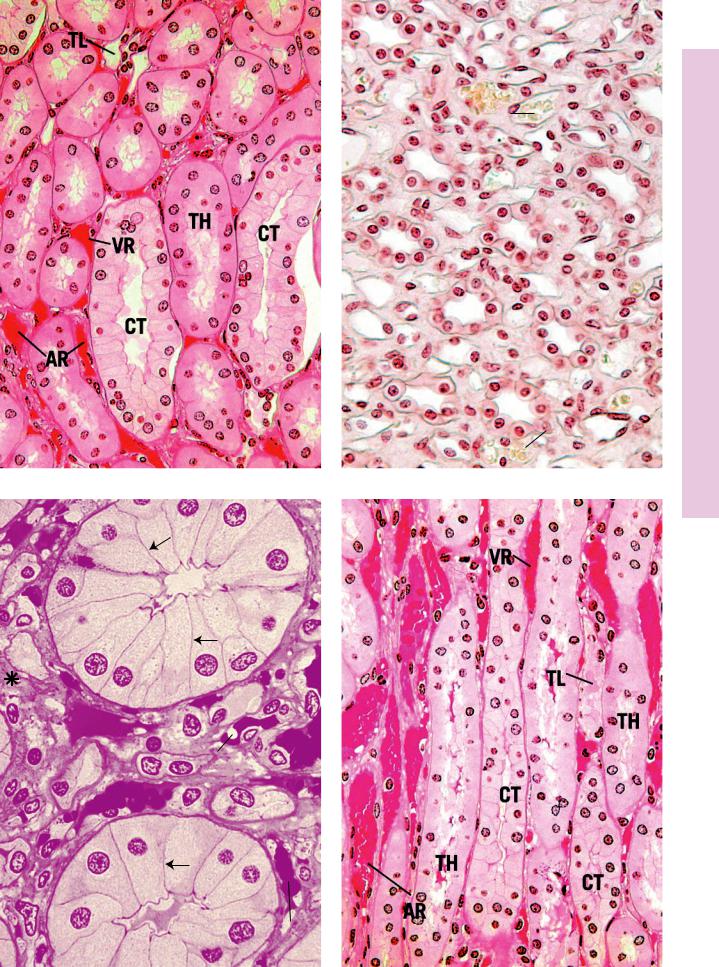
CT
VR
CT
TL
AR
FIGURE 1 |
FIGURE 2 |
PD
TL AR
TL
PD
VR
FIGURE 3 |
FIGURE 4 |
Medulla Renal• 5-16 PLATE
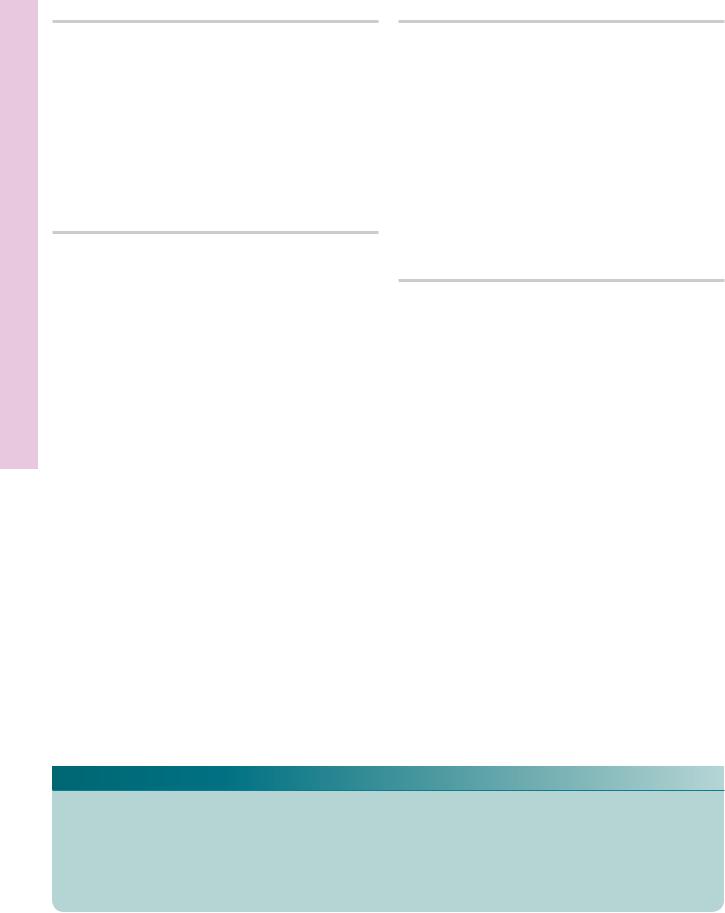
Bladder Urinary and eter • Ur6-16 PLATE
400 U R I N A R Y S Y S T E M
FIGURE 1. Ureter. x.s. Human. Paraffin section. ×14.
This low-power photomicrograph of the ureter displays its stel- late-shaped lumen (L) and thick lining epithelium (E). The interface between the subepithelial connective tissue (SCT) and the smooth muscle coat (SM) is indicated by arrows. The muscle coat is surrounded by a fibrous adventitia (Ad), which houses the numerous vascular channels and nerve fibers that travel with the ureter. Thus, the wall of the ureter consists of the mucosa (epithelium and underlying connective tissue), muscularis, and adventitia.
FIGURE 3. Urinary bladder. Monkey. Plastic section. ×14.
The urinary bladder stores urine until it is ready to be voided. Since the volume of the bladder changes with the amount of urine it contains, its mucosa may or may not display folds. This particular specimen is not distended, hence the numerous folds (arrows). Moreover, the transitional epithelium (TE) of this preparation is also thick, whereas in the distended phase, the epithelium would be much thinner. Note also that the thick muscularis is composed of three layers of smooth muscle: inner longitudinal (IL), middle circular (MC), and outer longitudinal (OL). The muscle layers are surrounded either by an adventitia composed of loose connective tissue—as is the case in this photomicrograph—or by a serosa, depending on the region of the bladder being examined.
FIGURE 2. Ureter. x.s. Monkey. Plastic section. ×132.
The mucosa is highly convoluted and consists of a thick, transitional epithelium whose free surface possesses characteristic dome-shaped cells (D). The basal cell layer sits on a basal lamina (arrows), which separates the epithelium from the underlying fibrous connective tissue. The muscularis consists of three layers of smooth muscle: inner longitudinal (IL), middle circular (MC), and outer longitudinal (OL). These three layers are not always present, for the outer longitudinal layer is found only in the inferior one-third of the ureter, that is, the portion nearest the urinary bladder. The adventitia (Ad) is composed of fibrous connective tissue that anchors the ureter to the posterior body wall and adjacent structures.
FIGURE 4. Urinary bladder. Monkey. Plastic section. ×132.
The bladder is lined by transitional epithelium (TE), whose typical surface dome-shaped cells are shown to advantage. Some of these cells are binucleated. The epithelium is separated from the underlying connective tissue by a basal lamina (arrows). This subepithelial connective tissue is frequently said to be divided into a lamina propria (LP) and a submucosa (Sm). The vascularity of this region is demonstrated by the numerous venules
(V) and arterioles (A). These vessels possess smaller tributaries and branches that supply the regions closer to the epithelium.
Inset. Transitional epithelium. Monkey. Plastic section. ×540. The boxed region of the transitional epithelium is presented at a higher magnification to demonstrate the large, dome-shaped cells (arrow) at the free surface. These cells are characteristic of the empty bladder. When that structure is distended with urine, the dome-shaped cells assume a flattened morphology, and the entire epithelium becomes thinner (being reduced from five to seven to only three cell layers thick). Note that occasional cells may be binucleated.
KEY
A |
arteriole |
LP |
lamina propria |
SM |
smooth muscle coat |
Ad |
adventitia |
MC |
middle circular muscularis |
Sm |
submucosa |
D |
dome-shaped cell |
OL |
outer longitudinal |
TE |
transitional epithelium |
E |
epithelium |
|
muscularis |
V |
venule |
IL |
inner longitudinal |
SCT |
subepithelial connective |
|
|
|
muscularis |
|
tissue |
|
|
Llumen

Bladder Urinary and eter • Ur6-16 PLATE
FIGURE 1 |
FIGURE 2 |
FIGURE 3 |
FIGURE 4 |