
книги студ / color atlas of physiology 5th ed[1]. (a. despopoulos et al, thieme 2003)
.pdf
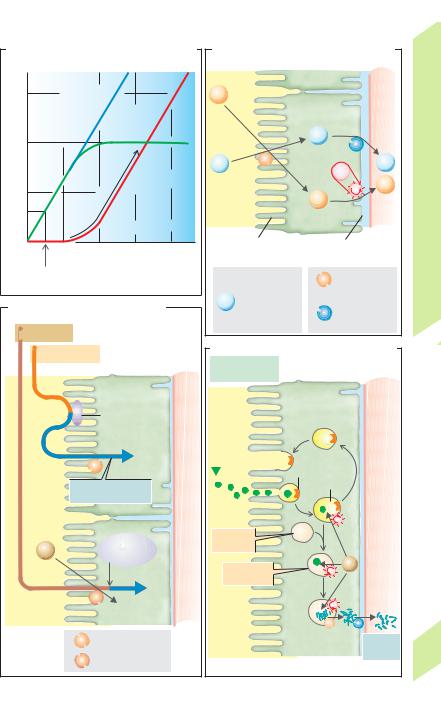





|
Reabsorption of Water, Formation of |
pertonic towards the papillae (see below) and |
|||
|
if the vasa recta are permeable to water. Part of |
||||
|
Concentrated Urine |
|
|||
|
|
the water diffuses by osmosis from the de- |
|||
|
|
|
|||
|
The glomeruli filter around 180 L of plasma |
scending vasa recta to the ascending ones, |
|||
|
water each day (= GFR; !p. 152). By compari- |
thereby “bypassing” the inner medulla (!A4). |
|||
|
. |
|
Due to the extraction of water, the concentra- |
||
|
son, the normal urine output (VU) is relatively |
||||
|
small (0.5 to 2 L/day). Normal fluctuations are |
tion of all other blood components increases as |
|||
|
. |
|
the blood approaches the papilla. The plasma |
||
|
called antidiuresis (low VU) and diuresis (high |
||||
|
. |
|
osmolality in the vasa recta is therefore con- |
||
Balance |
VU; !p. 172). Urine output above the range of |
||||
normal is called polyuria. Below normal output |
tinuously adjusted to the osmolality of the sur- |
||||
|
|||||
|
is defined as oliguria (!0.5 L/day) or anuria |
rounding interstitium, which rises towards the |
|||
Water |
(!0.1 L/day). The osmolality (!p. 377) of |
papilla. The hematocrit in the vasa recta also |
|||
plasma and glomerular filtrate is |
about |
rises. Conversely, substances entering the |
|||
|
|||||
|
290 mOsm/kg H2O (= Posm); that of the final |
blood in the renal medulla diffuse from the as- |
|||
and |
urine (Uosm) ranges from 50 (hypotonic urine in |
cending to the descending vasa recta, provided |
|||
extreme water diuresis) to about 1200 mOsm/ |
the walls of both vessels are permeable to |
||||
Salt, |
kg H2O (hypertonic urine in maximally con- |
them (e.g., urea; !C). The countercurrent ex- |
|||
centrated urine). Since water diuresis permits |
change in the vasa recta permits the necessary |
||||
Kidneys, |
the excretion of large volumes of H2O without |
supply of blood to the renal medulla without |
|||
the simultaneous loss of NaCl and other sol- |
significantly altering the high osmolality of the |
||||
|
|||||
|
utes, this is known as “free water excretion”, or |
renal medulla and hence impairing the urine |
|||
7 |
“free water clearance” (CH2O). This allows the |
concentration capacity of the kidney. |
|||
kidney to normalize decreases in plasma |
In a countercurrent multiplier such as the |
||||
|
|||||
|
osmolality, for example (!p. 170). The CH2O |
loop of Henle, a concentration gradient be- |
|||
|
represents to the volume of water that could be |
tween the two limbs is maintained by the ex- |
|||
|
theoretically extracted in order for the urine to |
penditure of energy (!A5). The countercur- |
|||
|
|||||
|
reach the same osmolality as the plasma: |
rent |
flow amplifies the relatively small |
||
|
. |
[7.11] |
gradient at all points between the limbs (local |
||
|
CH2O " VU (1–[Uosm/Posm]). |
||||
|
Countercurrent Systems |
|
gradient of about 200 mOsm/kg H2O) to a rela- |
||
|
|
tively large gradient along the limb of the loop |
|||
|
A simple exchange system (!A1) can consist of |
(about 1000 mOsm/kg H2O). The longer the |
|||
|
two tubes in which parallel streams of water flow, one |
loop and the higher the one-step gradient, the |
|||
|
cold (0 #C) and one hot (100 #C). Due to the exchange |
steeper the multiplied gradient. In addition, it |
|||
|
of heat between them, the water leaving the ends of |
is inversely proportional to (the square of) the |
|||
|
both tubes will be about 50 #C, that is, the initially |
||||
|
flow rate in the loop. |
||||
|
steep temperature gradient of 100 #C will be offset. |
||||
|
|
|
|||
|
In countercurrent exchange of heat (!A2), the |
Reabsorption of Water |
|||
|
fluid within the tubes flows in opposite directions. |
||||
|
Approximately 65% of the GFR is reabsorbed at |
||||
|
Since a temperature gradient is present in all parts of |
||||
|
the tube, heat is exchanged along the entire length. |
the proximal convoluted tubule, PCT (!B and |
|||
|
Molecules can also be exchanged, provided the wall |
p. 157 D). The driving “force” for this is the re- |
|||
|
of the tube is permeable to them and that a concen- |
absorption of solutes, especially Na+ and Cl–. |
|||
|
tration gradient exists for the substance. |
|
This slightly dilutes the urine in the tubule, but |
||
|
If the countercurrent exchange of heat occurs in a |
||||
|
H2O immediately follows this small osmotic |
||||
|
hairpin-shaped loop, the bend of which is in contact |
||||
|
gradient because the PCT is “leaky” (!p. 154). |
||||
|
with an environment with a temperature different |
||||
|
from that inside the tube (ice, !A3), the fluid exit- |
The reabsorption of water can occur by a para- |
|||
|
ing the loop will be only slightly colder than that |
cellular route (through leaky tight junctions) |
|||
|
entering it, because heat always passes from the |
or transcellular route, i.e., through water chan- |
|||
|
warmer limb of the loop to the colder limb. |
|
nels (aquaporin type 1 = AQP1) in the two cell |
||
|
Countercurrent exchange of water in the vasa |
membranes. The urine in PCT therefore re- |
|||
164 |
recta of the renal medulla (!A6 and p. 150) oc- |
mains |
(virtually) isotonic. Oncotic pressure |
||
curs if the medulla becomes increasingly hy- |
(!p. 378) in the peritubular capillaries pro- |
||||
|
!
Despopoulos, Color Atlas of Physiology © 2003 Thieme
All rights reserved. Usage subject to terms and conditions of license.


