
книги студ / color atlas of physiology 5th ed[1]. (a. despopoulos et al, thieme 2003)
.pdf


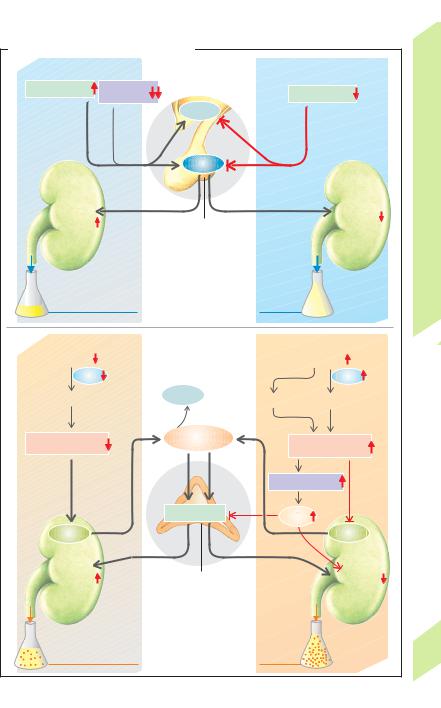

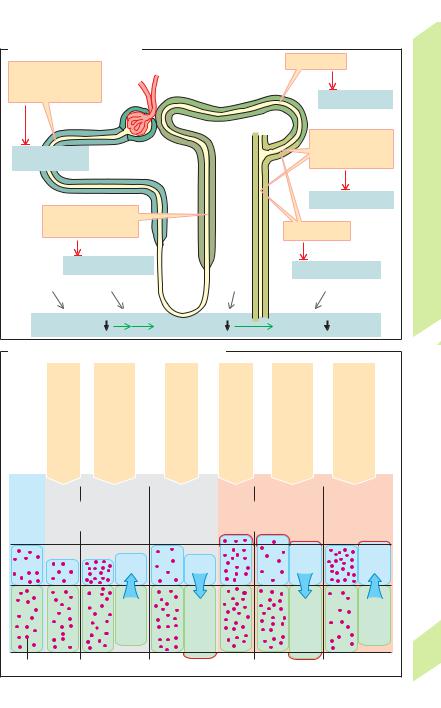

|
The Kidney and Acid–Base Balance |
CO2 and H2O (!B). CAIV anchored in the mem- |
|||||||
|
brane catalyzes this reaction. CO2 readily dif- |
||||||||
|
|
|
|
||||||
|
Main functions of renal H+ secretion (!A): |
fuses into the cell, perhaps via aquaporin 1 |
|||||||
|
— reabsorption of filtered bicarbonate (!B), |
(!p. 166). CAII then catalyzes the transforma- |
|||||||
|
— excretion of H+ ions measurable as titratable |
tion of CO2 + H2O to H+ + HCO3– within the cell |
|||||||
|
acidity (!C), and |
|
(!B). The H+ ions are again secreted, while |
||||||
|
— nonionic transport of NH4+, i.e. in the form |
HCO3– exits through the basolateral membrane |
|||||||
|
of NH3 (!D1, 2). |
|
of the cell via an electrogenic carrier (hNBC = |
||||||
Balance |
1. Very large quantities of H+ ions are secreted |
human Na+-bicarbonate co-transporter; !B). |
|||||||
into the lumen of the proximal tubule (!A1) |
The hNBC co-transports 1 Na+ with 3 HCO3– |
||||||||
|
by (a) primary active transport via H+-ATPase |
(and/or with 1 HCO3– + 1 CO32 –?) Thus, HCO3– |
|||||||
Water |
and (b) by secondary active transport via an |
is transported through the luminal membrane |
|||||||
electroneutral Na+/H+-antiporter (NHE3 car- |
in the form of CO2 (driving force: |
PCO2), and |
|||||||
|
rier, !p. 162). The luminal pH then decreases |
exits the cell across the basolateral membrane |
|||||||
and |
from 7.4 (filtrate) to about 6.6. One OH– ion re- |
as HCO3– (main driving force: membrane |
|||||||
mains in the cell for each H+ ion secreted; OH– |
potential). |
|
|
|
|
||||
Salt, |
reacts with CO2 to form HCO3– (accelerated by |
Hypokalemia leads to a rise in membrane potential |
|||||||
carbonic anhydrase-II, see below). |
HCO3– |
||||||||
|
|
|
|
||||||
Kidneys, |
leaves the cell for the blood, where it binds one |
(Nernst equation, !p. 32) and thus to a rise in baso- |
|||||||
lateral HCO3– transport. This results in increased H+ |
|||||||||
tion of one H+ |
ion from the body, except the |
Urinary acid excretion. If the dietary protein |
|||||||
|
H+ ion. Thus, each H+ ion secreted into the |
secretion and, ultimately, in hypokalemic alkalosis. |
|||||||
|
lumen (and excreted) results in the elimina- |
|
|
|
|
|
|
||
7 |
secreted H+ is accompanied by a secreted NH3 |
intake is 70 g per day (!p. 226), a daily load of |
|||||||
|
about 190 mmol of H+ occurs after the amino |
||||||||
|
(see below). |
|
|
||||||
|
|
|
acids of the protein have been metabolized. |
||||||
|
2. In the connecting tubule and collecting |
||||||||
|
HCl (from |
arginine, lysine and |
histidine), |
||||||
|
duct (!A2) type A intercalated cells secrete H+ |
||||||||
|
H2SO4 (from methionine and cystine), H3PO4, |
||||||||
|
ions via H+ /K+-ATPase and H+-ATPase, allowing |
||||||||
|
the luminal pH to drop as far as 4.5. In meta- |
and lactic acid are the main sources of H+ ions. |
|||||||
|
They are “fixed” acids which, unlike CO2, are |
||||||||
|
bolic alkalosis, |
type B intercalated cells can |
|||||||
|
not eliminated |
by respiration. Since about |
|||||||
|
secrete HCO3– (!A3). |
|
|||||||
|
|
130 mmol H+/day are used to break down or- |
|||||||
|
Carbonic anhydrase (CA) is important in all |
||||||||
|
ganic anions (glutamate–, aspartate–, lactate–, |
||||||||
|
cases where H+ ions exit from one side of a cell |
||||||||
|
etc.), the net H+ production is about 60 (40–80) |
||||||||
|
and/or HCO3– exits from the other, e.g., in renal |
||||||||
|
mmol/day. Although the H+ ions are buffered |
||||||||
|
tubule cells, which contain CAII in the cytosol |
||||||||
|
at their production site, they must be excreted |
||||||||
|
and CAIV on the outside of the luminal mem- |
||||||||
|
to regenerate the buffers. |
|
|
||||||
|
brane; !A, B, D), as well as in the stomach, |
|
|
||||||
|
In extreme cases, the urinary pH can rise to |
||||||||
|
small intestine, pancreatic duct and erythro- |
||||||||
|
about pH 8 (high HCO3– excretion) or fall to |
||||||||
|
cytes, etc. CA catalyzes the gross reaction |
||||||||
|
about |
pH |
4.5 |
(maximum |
H+ |
conc. is |
|||
|
H2O + CO2 |
H+ + HCO3–. |
|
||||||
|
|
0.03 mmol/L). At a daily urine output of 1.5 L, |
|||||||
|
|
|
|
||||||
|
Carbonic acid (H2CO3) is often considered to be the |
the kidneys will excrete !1% of the produced |
|||||||
|
intermediate product of this reaction, but OH– (not |
H+ ions in their free form. |
|
|
|||||
|
H2O) probably combines with CA. Therefore, the re- |
Titratable acids (80% phosphate, 20% uric |
|||||||
|
actions H2O OH – + H+ and OH– + CO2 |
HCO3– |
acid, citric |
acid, |
etc.) comprise |
a |
significant |
||
|
underlie the aforementioned gross reaction. |
|
|||||||
|
|
fraction |
(10–30 mmol/day) of |
H+ |
excretion |
||||
|
|
|
|
||||||
|
Reabsorption of HCO3– (!B). The amount of |
(!C1). This amount of H+ ions can be deter- |
|||||||
|
HCO3– filtered each day is 40 times the quan- |
mined by titrating the urine with NaOH back to |
|||||||
|
tity present in the blood. HCO3– must therefore |
the plasma pH value, which is normally pH 7.4 |
|||||||
|
be reabsorbed to maintain acid–base balance |
(!C2). Around 80% of phosphate (pKa = 6.8) in |
|||||||
|
(!p. 183ff.). The H+ ions secreted into the |
the blood occurs in the form of HPO42 –, |
|||||||
174 |
lumen of the proximal convoluted tubule react |
whereas about all phosphate in acidic urine |
|||||||
|
with about 90% of the filtered HCO3– to form |
occurs as H2PO4– (!p. 380), i.e., the secreted |
!
Despopoulos, Color Atlas of Physiology © 2003 Thieme
All rights reserved. Usage subject to terms and conditions of license.


