
- •CONTENTS
- •Preface
- •Contributors
- •1 Introduction to Toxicology
- •1.1 Definition and Scope, Relationship to Other Sciences, and History
- •1.1.2 Relationship to Other Sciences
- •1.1.3 A Brief History of Toxicology
- •1.3 Sources of Toxic Compounds
- •1.3.1 Exposure Classes
- •1.3.2 Use Classes
- •1.4 Movement of Toxicants in the Environment
- •Suggested Reading
- •2.1 Introduction
- •2.2 Cell Culture Techniques
- •2.2.1 Suspension Cell Culture
- •2.2.2 Monolayer Cell Culture
- •2.2.3 Indicators of Toxicity in Cultured Cells
- •2.3 Molecular Techniques
- •2.3.1 Molecular Cloning
- •2.3.2 cDNA and Genomic Libraries
- •2.3.3 Northern and Southern Blot Analyses
- •2.3.4 Polymerase Chain Reaction (PCR)
- •2.3.5 Evaluation of Gene Expression, Regulation, and Function
- •2.4 Immunochemical Techniques
- •Suggested Reading
- •3.1 Introduction
- •3.2 General Policies Related to Analytical Laboratories
- •3.2.1 Standard Operating Procedures (SOPs)
- •3.2.2 QA/QC Manuals
- •3.2.3 Procedural Manuals
- •3.2.4 Analytical Methods Files
- •3.2.5 Laboratory Information Management System (LIMS)
- •3.3 Analytical Measurement System
- •3.3.1 Analytical Instrument Calibration
- •3.3.2 Quantitation Approaches and Techniques
- •3.4 Quality Assurance (QA) Procedures
- •3.5 Quality Control (QC) Procedures
- •3.6 Summary
- •Suggested Reading
- •4 Exposure Classes, Toxicants in Air, Water, Soil, Domestic and Occupational Settings
- •4.1 Air Pollutants
- •4.1.1 History
- •4.1.2 Types of Air Pollutants
- •4.1.3 Sources of Air Pollutants
- •4.1.4 Examples of Air Pollutants
- •4.1.5 Environmental Effects
- •4.2 Water and Soil Pollutants
- •4.2.1 Sources of Water and Soil Pollutants
- •4.2.2 Examples of Pollutants
- •4.3 Occupational Toxicants
- •4.3.1 Regulation of Exposure Levels
- •4.3.2 Routes of Exposure
- •4.3.3 Examples of Industrial Toxicants
- •Suggested Reading
- •5 Classes of Toxicants: Use Classes
- •5.1 Introduction
- •5.2 Metals
- •5.2.1 History
- •5.2.2 Common Toxic Mechanisms and Sites of Action
- •5.2.3 Lead
- •5.2.4 Mercury
- •5.2.5 Cadmium
- •5.2.6 Chromium
- •5.2.7 Arsenic
- •5.2.8 Treatment of Metal Poisoning
- •5.3 Agricultural Chemicals (Pesticides)
- •5.3.1 Introduction
- •5.3.3 Organochlorine Insecticides
- •5.3.4 Organophosphorus Insecticides
- •5.3.5 Carbamate Insecticides
- •5.3.6 Botanical Insecticides
- •5.3.7 Pyrethroid Insecticides
- •5.3.8 New Insecticide Classes
- •5.3.9 Herbicides
- •5.3.10 Fungicides
- •5.3.11 Rodenticides
- •5.3.12 Fumigants
- •5.3.13 Conclusions
- •5.4 Food Additives and Contaminants
- •5.5 Toxins
- •5.5.1 History
- •5.5.2 Microbial Toxins
- •5.5.3 Mycotoxins
- •5.5.4 Algal Toxins
- •5.5.5 Plant Toxins
- •5.5.6 Animal Toxins
- •5.6 Solvents
- •5.7 Therapeutic Drugs
- •5.8 Drugs of Abuse
- •5.9 Combustion Products
- •5.10 Cosmetics
- •Suggested Reading
- •6 Absorption and Distribution of Toxicants
- •6.1 Introduction
- •6.2 Cell Membranes
- •6.3 Mechanisms of Transport
- •6.3.1 Passive Diffusion
- •6.4 Physicochemical Properties Relevant to Diffusion
- •6.4.1 Ionization
- •6.5 Routes of Absorption
- •6.5.1 Extent of Absorption
- •6.5.2 Gastrointestinal Absorption
- •6.5.3 Dermal Absorption
- •6.5.4 Respiratory Penetration
- •6.6 Toxicant Distribution
- •6.6.1 Physicochemical Properties and Protein Binding
- •6.7 Toxicokinetics
- •Suggested Reading
- •7 Metabolism of Toxicants
- •7.1 Introduction
- •7.2 Phase I Reactions
- •7.2.4 Nonmicrosomal Oxidations
- •7.2.5 Cooxidation by Cyclooxygenases
- •7.2.6 Reduction Reactions
- •7.2.7 Hydrolysis
- •7.2.8 Epoxide Hydration
- •7.2.9 DDT Dehydrochlorinase
- •7.3 Phase II Reactions
- •7.3.1 Glucuronide Conjugation
- •7.3.2 Glucoside Conjugation
- •7.3.3 Sulfate Conjugation
- •7.3.4 Methyltransferases
- •7.3.7 Acylation
- •7.3.8 Phosphate Conjugation
- •Suggested Reading
- •8 Reactive Metabolites
- •8.1 Introduction
- •8.2 Activation Enzymes
- •8.3 Nature and Stability of Reactive Metabolites
- •8.4 Fate of Reactive Metabolites
- •8.4.1 Binding to Cellular Macromolecules
- •8.4.2 Lipid Peroxidation
- •8.4.3 Trapping and Removal: Role of Glutathione
- •8.5 Factors Affecting Toxicity of Reactive Metabolites
- •8.5.1 Levels of Activating Enzymes
- •8.5.2 Levels of Conjugating Enzymes
- •8.5.3 Levels of Cofactors or Conjugating Chemicals
- •8.6 Examples of Activating Reactions
- •8.6.1 Parathion
- •8.6.2 Vinyl Chloride
- •8.6.3 Methanol
- •8.6.5 Carbon Tetrachloride
- •8.6.8 Acetaminophen
- •8.6.9 Cycasin
- •8.7 Future Developments
- •Suggested Reading
- •9.1 Introduction
- •9.2 Nutritional Effects
- •9.2.1 Protein
- •9.2.2 Carbohydrates
- •9.2.3 Lipids
- •9.2.4 Micronutrients
- •9.2.5 Starvation and Dehydration
- •9.2.6 Nutritional Requirements in Xenobiotic Metabolism
- •9.3 Physiological Effects
- •9.3.1 Development
- •9.3.2 Gender Differences
- •9.3.3 Hormones
- •9.3.4 Pregnancy
- •9.3.5 Disease
- •9.3.6 Diurnal Rhythms
- •9.4 Comparative and Genetic Effects
- •9.4.1 Variations Among Taxonomic Groups
- •9.4.2 Selectivity
- •9.4.3 Genetic Differences
- •9.5 Chemical Effects
- •9.5.1 Inhibition
- •9.5.2 Induction
- •9.5.3 Biphasic Effects: Inhibition and Induction
- •9.6 Environmental Effects
- •9.7 General Summary and Conclusions
- •Suggested Reading
- •10 Elimination of Toxicants
- •10.1 Introduction
- •10.2 Transport
- •10.3 Renal Elimination
- •10.4 Hepatic Elimination
- •10.4.2 Active Transporters of the Bile Canaliculus
- •10.5 Respiratory Elimination
- •10.6 Conclusion
- •Suggested Reading
- •11 Acute Toxicity
- •11.1 Introduction
- •11.2 Acute Exposure and Effect
- •11.3 Dose-response Relationships
- •11.4 Nonconventional Dose-response Relationships
- •11.5 Mechanisms of Acute Toxicity
- •11.5.1 Narcosis
- •11.5.2 Acetylcholinesterase Inhibition
- •11.5.3 Ion Channel Modulators
- •11.5.4 Inhibitors of Cellular Respiration
- •Suggested Reading
- •12 Chemical Carcinogenesis
- •12.1 General Aspects of Cancer
- •12.2 Human Cancer
- •12.2.1 Causes, Incidence, and Mortality Rates of Human Cancer
- •12.2.2 Known Human Carcinogens
- •12.3 Classes of Agents Associated with Carcinogenesis
- •12.3.2 Epigenetic Agents
- •12.4 General Aspects of Chemical Carcinogenesis
- •12.5 Initiation-Promotion Model for Chemical Carcinogenesis
- •12.6 Metabolic Activation of Chemical Carcinogens and DNA Adduct Formation
- •12.7 Oncogenes
- •12.8 Tumor Suppressor Genes
- •12.8.1 Inactivation of Tumor Suppressor Genes
- •12.8.2 p53 Tumor Suppressor Gene
- •12.9 General Aspects of Mutagenicity
- •12.10 Usefulness and Limitations of Mutagenicity Assays for the Identification of Carcinogens
- •Suggested Reading
- •13 Teratogenesis
- •13.1 Introduction
- •13.2 Principles of Teratology
- •13.3 Mammalian Embryology Overview
- •13.4 Critical Periods
- •13.5 Historical Teratogens
- •13.5.1 Thalidomide
- •13.5.2 Accutane (Isotetrinoin)
- •13.5.3 Diethylstilbestrol (DES)
- •13.5.4 Alcohol
- •13.6 Testing Protocols
- •13.6.1 FDA Guidelines for Reproduction Studies for Safety Evaluation of Drugs for Human Use
- •13.6.3 Alternative Test Methods
- •13.7 Conclusions
- •Suggested Reading
- •14 Hepatotoxicity
- •14.1 Introduction
- •14.1.1 Liver Structure
- •14.1.2 Liver Function
- •14.2 Susceptibility of the Liver
- •14.3 Types of Liver Injury
- •14.3.1 Fatty Liver
- •14.3.2 Necrosis
- •14.3.3 Apoptosis
- •14.3.4 Cholestasis
- •14.3.5 Cirrhosis
- •14.3.6 Hepatitis
- •14.3.7 Oxidative Stress
- •14.3.8 Carcinogenesis
- •14.4 Mechanisms of Hepatotoxicity
- •14.5 Examples of Hepatotoxicants
- •14.5.1 Carbon Tetrachloride
- •14.5.2 Ethanol
- •14.5.3 Bromobenzene
- •14.5.4 Acetaminophen
- •14.6 Metabolic Activation of Hepatotoxicants
- •Suggested Reading
- •15 Nephrotoxicity
- •15.1 Introduction
- •15.1.1 Structure of the Renal System
- •15.1.2 Function of the Renal System
- •15.2 Susceptibility of the Renal System
- •15.3 Examples of Nephrotoxicants
- •15.3.1 Metals
- •15.3.2 Aminoglycosides
- •15.3.3 Amphotericin B
- •15.3.4 Chloroform
- •15.3.5 Hexachlorobutadiene
- •Suggested Reading
- •16 Toxicology of the Nervous System
- •16.1 Introduction
- •16.2 The Nervous system
- •16.2.1 The Neuron
- •16.2.2 Neurotransmitters and their Receptors
- •16.2.3 Glial Cells
- •16.3 Toxicant Effects on the Nervous System
- •16.3.1 Structural Effects of Toxicants on Neurons
- •16.3.2 Effects of Toxicants on Other Cells
- •16.4 Neurotoxicity Testing
- •16.4.1 In vivo Tests of Human Exposure
- •16.4.2 In vivo Tests of Animal Exposure
- •16.4.3 In vitro Neurochemical and Histopathological End Points
- •16.5 Summary
- •Suggested Reading
- •17 Endocrine System
- •17.1 Introduction
- •17.2 Endocrine System
- •17.2.1 Nuclear Receptors
- •17.3 Endocrine Disruption
- •17.3.1 Hormone Receptor Agonists
- •17.3.2 Hormone Receptor Antagonists
- •17.3.3 Organizational versus Activational Effects of Endocrine Toxicants
- •17.3.4 Inhibitors of Hormone Synthesis
- •17.3.5 Inducers of Hormone Clearance
- •17.3.6 Hormone Displacement from Binding Proteins
- •17.4 Incidents of Endocrine Toxicity
- •17.4.1 Organizational Toxicity
- •17.4.2 Activational Toxicity
- •17.4.3 Hypothyroidism
- •17.5 Conclusion
- •Suggested Reading
- •18 Respiratory Toxicity
- •18.1 Introduction
- •18.1.1 Anatomy
- •18.1.2 Cell Types
- •18.1.3 Function
- •18.2 Susceptibility of the Respiratory System
- •18.2.1 Nasal
- •18.2.2 Lung
- •18.3 Types of Toxic Response
- •18.3.1 Irritation
- •18.3.2 Cell Necrosis
- •18.3.3 Fibrosis
- •18.3.4 Emphysema
- •18.3.5 Allergic Responses
- •18.3.6 Cancer
- •18.3.7 Mediators of Toxic Responses
- •18.4 Examples of Lung Toxicants Requiring Activation
- •18.4.1 Introduction
- •18.4.2 Monocrotaline
- •18.4.3 Ipomeanol
- •18.4.4 Paraquat
- •18.5 Defense Mechanisms
- •Suggested Reading
- •19 Immunotoxicity
- •19.1 Introduction
- •19.2 The Immune System
- •19.3 Immune Suppression
- •19.4 Classification of Immune-Mediated Injury (Hypersensitivity)
- •19.5 Effects of Chemicals on Allergic Disease
- •19.5.1 Allergic Contact Dermatitis
- •19.5.2 Respiratory Allergens
- •19.5.3 Adjuvants
- •19.6 Emerging Issues: Food Allergies, Autoimmunity, and the Developing Immune System
- •Suggested Reading
- •20 Reproductive System
- •20.1 Introduction
- •20.2 Male Reproductive Physiology
- •20.3 Mechanisms and Targets of Male Reproductive Toxicants
- •20.3.1 General Mechanisms
- •20.3.2 Effects on Germ Cells
- •20.3.3 Effects on Spermatogenesis and Sperm Quality
- •20.3.4 Effects on Sexual Behavior
- •20.3.5 Effects on Endocrine Function
- •20.4 Female Reproductive Physiology
- •20.5 Mechanisms and Targets of Female Reproductive Toxicants
- •20.5.1 Tranquilizers, Narcotics, and Social Drugs
- •20.5.2 Endocrine Disruptors (EDs)
- •20.5.3 Effects on Germ Cells
- •20.5.4 Effects on the Ovaries and Uterus
- •20.5.5 Effects on Sexual Behavior
- •Suggested Reading
- •21 Toxicity Testing
- •21.1 Introduction
- •21.2 Experimental Administration of Toxicants
- •21.2.1 Introduction
- •21.2.2 Routes of Administration
- •21.3 Chemical and Physical Properties
- •21.4 Exposure and Environmental Fate
- •21.5 In vivo Tests
- •21.5.1 Acute and Subchronic Toxicity Tests
- •21.5.2 Chronic Tests
- •21.5.3 Reproductive Toxicity and Teratogenicity
- •21.5.4 Special Tests
- •21.6 In vitro and Other Short-Term Tests
- •21.6.1 Introduction
- •21.6.2 Prokaryote Mutagenicity
- •21.6.3 Eukaryote Mutagenicity
- •21.6.4 DNA Damage and Repair
- •21.6.5 Chromosome Aberrations
- •21.6.6 Mammalian Cell Transformation
- •21.6.7 General Considerations and Testing Sequences
- •21.7 Ecological Effects
- •21.7.1 Laboratory Tests
- •21.7.2 Simulated Field Tests
- •21.7.3 Field Tests
- •21.8 Risk Analysis
- •21.9 The Future of Toxicity Testing
- •Suggested Reading
- •22 Forensic and Clinical Toxicology
- •22.1 Introduction
- •22.2 Foundations of Forensic Toxicology
- •22.3 Courtroom Testimony
- •22.4.1 Documentation Practices
- •22.4.2 Considerations for Forensic Toxicological Analysis
- •22.4.3 Drug Concentrations and Distribution
- •22.5 Laboratory Analyses
- •22.5.1 Colorimetric Screening Tests
- •22.5.2 Thermal Desorption
- •22.5.6 Enzymatic Immunoassay
- •22.6 Analytical Schemes for Toxicant Detection
- •22.7 Clinical Toxicology
- •22.7.1 History Taking
- •22.7.2 Basic Operating Rules in the Treatment of Toxicosis
- •22.7.3 Approaches to Selected Toxicoses
- •Suggested Reading
- •23 Prevention of Toxicity
- •23.1 Introduction
- •23.2 Legislation and Regulation
- •23.2.1 Federal Government
- •23.2.2 State Governments
- •23.2.3 Legislation and Regulation in Other Countries
- •23.3 Prevention in Different Environments
- •23.3.1 Home
- •23.3.2 Workplace
- •23.3.3 Pollution of Air, Water, and Land
- •23.4 Education
- •Suggested Reading
- •24 Human Health Risk Assessment
- •24.1 Introduction
- •24.2 Risk Assessment Methods
- •24.2.2 Exposure Assessment
- •24.2.3 Dose Response and Risk Characterization
- •24.3 Noncancer Risk Assessment
- •24.3.1 Default Uncertainty and Modifying Factors
- •24.3.2 Derivation of Developmental Toxicant RfD
- •24.3.3 Determination of RfD and RfC of Naphthalene with the NOAEL Approach
- •24.3.4 Benchmark Dose Approach
- •24.3.5 Determination of BMD and BMDL for ETU
- •24.3.6 Quantifying Risk for Noncarcinogenic Effects: Hazard Quotient
- •24.3.7 Chemical Mixtures
- •24.4 Cancer Risk Assessment
- •24.5 PBPK Modeling
- •Suggested Reading
- •25 Analytical Methods in Toxicology
- •25.1 Introduction
- •25.2 Chemical and Physical Methods
- •25.2.1 Sampling
- •25.2.2 Experimental Studies
- •25.2.3 Forensic Studies
- •25.2.4 Sample Preparation
- •25.2.6 Spectroscopy
- •25.2.7 Other Analytical Methods
- •Suggested Reading
- •26 Basics of Environmental Toxicology
- •26.1 Introduction
- •26.2 Environmental Persistence
- •26.2.1 Abiotic Degradation
- •26.2.2 Biotic Degradation
- •26.2.3 Nondegradative Elimination Processes
- •26.3 Bioaccumulation
- •26.4 Toxicity
- •26.4.1 Acute Toxicity
- •26.4.2 Mechanisms of Acute Toxicity
- •26.4.3 Chronic Toxicity
- •26.4.5 Abiotic and Biotic Interactions
- •26.5 Conclusion
- •Suggested Reading
- •27.1 Introduction
- •27.2 Sources of Toxicants to the Environment
- •27.3 Transport Processes
- •27.3.1 Advection
- •27.3.2 Diffusion
- •27.4 Equilibrium Partitioning
- •27.5 Transformation Processes
- •27.5.1 Reversible Reactions
- •27.5.2 Irreversible Reactions
- •27.6 Environmental Fate Models
- •Suggested Reading
- •28 Environmental Risk Assessment
- •28.1 Introduction
- •28.2 Formulating the Problem
- •28.2.1 Selecting Assessment End Points
- •28.2.2 Developing Conceptual Models
- •28.2.3 Selecting Measures
- •28.3 Analyzing Exposure and Effects Information
- •28.3.1 Characterizing Exposure
- •28.3.2 Characterizing Ecological Effects
- •28.4 Characterizing Risk
- •28.4.1 Estimating Risk
- •28.4.2 Describing Risk
- •28.5 Managing Risk
- •Suggested Reading
- •29 Future Considerations for Environmental and Human Health
- •29.1 Introduction
- •29.2 Risk Management
- •29.3 Risk Assessment
- •29.4 Hazard and Exposure Assessment
- •29.5 In vivo Toxicity
- •29.6 In vitro Toxicity
- •29.7 Biochemical and Molecular Toxicology
- •29.8 Development of Selective Toxicants
- •Glossary
- •Index
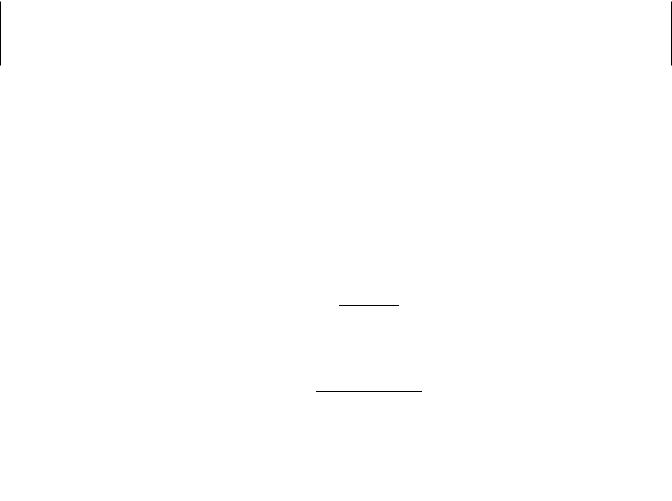
88 ABSORPTION AND DISTRIBUTION OF TOXICANTS
where P is the partition coefficient and usually expressed in terms of its logarithmic value (log P ), Vw and Vo are the volumes of aqueous and oil or organic phase, respectively, and Cwo and Cw are drug or toxic concentrations in the aqueous phase before and after shaking, respectively.
The lower the partition coefficient, the more water soluble, and the least permeable the toxicant is across a membrane. Regarding dermal absorption, partition coefficients can be predictive of absorption. However, toxicants with extremely high partition coefficients tend to remain in the membrane or skin. This explains why a strong correlation between permeability and the partition coefficient can exist for a hypothetical series of analogous chemicals for a specific range of partition coefficients, but the correlation does not exists for log P values greater than 6 in many instances. A log P of around 1 is often taken as desirable for skin penetration. The reader should also recall that this parameter is operative as the chemical diffuses across membranes (Figure 6.1) of varying lipid content during absorption, distribution, and elimination processes.
6.5ROUTES OF ABSORPTION
Primary routes of entry of toxicants to the human body are dermal, gastrointestinal, and respiratory. Methods for studying these different routes are numerous, but they are perhaps best developed for the study of dermal absorption because this route is subject to more direct methodology, whereas methods for studying respiratory or gastrointestinal absorption require more highly specialized instrumentation. Additional routes encountered in experimental studies include intraperitoneal, intramuscular, and subcutaneous routes. When direct entry into the circulatory system is desired, intravenous (IV) or intra-arterial injections can be used to bypass the absorption phase. Information from this more direct route of entry (e.g., IV) should, however, be used in addition to data from the extravascular route of interest to adequately assess the true extent of absorption of a toxicant.
6.5.1Extent of Absorption
It is often useful to determine how much of the drug actually penetrates the membrane barrier (e.g., skin or gastrointestinal tract) and gets into the blood stream. This is usually determined experimentally for oral and dermal routes of administration. The area under the curve (AUC) of the concentration-time profiles for oral or dermal routes is compared with the AUC for IV routes of administration. The AUC is determined by breaking the curve up into a series of trapezoids and summing all of the areas with the aid of an appropriate computer program (Figure 6.5).
The intravenous correction is very important if absolute bioavailability is desired. The ratio of these AUC values is absolute bioavailability, F :
F = (AUC)route .
(AUC)IV
The relationship above holds if the same doses are used with both routes, but the bioavailability should be corrected if different doses are used:
F= AUCroute × DoseIV . AUCIV × Doseroute
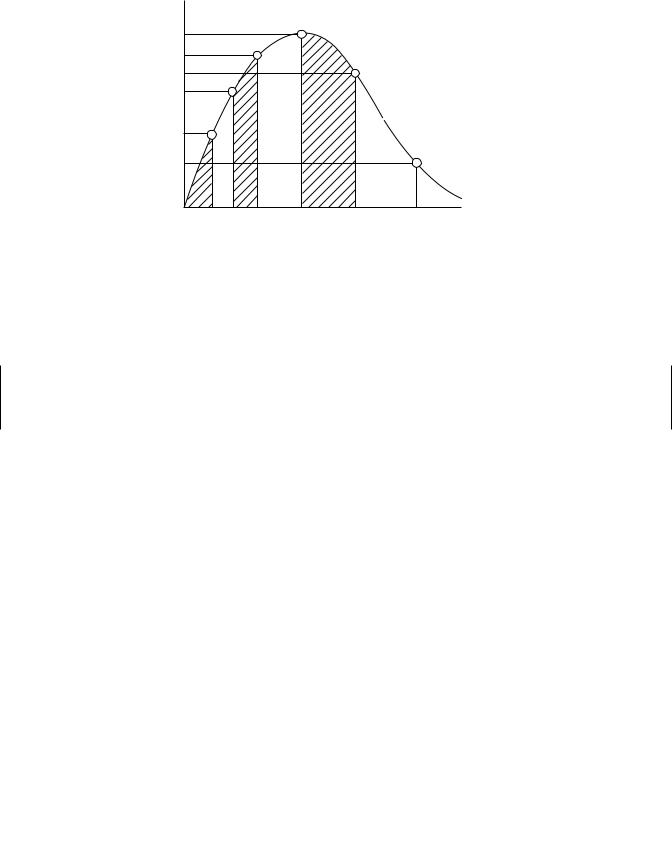
ROUTES OF ABSORPTION |
89 |
|
C4 |
|
|
|
|
|
|
C3 |
|
|
|
|
|
Concentration |
C5 |
|
|
|
|
|
C2 |
|
|
|
|
|
|
C1 |
|
|
|
|
|
|
|
|
|
|
|
|
|
|
|
|
|
|
|
Slope |
|
C6 |
|
|
|
|
|
|
T1 |
T2 |
T3 |
T4 |
T5 |
T6 |
|
|
|
|
|
Time |
|
Figure 6.5 Plasma concentration time profile for oral exposure to a toxicant and depiction of AUCs determined by summation of trapezoids at several time periods.
Another technique is to monitor drug or toxicant excretion rather than blood concentrations, especially when blood or plasma concentrations are very low. Using the same equations, the AUC is now replaced by chemical concentrations in urine, feces, and expired air. Some chemicals are primarily excreted by the kidney and urine data alone may be necessary. The rate and extent of absorption are clearly important for therapeutic and toxicological considerations. For example, different formulations of the same pesticide can change the absorption rate in skin or gastrointestinal tract, and not bioavailability, but can result in blood concentrations near the toxic dose. Also different formulations can result in similar absorption rates but different bioavailability.
6.5.2Gastrointestinal Absorption
The gastrointestinal tract (GIT) is a hollow tube (Figure 6.6a) lined by a layer of columnar cells, and usually protected by mucous, which offers minimal resistance to toxicant penetration. The distance from the outer membrane to the vasculature is about 40 µm, from which point further transport can easily occur. However, the cornified epithelium of the esophagus prevents absorption from this region of the GIT. Most of the absorption will therefore occur in the intestine (pH = 6), and to some extent in the stomach (pH = 1–3). Buccal and rectal absorption can occur in special circumstances. Note that secretions from the lachrymal duct, salivary gland, and nasal passages can enter the GIT via the buccal cavity. Therefore, following IV administration, a toxicant can enter the GIT if the drug is in these secretions.
The intestine can compensate the 2.5 log units difference between it and the stomach by the increased surface area in the small intestines. The presence of microvilli (Figure 6.6b) in the intestine is an increase of 600-fold in surface area compared to a hollow tube of comparable length. Note that there is no absorption, except for water, in the large intestine.
Most of the absorption in the GIT is by passive diffusion, except for nutrients; glucose, amino acids, and drugs that look like these substances are taken up by
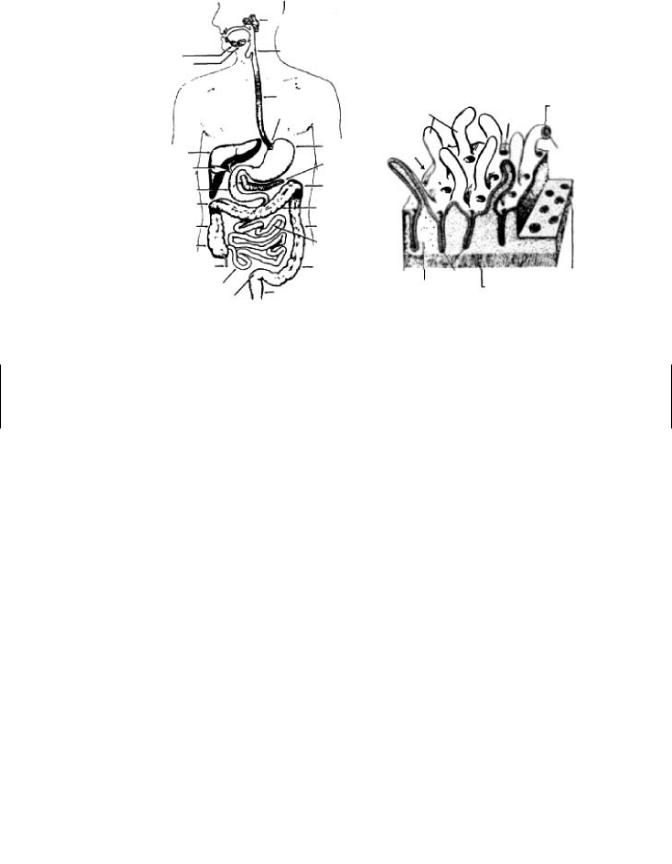
90 |
ABSORPTION AND DISTRIBUTION OF TOXICANTS |
|
|
|
|
|
|
Parotid Salivary Gland |
|
|
|
|
Salivary Glands |
Pharynx |
|
|
|
|
Sublingual |
|
|
|
|
|
Submaxillary |
|
|
|
|
|
|
Esophagus |
|
Opening of |
Villus |
|
|
|
Villi |
||
|
|
Cardia |
Crypt |
Cross |
|
|
|
|
|||
|
|
|
|
|
Sect. |
|
Liver |
Stomach |
Surface |
|
L.D |
|
|
|
|||
|
|
|
Core |
||
|
Gallbladder |
Pancreas |
|
|
|
|
|
|
|
||
|
|
|
|
|
|
|
Duodenum |
Left Colic |
|
|
|
|
Flexure |
|
|
|
|
Hepatic Flexure |
Transverse |
|
|
|
|
|
Ascending |
Colon |
|
|
|
|
Descending |
|
|
|
|
|
Colon |
Colon |
|
|
|
|
Cecum |
Jejunum |
|
|
|
|
Appendix |
Sigmoid |
Crypts |
Crypts Cross Sect. |
|
|
Ileum |
|
|||
|
Rectum |
Lamina |
Muscularis |
|
|
|
Sigmoid Flexure |
Propria |
Mucosa |
|
|
|
(a) |
|
|
(b) |
|
Figure 6.6 Schematic showing (a) alimentary canal and associated structures and (b) lining of the small intestine. (Sources:(a) Scholtelius and Scholtelius in Textbook of Physiology, Mosby, 1973; (b) Ham and Cormack, in Histology, 8th ed., Lippincott, 1979.)
active transport. For toxicants with structural similarities to compounds normally taken up by these active transport mechanisms, entry is enhanced. For example, cobalt is absorbed by the same active transport mechanism that normally transports iron, and 5-bromouracil is absorbed by the pyrimidine transport system.
Very lipid soluble toxicants and drugs, which are not miscible in the aqueous intestinal fluid, are presented as emulsions, and brought into solution through the action of detergent-like bile acids. The product of this mixing is large surface area micelles (hydrophobic interior) that deliver the lipids to the brush border of the intestine for diffusion across the membrane. As stated previously, the rate of passive transfer will be dependent on ionization and lipid solubility. Very strong bases (e.g., tubocurarine, succinylcholine) and strong acids are not readily absorbed in the GIT. These muscle relaxants therefore are given IV. The smaller the particle size of the toxicant, the greater is the absorption, and a chemical must be in aqueous solution for it to be absorbed in the GIT. A feature of the GIT that seems to contradict basic assumptions of absorption is the penetration of certain very large molecules. Compounds such as bacterial endotoxins, large particles of azo dyes, and carcinogens are apparently absorbed by endocytotic mechanisms.
GIT motility has a significant effect on GIT absorption of a toxicant. For example, excessively rapid movement of gut contents can reduce absorption by reducing residence time in the GIT, while the presence of food in the stomach can delay the progress of drugs from the stomach to the small intestine where most of the absorption will occur. Increased splanchnic blood flow after a meal can result in absorption of several drugs (e.g., propranolol), but in hypovolemic states, absorption can be reduced.
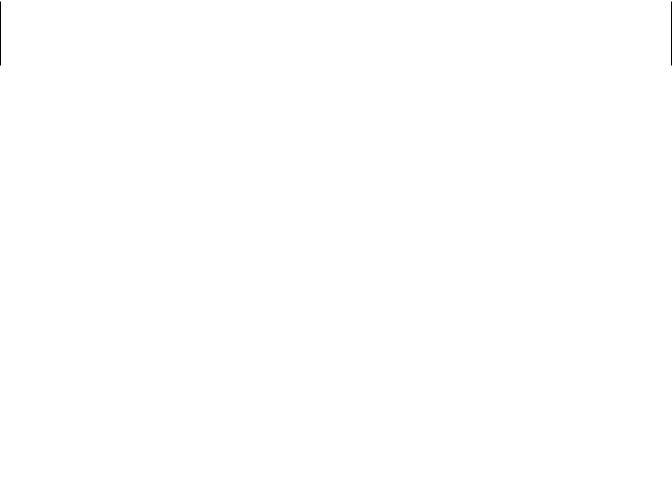
ROUTES OF ABSORPTION |
91 |
Biotransformation in the GIT prior to absorption can have a significant impact on bioavailability of a toxicant. The resident bacterial population can metabolize drugs in the GIT. Because of microbial fermentation in the rumen of ruminants and large intestine and cecum of horses and rabbits, its is often difficult to compare drug absorption profiles with carnivores (e.g., dogs) and omnivores (e.g., humans, pigs). Acid hydrolysis of some compounds can also occur, and enzymes in the intestinal mucosa can also have an effect on oral bioavailability. If the toxicant survive these microbial and chemical reactions in the stomach and small intestine, it is absorbed in the GIT and carried by the hepatic portal vein to the liver, which is the major site of metabolism. Chapters 7, 8, and 9 will discuss liver metabolism of toxicants in more detail. In brief, this activity in the liver can result in detoxification and/or bioactivation. Some drugs and toxicant that are conjugated (e.g., glucuronidation) in the liver are excreted via the biliary system back into the GIT. Once secreted in bile by active transport and excreted from the bile duct into the small intestine, this conjugated toxicant can be subjected to microbial beta-glucuronidase activity that can result in regeneration of the parent toxicant that is more lipophilic than the conjugate. The toxicant can now be reabsorbed by the GIT, prolonging the presence of the drug or toxicant in the systemic circulation. This is called enterohepatic circulation, which will be covered in greater detail in subsequent chapters.
6.5.3Dermal Absorption
The skin is a complex multilayered tissue with a large surface area exposed to the environment. Skin anatomy, physiology, and biochemistry vary among species, within species, and even between anatomic sites within an individual animal or human. Logically these biological factors alone can influence dermal absorption. What is consistent is that the outer layer, the stratum corneum (SC), can provide as much as 80% of the resistance to absorption to most ions as well as aqueous solutions. However, the skin is permeable to many toxicants, and dermal exposure to agricultural pesticides and industrial solvent can result in severe systemic toxicity.
The anatomy of the skin is depicted in the schematic diagram of Figure 6.7. In mammalian skin there are really three distinct layers, which are the epidermis, dermis, and hypodermis or subcutaneous fat layer. Human skin is 3 mm thick, but it is the epidermis, which is only 0.1 to 0.8 mm, that provides the greatest resistance to toxicant penetration. The five layers of the epidermis, starting from the outside, are the stratum corneum, stratum lucidum, stratum granulosum, stratum spinosum, and stratum basale. The basal cells of the epidermis proliferate and differentiate as they migrate outward toward the surface of the skin. It requires about 2 to 28 days for cells to migrate from the basal layer to the stratum corneum, where they are eventually sloughed off. These dead, keratinized cells are, however, very water absorbant (hydrophilic), a property that keeps the skin soft and supple. Sebum, a natural oil covering the skin, functions in maintaining the water-holding ability of the epidermis. The stratum corneum is the primary barrier to penetration, and it consists primarily of these dead keratin-filled keratinocytes embedded in an extracellular lipid matrix. The lipids are primarily sterols, other neutral lipids, and ceramides. This association between lipids and dead keratinized cells, which is often referred to as the “brick and mortar” model as depicted in Figure 6.7b, is used to simplify the composition of the stratum corneum that is integral to chemical transport through skin.
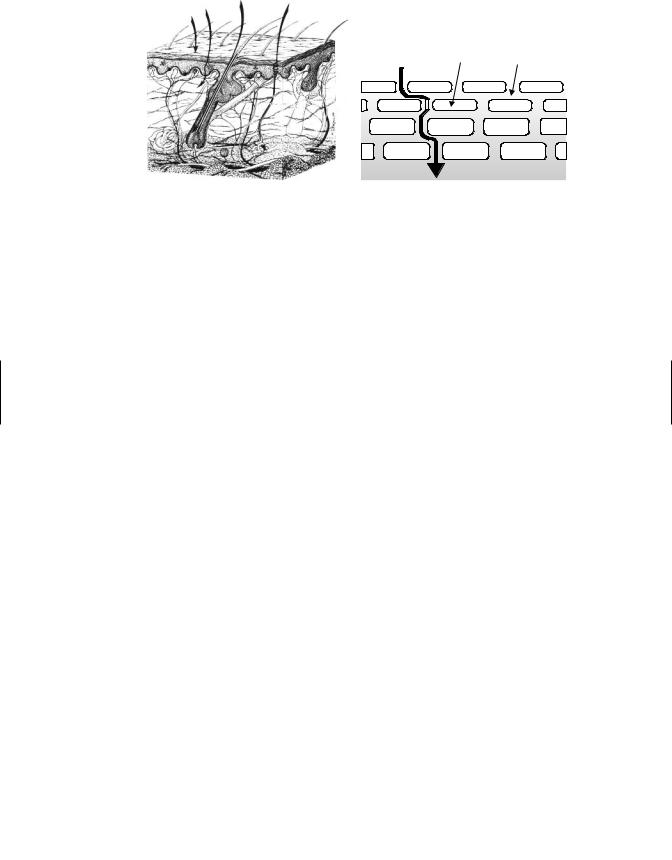
92 ABSORPTION AND DISTRIBUTION OF TOXICANTS
A B C D
Protein Rich |
Intercellular |
|
Corneocytes |
||
Lipids |
||
|
||
|
|
|
|
|
(a) |
(b) |
Figure 6.7 (a) Schematic diagram of the microstructure of mammalian skin and potential pathways for absorption by (A) intercellular, (B) transcellular, (C) transfollicular, or (D) sweat pore routes. (b) Brick-and-mortar” model of the stratum corneum depicting intercellular pathway (i.e., route A) between keratinocytes through the lipid domain of the stratum corneum.
A number of appendages are associated with the skin, including hair follicles, sebaceous glands, eccrine and apocrine sweat glands, and nails. Recently it was found that removal of the stratum corneum does not allow complete absorption; thus it is apparent that some role, although of lesser importance, is played by other parts of the skin. The dermis and subcutaneous areas of the skin are less important in influencing penetration, and once a toxicant has penetrated the epidermis, the other layers are traversed rather easily. The dermis is highly vascular, a characteristic that provides maximal opportunity for further transport once molecules have gained entry through the epidermis or through skin appendages. Most of the systemic absorption occurs at the capillary loops located at the epidermis-dermis junction. The blood supply of the dermis is under neural and humoral influences whose temperature-regulating functions could thus affect penetration and distribution of toxicants. Vasoactive drugs or environmental temperature can also influence absorption by altering blood flow to these capillaries. The subcutaneous layer of the skin is highly lipid in nature and serves as a shock absorber, an insulator, and a reserve depot of energy. The pH of the skin varies between 4 and 7 and is markedly affected by hydration.
Cutaneous biotransformation is mostly associated with the stratum basale layer where there can be phase I and phase II metabolism. However, the skin is not very efficient, compared to the liver. The epidermal layer accounts for the major portion of biochemical transformations in skin, although the total skin activity is low (2–6% that of the liver). Where activity is based on epidermis alone, that layer is as active as the liver or, in the case of certain toxicants, several times more active. For some chemicals, metabolism can influence absorption, and transdermal delivery systems of drugs utilize this activity. For example prodrug such as lipid esters are applied topically, and cutaneous esterases liberate the free drug. These basal cells and extracellular esterases have been shown to be involved in detoxification of several pesticides and bioactivation of carcinogens such as benzo(a)pyrene. For rapidly penetrating substances, metabolism by the skin is not presently considered to be of major significance, but skin may have an important first-pass metabolic function, especially for compounds that are absorbed slowly.
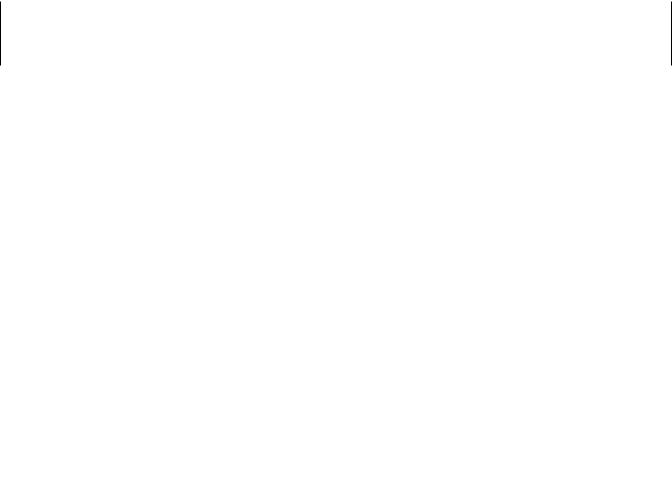
ROUTES OF ABSORPTION |
93 |
The intercellular pathway is now accepted as the major pathway for absorption. Recall that the rate of penetration is often correlated with the partition coefficient. In fact this is a very tortuous pathway, and the h (skin thickness) in Fick’s first law of diffusion is really 10× the measured distance. By placing a solvent (e.g., ether, acetone) on the surface or tape stripping the surface, the stratum corneum (SC) is removed, and absorption can be significantly increased by removing this outer barrier. This may not be the case for very lipophilic chemical. This is because the viable epidermis and dermis are regarded as aqueous layers compared to the SC. Note that the more lipophilic the drug, the more likely it will form a depot in the SC and be slowly absorbed over time and thus have a prolonged half-life.
The transcellular pathway has been discredited as a major pathway, although some polar substances can penetrate the outer surface of the protein filaments of hydrated stratum corneum. The transfollicular pathway is really an invagination of the epidermis into the dermis, and the chemical still has to penetrate the epidermis to be absorbed into the blood stream. This is also a regarded as minor route. Sweat pores are not lined with the stratum corneum layer, but the holes are small, and this route is still considered a minor route for chemical absorption. In general, the epidermal surface is 100 to 1000 times the surface area of skin appendages, and it is likely that only very small and/or polar molecules penetrate the skin via these appendages.
Variations in areas of the body cause appreciable differences in penetration of toxicants. The rate of penetration is in the following order:
Scrotal > Forehead > Axilla >= Scalp > Back = Abdomen > Palm and plantar.
The palmar and plantar regions are highly cornified and are 100 to 400 times thicker than other regions of the body. Note that there are differences in blood flow and to a lesser extent, hair density, that may influence absorption of more polar toxicants.
Formulation additives used in topical drug or pesticide formulations can alter the stratum corneum barrier. Surfactants are least likely to be absorbed, but they can alter the lipid pathway by fluidization and delipidization of lipids, and proteins within the keratinocytes can become denatured. This is mostly likely associated with formulations containing anionic surfactants than non-ionic surfactants. Similar effects can be observed with solvents. Solvents can partition into the intercellular lipids, thereby changing membrane lipophilicity and barrier properties in the following order: ether/acetone > DMSO > ethanol > water. Higher alcohols and oils do not damage the skin, but they can act as a depot for lipophilic drugs on the skin surface. The presence of water in several of these formulations can hydrate the skin. Skin occlusion with fabric or transdermal patches, creams, and ointments can increase epidermal hydration, which can increase permeability.
The reader should be aware of the animal model being used to estimate dermal absorption of toxicants in humans. For many toxicants, direct extrapolation from a rodent species to human is not feasible. This is because of differences in skin thickness, hair density, lipid composition, and blood flow. Human skin is the least permeable compared to skin from rats, mice, and rabbits. Pig skin is, however, more analogous to human skin anatomically and physiologically, and pig skin is usually predictive of dermal absorption of most drugs and pesticides in human skin. Human skin is the best model, followed by skin from pigs, primates, and hairless guinea pigs, and then rats, mice, and rabbits. In preliminary testing of a transdermal drug, if the drug does
- #15.08.20134.04 Mб17Hastie T., Tibshirani R., Friedman J. - The Elements of Statistical Learning Data Mining, Inference and Prediction (2002)(en).djvu
- #
- #
- #
- #
- #
- #
- #
- #15.08.201315.44 Mб26Hudlicky M, Pavlath A.E. (eds.) - Chemistry of Organic Fluorine Compounds 2[c] A critical Review (1995)(en).djvu
- #
- #